Analytical Chemistry Titration - PDF
Document Details
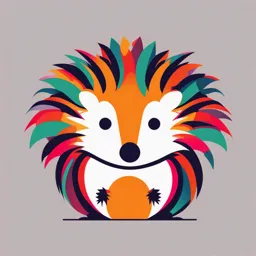
Uploaded by EminentMorganite
Tags
Summary
This document discusses the importance of neutralization titrations in analytical chemistry, focusing on the determination of acid and base concentrations. It includes examples, calculations, and the use of standard solutions. The text describes procedures for preparing and standardizing acid solutions, with a focus on sodium carbonate as a primary standard.
Full Transcript
2. A 100.0-mL sample of brackish water was made ammoniacal, and the sulfide it contained was titrated with 16.47 mL of 0.02310 M AgNO3. The analytical reaction is: 2 Ag+ + S2- à Ag2S Calculate the concentration of H2S (molar mass = 34 g/mol) in the water in parts per million, ppm. Step 1. Find the s...
2. A 100.0-mL sample of brackish water was made ammoniacal, and the sulfide it contained was titrated with 16.47 mL of 0.02310 M AgNO3. The analytical reaction is: 2 Ag+ + S2- à Ag2S Calculate the concentration of H2S (molar mass = 34 g/mol) in the water in parts per million, ppm. Step 1. Find the stoichiometric ratio. 1 mole of H2S reacts with 2 moles AgNO3, so the stoichiometric ratio is: 1 𝑚𝑜𝑙 𝐻# 𝑆 𝑠𝑡𝑜𝑖𝑐ℎ𝑖𝑜𝑚𝑒𝑡𝑟𝑖𝑐 𝑟𝑎𝑡𝑖𝑜 = 2 𝑚𝑜𝑙 AgN𝑂8 Step 2. Find the number of moles AgNO3 used: 𝑛𝑜. 𝑜𝑓 𝑚𝑜𝑙𝑒𝑠 𝐴𝑔𝑁𝑂8 = 16.47 𝒎𝑳 𝑨𝒈𝑵𝑶𝟑 × 1 𝑳 𝑨𝒈𝑵𝑶𝟑 𝑚𝑜𝑙 𝐴𝑔𝑁𝑂8 × 0.02310 1000 𝒎𝑳 𝑨𝒈𝑵𝑶𝟑 𝑳 𝑨𝒈𝑵𝑶𝟑 Step 3. Find the number of moles H2S in the sample: 1 𝑳 𝑨𝒈𝑵𝑶𝟑 𝒎𝒐𝒍 𝑨𝒈𝑵𝑶𝟑 1 𝑚𝑜𝑙 𝐻# 𝑆 𝑛𝑜. 𝑜𝑓 𝑚𝑜𝑙𝑒𝑠 𝐻# 𝑆 = 16.47 𝒎𝑳 𝑨𝒈𝑵𝑶𝟑 × × 0.02310 × 1000 𝒎𝑳 𝑨𝒈𝑵𝑶𝟑 𝑳 𝑨𝒈𝑵𝑶𝟑 2 𝒎𝒐𝒍 𝐀𝐠𝐍𝑶𝟑 Step 4. Find the mass of H2S (in mg): 3 𝑳 𝑨𝒈𝑵𝑶𝟑 𝑚𝑔 𝐻# 𝑆 = 16.47 𝒎𝑳 𝑨𝒈𝑵𝑶𝟑 × 3??? 𝒎𝑳 𝑨𝒈𝑵𝑶 × 0.02310 𝟑 𝒎𝒐𝒍 𝑨𝒈𝑵𝑶𝟑 𝑳 𝑨𝒈𝑵𝑶𝟑 3 𝒎𝒐𝒍 𝑯 𝑺 8$ 𝒈 𝑯 𝑺 𝟐 × # 𝒎𝒐𝒍 𝐀𝐠𝐍𝑶 × 3 𝒎𝒐𝒍 𝑯𝟐 𝑺 × 𝟑 𝟐 3??? H( 𝑯𝟐 𝑺 3 ( 𝑯𝟐 𝑺 Step 5. Find the ppm H2S: 𝑝𝑝𝑚 𝐻# 𝑆 1 𝑳 𝑨𝒈𝑵𝑶𝟑 𝒎𝒐𝒍 𝑨𝒈𝑵𝑶𝟑 1 𝒎𝒐𝒍 𝑯𝟐 𝑺 34 𝒈 𝑯𝟐 𝑺 1000 𝑚𝑔 𝑯𝟐 𝑺 16.47 𝒎𝑳 𝑨𝒈𝑵𝑶𝟑 × × 0.02310 × × × 1000 𝒎𝑳 𝑨𝒈𝑵𝑶𝟑 𝑳 𝑨𝒈𝑵𝑶𝟑 2 𝒎𝒐𝒍 𝐀𝐠𝐍𝑶𝟑 1 𝒎𝒐𝒍 𝑯𝟐 𝑺 1 𝑔 𝑯𝟐 𝑺 = 1𝐿 100 𝑚𝐿 × 1000 𝑚𝐿 = 64. 8 𝑝𝑝𝑚 IV. STANDARD SOLUTIONS IN NEUTRALIZATION TITRATION Importance of Neutralization Titrations Neutralization titrations are widely used to determine the amounts of acids and bases. In addition, neutralization titrations can be used to monitor the progress of reactions that produce or consume hydrogen ions. In clinical chemistry, for example, pancreatitis can be diagnosed by measuring the activity of serum lipase. Lipases hydrolyze the long-chain fatty acid triglyceride. The reaction liberates two moles of fatty acid and one mole of monoglyceride for each mole of triglyceride present according to: triglyceride à monoglyceride + 2 fatty acid The reaction is allowed to proceed for a certain amount of time, and then the liberated fatty acid is titrated with NaOH using a phenolphthalein indicator. The amount of fatty acid produced in a fixed time is related to the lipase activity. Moreover, acids and bases are very important in the environment. For instance, acid rain falling on the surface waters of lakes and rivers can cause these waters to become acidic. In the eastern United States, the number of acidic lakes increased dramatically over the period of 1930 to 1970, and this increase has been attributed to acid rain. On the other hand, many lakes in the Midwest have no problem with acidification even though the industrial Midwest is presumed to be a major source of the acids found in acid rain. In the Midwest, the surface rock is mostly limestone (calcium carbonate), which reacts with CO2 and H2O to form bicarbonate. Bicarbonate in turn neutralizes acids to maintain the pH relatively constant. This effect is characterized by the acid neutralizing Page 8 of 15 capacity of the lake, which is usually quite large in limestone-rich areas. In contrast, many eastern lakes and streams are surrounded by granite, which is a much less reactive rock. These bodies of water have little neutralizing capacity and so are more susceptible to acidification. To combat this problem, limestone is often imported from limestone-rich states into the eastern states and applied to lakes and streams. Acid neutralizing capacity is frequently determined by titration with a standard solution of acid. As seen in the situations above, neutralization titrations are widely used to determine the concentration of acidic or basic analytes or analytes that can be converted to acids or bases by suitable treatment. Like all titrations, neutralization titrations depend on a chemical reaction of the analyte with a standard reagent where a method of determining the point of chemical equivalence is available. Typically, a chemical is used to locate the end point. The discussion below focuses on the types of standard solutions and the chemical indicators that are used for neutralization titrations. A. Preparation and Standardization of Standard Acid Solutions Preparation of Standard Acid Solutions To obtain most standard acid solutions, a solution of an approximate concentration is first prepared by diluting the concentrated reagent. The diluted acid solution is then standardized against a primary-standard base. The standard acid solutions used in neutralization titrations are strong acids because these substances react more completely with an analyte than do weak acids, and as a result, they produce sharper end points. Standard solutions of acids are prepared by diluting concentrated hydrochloric acid (HCl), perchloric acid (HClO4), or sulfuric acid (H2SO4). Nitric acid (HNO3) is seldom used because its oxidizing properties offer the potential for undesirable side reactions. Among the acids mentioned above, hydrochloric acid solutions are widely used as standard solutions for titrating bases. Dilute solutions of HCl are stable indefinitely, and many chloride salts are soluble in aqueous solution. Solutions of perchloric acid and sulfuric acid are also stable and are useful for titrations where chloride ion interferes by forming precipitates. Standardization of Acids Sodium carbonate (Na2CO3) o Na2CO3 is the most frequently used reagent for standardizing acids. Primary-standard-grade sodium carbonate is available commercially or can be prepared by heating purified sodium hydrogen carbonate between 270 to 300°C for 1 hr. o o o Page 9 of 15 2 NaHCO3 (s) à Na2CO3 + H2O (g) + CO2 (g) An accurately determined mass of the primary-standard material is then taken to standardize the acid. In the titration of Na2CO3, there are two end points. The first corresponds to the conversion of carbonate to hydrogen carbonate and the second involves the formation of carbonic acid and carbon dioxide. That is why, typically, an even sharper end point can be achieved by boiling the solution briefly to eliminate the reaction product, carbonic acid and carbon dioxide. The sample is titrated to the first appearance of the acid color of the indicator (such as methyl orange). At this point, the solution contains a large amount of dissolved carbon dioxide and small amounts of carbonic acid and unreacted hydrogen carbonate. Boiling effectively destroys this buffer by eliminating the carbonic acid: H2CO3 (aq) à CO2 (g) + H2O (l) The solution then becomes alkaline again due to the residual hydrogen carbonate ion. The titration is completed after the solution has cooled. Alternatively, an amount of acid slightly in excess of that needed to convert the sodium carbonate to carbonic acid can be introduced. The solution is boiled as before to remove carbon dioxide and cooled. The excess acid is then back-titrated with a dilute solution of standard base. Any indicator suitable for a strong acid/strong base titration is satisfactory. An independent titration is used to establish the volume ratio of acid to base. Other Primary Standards o Tris-(hydroxymethyl)aminomethane, (HOCH2)3CNH2, known also as TRIS or THAM, is available in primary-standard purity from commercial sources. The main advantage of TRIS is its much greater mass per mole of H+ consumed (121.1 g/mol) than sodium carbonate (53.0 g/mol). The reaction of TRIS with acids is o (HOCH2)3CNH2 + H3O+ à (HOCH2)3CNH3+ + H2O Sodium tetraborate decahydrate and mercury(II) oxide have also been recommended as primary standards. B. Preparation and Standardization of Standard Base Solutions Preparation of Standard Base Solutions The standard base solutions used in neutralization titrations are also prepared from strong bases for the same reason as why strong acids are used to prepare standard acid solutions. Sodium hydroxide (NaOH) is the most common base for preparing standard solutions, although potassium hydroxide (KOH) and barium hydroxide (Ba(OH)2) are also used. These bases cannot be obtained in primary-standard purity, and so, all must be standardized after they are prepared. In solution as well as in the solid state, the hydroxides of sodium, potassium, and barium react rapidly with atmospheric carbon dioxide to produce the corresponding carbonate: CO2 (g) + 2 OH- à CO32- + H2O The solid reagents used to prepare standard solutions of base are always contaminated by significant amounts of carbonate ion. This decrease the sharpness of end points. For this reason, carbonate ion is usually removed before a solution of a base is standardized. The best method for preparing carbonate-free sodium hydroxide solutions takes advantage of the very low solubility of sodium carbonate in concentrated solutions of the base. An approximately 50% aqueous solution of sodium hydroxide is prepared or purchased from commercial sources. The solid sodium carbonate is allowed to settle to give a clear liquid that is decanted and diluted to give the desired concentration. Alternatively, the solid can be removed by vacuum filtration. Water that is used to prepare carbonate-free solutions of base must also be free of carbon dioxide. Distilled water, which is sometimes supersaturated with carbon dioxide, should be boiled briefly to eliminate CO2. The water is then allowed to cool to room temperature before base is introduced because hot alkali solutions rapidly absorb carbon dioxide. Deionized water usually does not contain significant amounts of carbon dioxide. Standardization of Bases Several excellent primary standards are available for standardizing bases. Most are weak organic acids that require the use of an indicator with a basic transition range. Potassium hydrogen phthalate (KHC8H4O4) o Potassium hydrogen phthalate, also known as KHP, is a nearly ideal primary standard. It is a nonhygroscopic crystalline solid with a relatively large molar mass (204 g/mol). For most purposes, the commercial analytical-grade salt can be used without further purification. Other Primary Standards for Bases o Benzoic acid can be obtained in primary-standard purity and used for the standardization of bases. Benzoic acid has limited solubility in water, so it is usually dissolved in ethanol prior Page 10 of 15 o to dilution with water and titration. A blank should always be carried through this standardization because commercial alcohol is sometimes slightly acidic. Potassium hydrogen iodate (KH(IO3)2) is an excellent primary standard with a high molar mass per mole of protons. It is also a strong acid that can be titrated using virtually any indicator with a transition range between pH 4 and 10. V. ACID/BASE INDICATORS Many naturally occurring and synthetic compounds exhibit colors that depend on the pH of the solutions in which they are dissolved. Some of these substances, which have been used for centuries to indicate the acidity or alkalinity of water, are still applied today as acid/base indicators. An acid/base indicator is a weak organic acid or a weak organic base whose undissociated form differs in color from its conjugate base or its conjugate acid form. For example, the behavior of an acid-base indicator, HIn, is described by the equilibrium: colorless HIn + H2O ↔ In- + H3O+ acid color base color In this reaction, internal structural changes accompany dissociation and cause the color change. As an example, the structural changes of the indicator phenolphthalein in response to changes in pH is shown at the side. Titration Errors with Acid/Base Indicators There are two types of titration error due to acid/base inidicators: o The first is a determinate error that occurs when the pH at which the indicator changes color differs from the pH at the equivalence point. This type of error can usually be minimized by choosing the indicator carefully or by making a blank correction. o The second type is an indeterminate error that originates from the limited ability of the human eye to distinguish reproducibly the intermediate color of the indicator. The magnitude of this error depends on the change in pH per milliliter of reagent at the equivalence point, on the concentration of the indicator, and on the sensitivity of the eye to the two indicator colors. On average, the visual uncertainty with an acid/base indicator is in the range of 60.5 to 61 pH unit. This uncertainty can often be decreased to as little as 60.1 pH unit by matching the color of the solution being titrated with that of a reference standard containing a similar amount of indicator at the appropriate pH. These uncertainties are approximations that vary considerably from indicator to indicator as well as from person to person. Common Acid/Base Indicators Common Transition Color Change Name Range 1.2 – 2.8 Red – Yellow Thymol blue 8.0 – 9.6 Yellow – Blue Methyl yellow 2.9 – 4.0 Red - Yellow Methyl orange 3.1 – 4.4 Red – Orange Methyl red 4.2 – 6.3 Red - Yellow Page 11 of 15 Common Name Phenol red Cresol purple Phenolphthalein Bromocresol green Transition Range 6.8 – 8.4 7.6 – 9.2 8.3 – 10.0 Yellow - Red Yellow - Purple Colorless - Red 3.8 – 5.4 Yellow - Blue Color Change VI. SOME APPLICATIONS OF NEUTRALIZATION TITRATIONS Neutralization titrations are used to determine the many inorganic, organic, and biological species that possess acidic or basic properties. In addition, however, there are nearly as many applications in which the analyte is converted to an acid or base by suitable chemical treatment and then titrated with a standard strong base or acid. Below are some applications for neutralization titration. A. Elemental Analysis Nitrogen Nitrogen occurs in a wide variety of substances of interest in the life sciences, in industry, and in agriculture. Examples include amino acids, proteins, synthetic drugs, fertilizers, explosives, soils, potable water supplies, and dyes. The most common method for determining organic nitrogen is the Kjeldahl method, which is based on a neutralization titration. Advantages of the Kjeldahl method: o The procedure is straightforward. o It requires no special equipment. o It is readily adapted to the routine analysis of large numbers of samples. The Kjeldahl method, or one of its modifications, is the standard process for determining the protein content of grains, meats, and biological materials. Since most proteins contain approximately the same percentage of nitrogen, multiplication of this percentage by a suitable factor (6.25 for meats, 6.38 for dairy products, and 5.70 for cereals) gives the percentage of protein in a sample. Mechanism of the Kjeldahl method: o The sample is decomposed in hot, concentrated sulfuric acid to convert the bound nitrogen to ammonium ion. o The resulting solution is then cooled, diluted, and made basic (usually by NaOH), a process that converts the ammonium ions to ammonia. o The ammonia is distilled from the basic solution, collected in an acidic solution, and determined by a neutralization titration. Some Considerations in the Kjeldahl method: o The critical step in the Kjeldahl method is the decomposition with sulfuric acid, which oxidizes the carbon and hydrogen in the sample to carbon dioxide and water. The fate of the nitrogen, however, depends on its state of combination in the original sample: § Amine and amide nitrogens are quantitatively converted to ammonium ion. § Nitro, azo, and azoxy groups are likely to yield the element or its various oxides, all of which are lost from the hot acidic medium. This loss can be avoided by pretreating the sample with a reducing agent to form amides or amines. In one such prereduction scheme, salicylic acid and sodium thiosulfate are added to the concentrated sulfuric acid solution containing the sample. After a brief period, the digestion is performed in the usual way. § Pyridine, pyridine derivatives, and some other aromatic heterocyclic compounds are particularly resistant to complete decomposition by sulfuric acid. Such compounds yield low results consequently unless special precautions are taken. o The decomposition step is frequently the most time-consuming aspect of a Kjeldahl determination. Some samples may require heating periods more than 1 hour. Numerous modifications of the original procedure have been proposed with the aim of shortening the digestion time. § In the most widely used modification, a neutral salt, such as potassium sulfate, is added to increase the boiling point of the sulfuric acid solution and thus the temperature at which the decomposition occurs. Page 12 of 15 In another modification, a solution of hydrogen peroxide is added to the mixture after the digestion has decomposed most of the organic matrix. Many substances catalyze the decomposition of organic compounds by sulfuric acid. Mercury, copper, and selenium, either combined or in the elemental state, are effective. Mercury(II), if present, must be precipitated with hydrogen sulfide prior to distillation to prevent retention of ammonia as a mercury(II) ammine complex. § o Example of calculation used in Kjeldahl method: A 0.7121 g sample of wheat flour was analyzed by the Kjeldahl method. The ammonia formed by addition of concentrated base after digestion with H2SO4 was distilled into 25.00 mL of 0.04977 M HCl. The excess HCl was then back-titrated with 3.97 mL of 0.04012 M NaOH. Calculate the percent protein in the flour, using the 5.70 factor for cereal. Solution: Step 1. Calculate the number of moles HCl originally present in the receiver. 1 𝑳 𝑯𝑪𝒍 𝑚𝑜𝑙 𝐻𝐶𝑙 𝑛𝑜. 𝑜𝑓 𝑚𝑜𝑙𝑒𝑠 𝐻𝐶𝑙 = 25.00 𝒎𝑳 𝑯𝑪𝒍 × × 0.04977 = 𝟎. 𝟎𝟎𝟏𝟐𝟒𝟒𝟑 𝒎𝒐𝒍 1000 𝒎𝑳 𝑯𝑪𝒍 𝑳 𝑯𝑪𝒍 Step 2. Calculate the number of moles NaOH used in back-titration. 1 𝑳 𝑵𝒂𝑶𝑯 𝑚𝑜𝑙 𝑁𝑎𝑂𝐻 𝑛𝑜. 𝑜𝑓 𝑚𝑜𝑙𝑒𝑠 𝑁𝑎𝑂𝐻 = 3.97 𝒎𝑳 𝑵𝒂𝑶𝑯 × × 0.04012 = 𝟎. 𝟎𝟎𝟎𝟏𝟓𝟗𝟑 𝒎𝒐𝒍 1000 𝒎𝑳 𝑵𝒂𝑶𝑯 𝑳 𝑵𝒂𝑶𝑯 Step 3. Calculate the number of moles N (difference between mol HCl and mol NaOH): 𝑛𝑜. 𝑜𝑓 𝑚𝑜𝑙𝑒𝑠 𝑁 = 𝑚𝑜𝑙 𝐻𝐶𝑙 − 𝑚𝑜𝑙 𝑁𝑎𝑂𝐻 = 0.0012443 𝑚𝑜𝑙 − 0.0001593 𝑚𝑜𝑙 = 𝟎. 𝟎𝟎𝟏𝟎𝟖𝟓𝟎 𝒎𝒐𝒍 Step 4. Calculate the %N in the sample: 14 𝑔 𝑁 0.0010850 𝒎𝒐𝒍 𝑵 × 1 𝒎𝒐𝒍 𝑵 %𝑁 = × 100% = 2.1432% 0.7121 𝑔 𝑠𝑎𝑚𝑝𝑙𝑒 Step 5. Calculate the %protein by multiplying %N by the factor: 5.170% 𝑝𝑟𝑜𝑡𝑒𝑖𝑛 %𝑝𝑟𝑜𝑡𝑒𝑖𝑛 = 2.1432 %𝑵 × = 𝟏𝟐. 𝟏𝟔 % %𝑵 Sulfur Sulfur in organic and biological materials is conveniently determined by burning the sample in a stream of oxygen. The sulfur dioxide formed during the oxidation is collected by distillation into a dilute solution of hydrogen peroxide: SO2 (g) + H2O2 à H2SO4 The sulfuric acid is then titrated with a standard base. B. Determination of Organic Functional Groups Carboxylic Acid and Sulfonic Acid Carboxylic and sulfonic acids are very common organic acids and are compound that are readily titrated. An indicator that changes color in a basic range, such as phenolphthalein, is required. Many carboxylic acids are not sufficiently soluble in water to permit direct titration in aqueous solution. When this problem exists, the acid can be dissolved in ethanol and titrated with aqueous base. Alternatively, the acid can be dissolved in an excess of standard base followed by backtitration with standard acid. Page 13 of 15 Sulfonic acids are generally strong acids that easily dissolve in water. Titration with standard base can be used for the determination. Neutralization titrations are often used to determine the equivalent weights of purified organic acids. Equivalent weights serve as an aid in the qualitative identification of organic acids. In this method, an accurately weighed sample of a pure acid is titrated to a well-defined equivalence point using a strong base with only one replaceable OH-. If we assume that the titration involves the transfer of n protons, then the moles of titrant needed to reach the equivalence point is given as 𝑀𝑜𝑙𝑒𝑠 𝑡𝑖𝑡𝑟𝑎𝑛𝑡 = 𝑛 × 𝑚𝑜𝑙𝑒𝑠 𝑎𝑛𝑎𝑙𝑦𝑡𝑒 (1) and the molecular weight (molar mass) is 𝑀𝑊 = 𝑔 𝑎𝑛𝑎𝑙𝑦𝑡𝑒 (2) 𝑚𝑜𝑙𝑒𝑠 𝑎𝑛𝑎𝑙𝑦𝑡𝑒 Finding the moles analyte from (1) and incorporating it in 2, gives us: 𝑀𝑊 = 𝑛 × 𝑔 𝑎𝑛𝑎𝑙𝑦𝑡𝑒 (3) 𝑚𝑜𝑙𝑒𝑠 𝑡𝑖𝑡𝑟𝑎𝑛𝑡 Since the actual number of protons transferred between the analyte and titrant is uncertain, we define the analyte’s equivalent weight (EW) as the apparent formula weight when n = 1, i.e. 𝐸𝑊 = 𝑔 𝑎𝑛𝑎𝑙𝑦𝑡𝑒 (4) 𝑚𝑜𝑙𝑒𝑠 𝑡𝑖𝑡𝑟𝑎𝑛𝑡 The true formula weight, therefore, can is an integer multiple of the calculated equivalent weight. Thus, if we titrate a weak acid having one replaceable H+ with a strong base, the EW and MW are identical. If the weak acid is diprotic, however, the MW will be twice as large as the EW. Example of calculation used in Equivalent Weight Determination of an Acid: A 0.2521-g sample of an unknown weak acid is titrated with a 0.1005 M solution of NaOH, requiring 42.68 mL to reach the phenolphthalein end point. Determine the compound’s equivalent weight. Which of the following compounds is most likely to be the unknown weak acid? ascorbic acid malonic acid succinic acid citric acid C6H8O6 C3H4O4 C4H6O4 C6H8O7 MW = 176 MW = 104 MW = 118 MW = 192 1 replaceable H+ 2 replaceable H+ 2 replaceable H+ 3 replaceable H+ Solution: Step 1. Find the number of moles titrant need to reach the end point. 𝑚𝑜𝑙 𝑁𝑎𝑂𝐻 𝑛𝑜. 𝑜𝑓 𝑚𝑜𝑙𝑒𝑠 𝑁𝑎𝑂𝐻 = 0.1005 × 0.04268 𝐿 𝑁𝑎𝑂𝐻 𝐿 𝑁𝑎𝑂𝐻 Step 2. Find the equivalent weight of the analyte. 𝐸𝑊 = Page 14 of 15 𝑔 𝑎𝑛𝑎𝑙𝑦𝑡𝑒 0.2521 𝑔 𝑔 = = 58.78 𝑚𝑜𝑙 𝑁𝑎𝑂𝐻 𝑚𝑜𝑙𝑒𝑠 𝑡𝑖𝑡𝑟𝑎𝑛𝑡 𝑚𝑜𝑙 0.1005 × 0.04268 𝐿 𝑁𝑎𝑂𝐻 𝐿 𝑁𝑎𝑂𝐻 Step 3. Find the possible molecular weight by multiplying with an integer corresponding to the number of replaceable H+ ions. Then, compare with the given molecular weights above. For n = 1: For n = 2: For n = 3: 𝑀𝑊 = 1 × 𝐸𝑊 = 58.78 𝑔/𝑚𝑜𝑙 𝑀𝑊 = 2 × 𝐸𝑊 = 117.6 𝑔/𝑚𝑜𝑙 𝑀𝑊 = 3 × 𝐸𝑊 = 176.3 𝑔/𝑚𝑜𝑙 If the weak acid has only one replaceable H+, then the MW must be 58.78 g/mol, eliminating ascorbic acid as a possibility. If the weak acid has 2 replaceable H+, then the MW must be 117.6 g/mo. Succinic acid, with a formula weight of 118.1 g/mol is a possibility, but malonic acid is not. If the weak acid has 3 replaceable H+, then its MW must be 176.3 g/mol, eliminating citric acid, as a possibility. Thus, only succinic acid provides a possible match. Amine Aliphatic amines generally can be titrated directly with a solution of strong acid. Aromatic amines such as aniline and its derivatives, however, are usually too weak for titration in aqueous solutions. Likewise, cyclic amines with aromatic character, such as pyridine and its derivatives, are usually too weak for titration in aqueous solutions. Many saturated cyclic amines, such as piperdine, tend to resemble aliphatic amines in their acid/base behavior and can thus be titrated in aqueous media. Many amines that are too weak to be titrated as bases in water are easily titrated in nonaqueous solvents, such as anhydrous acetic acid, which enhance their basicity. Ester Esters are commonly determined by saponification with a measured quantity of standard base: R1COOR2 + OH- à R1COO- + HOR2 The excess base is then titrated with standard acid. Esters vary widely in their rates of saponification. Some require several hours of heating with a base to complete the process, while a few react rapidly enough that direct titration with standard base is feasible. Typically, the ester is refluxed with standard 0.5 M KOH for 1 to 2 hours. After cooling, the excess base is titrated with standard acid. Page 15 of 15