Anaesthesia Lecture 24 Dr Maha PDF
Document Details
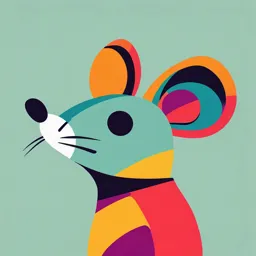
Uploaded by AthleticBeech
University of Baghdad, College of Dentistry
Dr Maha Mohsin Khalaf
Tags
Summary
These lecture notes cover pharmacology related to anesthesia. They discuss patient factors and different types of pre-anesthesia treatment, such as H2 blockers and benzodiazepines, and anesthetic agents, including general and local anesthetics.
Full Transcript
Pharmacology Dr Maha Mohsin Khalaf College of Dentistry University of Baghdad Introduction For patients undergoing surgical or medical procedures,...
Pharmacology Dr Maha Mohsin Khalaf College of Dentistry University of Baghdad Introduction For patients undergoing surgical or medical procedures, different levels of sedation can provide important benefits to facilitate procedural interventions. These levels of sedation range from anxiolysis to general anesthesia and can create: Sedation and reduced anxiety. Lack of awareness and amnesia. Skeletal muscle relaxation. Suppression of undesirable reflexes. Analgesia. Because no single agent provides all desired objectives, several categories of drugs are combined to produce the optimum level of sedation required. Anesthetics are agents that bring about reversible loss of sensation and consciousness. They are divided into two main groups: general anesthetics and local anesthetics. Drugs are chosen to provide safe and efficient sedation based on the type and duration of the procedure and patient characteristics, such as organ function, medical conditions, and concurrent medications. Preoperative medications provide anxiolysis and analgesia, and mitigate unwanted side effects of the anesthetic or the procedure itself. Neuromuscular blockers enable endotracheal intubation and muscle relaxation to facilitate surgery. Potent general anesthetic medications are delivered via inhalation and/or intravenously. Except for nitrous oxide, inhaled anesthetics are volatile, halogenated hydrocarbons, while intravenous (IV) anesthetics consist of several chemically unrelated drug classes commonly used to rapidly induce and/or maintain a state of general anesthesia. PATIENT FACTORS IN SELECTION OF ANESTHESIA Drugs are chosen to provide safe and efficient anesthesia based on the type of procedure and patient characteristics such as organ function, medical conditions, and concurrent medications. A. Status of organ systems 1. Cardiovascular system: Anesthetic agents suppress cardiovascular function to varying degrees. This is an important consideration in patients with coronary artery disease, heart failure, dysrhythmias, valvular disease, and other cardiovascular disorders. Hypotension may develop during anesthesia, resulting in reduced perfusion pressure and ischemic injury to tissues. 1 Treatment with vasoactive agents may be necessary. Some anesthetics, such as halothane, sensitize the heart to arrhythmogenic effects of sympathomimetic agents. 2. Respiratory system: Respiratory function must considered for all anesthetics. Asthma and ventilation or perfusion abnormalities complicate control of inhalation anesthetics. Inhaled agents depress respiration but also act as bronchodilators. IV anesthetics and opioids suppress respiration. These effects may influence the ability to provide adequate ventilation and oxygenation during and after surgery. 3. Liver and kidney: The liver and kidneys influence long-term distribution and clearance of drugs and are also target organs for toxic effects. Release of fluoride, bromide, and other metabolities of halogenated hydrocarbons can affect these organs, especially if they accumulate with frequently repeated administration of anesthetics. 4. Nervous system: The presence of neurologic disorders (for example, epilepsy, myasthenia gravis, neuromuscular disease, compromised cerebral circulation) influences the selection of anesthetic. 5. Pregnancy: Special precautions should be observed when anesthetics and adjunctive agents are administered during pregnancy. Effects of fetal organogenesis are a major concern in early pregnancy. Transient use of nitrous oxide may cause aplastic anemia in the fetus. Oral clefts have occurred in fetuses when mothers received benzodiazepines in early pregnancy. Benzodiazepines should not be used during labor because of resultant temporary hypotonia and altered thermoregulation in the new born. B. Concomitant use of drugs 1. Multiple adjunct agents: Commonly, patients receive one or more of these preanesthetic medications: H2 blockers (famotidine, ranitidine) to reduce gastric acidity. benzodiazepines (midazolam, diazepam) to allay anxiety and facilitate amnesia. nonopioids (acetaminophen, celecoxib) or opioids (fentanyl) for analgesia. antihistamines (diphenhydramine) to prevent allergic reactions. antiemetics (ondansetron) to prevent nausea. and/or anticholinergics (glycopyrrolate) to prevent bradycardia and secretion of fluids in to the respiratory tract. Premedications facilitate smooth induction of anesthesia and lower required anesthetic doses. However, they can also enhance undesirable anesthetic effects (hypoventilation) and, when coadministered, may produce negative effects not observed when given individually. 2. Concomitant user of other drugs: Patients may take medications for underlying diseases or abuse drugs that alter response to anesthetics. For example, alcoholics have elevated levels of liver enzymes that metabolize anesthetics, and drug abusers may be tolerant to opioids. 2 LEVELS OF SEDATION The levels of sedation occur in a dose-related continuum, which is variable and depends on individual patient response to various drugs. These “artificial” levels of sedation start with light sedation (anxiolysis), continue to moderate sedation, then deep sedation, and finally to a state of general anesthesia. The hallmarks of escalation from one level to the next are recognized by changes in mentation, hemodynamic stability, and respiratory competency. This escalation in levels is often very subtle and unpredictable; therefore, the sedation provider must always be ready to manage the unanticipated next level of sedation. STAGES OF GENERAL ANESTHESIA General anesthesia is a reversible state of central nervous system (CNS) depression, causing loss of response to and perception of stimuli. The state of general anesthesia can be divided into three stages: induction, maintenance, and recovery. Induction is the time from administration of a potent anesthetic to development of unconsciousness, while maintenance is the sustained period of general anesthesia. Recovery starts with the discontinuation of the anesthetic and continues until the return of consciousness and protective reflexes. Induction of anesthesia depends on how fast effective concentrations of anesthetic reach the brain. Recovery is essentially the reverse of induction and depends on how fast the anesthetic diffuses from the brain. The depth of general anesthesia is the degree to which the CNS is depressed, as evident in electroencephalograms A. Induction General anesthesia in adults is normally induced with an IV agent such as propofol, producing unconsciousness in 30 to 40 seconds. Often an IV neuromuscular blocker such as rocuronium, vecuronium, or succinylcholine is administered to facilitate endotracheal intubation by eliciting muscle relaxation. For children without IV access, nonpungent volatile agents, such as sevoflurane, are administered via inhalation to induce general anesthesia. B. Maintenance of anesthesia After administering the induction drug, vital signs and response to stimuli are vigilantly monitored to balance the amount of drug continuously inhaled or infused to maintain general anesthesia. Maintenance is commonly provided with volatile anesthetics, although totalintravenous anesthesia (TIVA) with drugs such as propofol can be used to maintain general anesthesia. Opioids such as fentanyl are used for analgesia along with inhalation agents, because the latter alter consciousness but not perception of pain. C. Recovery After cessation of the maintenance anesthetic drug, the patient is evaluated for return of consciousness. For most anesthetic agents, redistribution from the site of action (rather than metabolism of the drug) underlies recovery. Neuromuscular blocking drugs are typically reversed after completion of surgery, unless enough time has elapsed for their metabolism. The patient is 3 monitored to assure full recovery of all normal physiologic functions (spontaneous respiration, blood pressure, heart rate, and all protective reflexes). Depth of anesthesia The depth of anesthesia has four sequential stages characterized by increasing CNS depression as the anesthetic accumulates in the brain [Note: These stages were defined for the original anesthetic ether, which produces a slow onset of anesthesia. With modern anesthetics, the stages merge because of the rapid onset of stage III.] 1. Stage I—Analgesia: Loss of pain sensation results from interference with sensory transmission in the spinothalamic tract. The patient progresses from conscious and conversational to drowsy. Amnesia and reduced awareness of pain occur as stage II is approached. 2. Stage II—Excitement: The patient displays delirium and possibly combative behavior. A rise and irregularity in blood pressure and respiration occur, as well as a risk of laryngospasm. To shorten or eliminate this stage, rapid-acting IV agents are given before inhalation anesthesia is administered. 3. Stage III—Surgical anesthesia: There is gradual loss of muscle tone and reflexes as the CNS is further depressed. Regular respiration and relaxation of skeletal muscles with eventual loss of spontaneous movement occur. This is the ideal stage of surgery. Careful monitoring is needed to prevent undesired progression to stage IV. 4. Stage IV—Medullary paralysis: Severe depression of the respiratory and vasomotor centers occurs. Ventilation and/or circulation must be supported to prevent death. INHALATION ANESTHETICS Inhaled gases are used primarily for maintenance of anesthesia after administration of an IV drug. Depth of anesthesia can be rapidly altered by changing the inhaled gas concentration. Inhalational agents have steep dose–response curves with very narrow therapeutic indices, so the difference in concentrations from eliciting general anesthesia to cardiopulmonary collapse is small. No antagonists exist. To minimize waste, inhaled gases are delivered in a recirculation system that contains absorbents to remove carbon dioxide and allow rebreathing of the gas. Common features of inhalation anesthetics Modern inhalation anesthetics are nonflammable, nonexplosive agents, which include nitrous oxide and volatile, halogenated hydrocarbons. These agents decrease cerebrovascular resistance, resulting in increased brain perfusion. They cause bronchodilation but also decrease both respiratory drive and hypoxic pulmonary vasoconstriction (increased pulmonary vascular resistance in poorly oxygenated regions of the lungs, redirecting blood flow to better oxygenated 4 regions). Movement of these gases from the lungs to various body compartments depends upon their solubility in blood and tissues, as well as on blood flow. Mechanism of action No specific receptor has been identified as the locus to create a state of general anesthesia. The fact that chemically unrelated compounds produce unconsciousness argues against the existence of a single receptor, and it appears that a variety of molecular mechanisms may contribute to the activity of anesthetics. At clinically effective concentrations, general anesthetics increase the sensitivity of the γ-aminobutyric acid (GABAA) receptors to the inhibitory neurotransmitter GABA. This increases chloride ion influx and hyperpolarization of neurons. Postsynaptic neuronal excitability and, thus, CNS activity are diminished. Unlike other anesthetics, nitrous oxideand ketamine do not have actions on GABAA receptors. Their effects are mediated via inhibition of N-methyl-d-aspartate (NMDA) receptors. [Note: The NMDA receptor is a glutamate receptor, which is the body’s main excitatory neurotransmitter.] Receptors other than GABA that are affected by volatile anesthetics include the inhibitory glycine receptors found in the spinal motor neurons. Additionally, inhalation anesthetics block excitatory postsynaptic currents found on nicotinic receptors. However, the mechanisms by which anesthetics perform these modulatory roles are not fully understood. Isoflurane Isoflurane, like other halogenated gases, produces dose-dependent hypotension predominantly from relaxation of systemic vasculature. Hypotension can be treated with a direct-acting vasoconstrictor, such as phenylephrine. Because it undergoes little metabolism, isoflurane is considered nontoxic to the liver and kidney. Its pungent odor stimulates respiratory reflexes (breath holding, salivation, coughing, laryngospasm), so it is not used for inhalation induction. With a higher blood solubility than Desflurane and sevoflurane, isoflurane takes longer to reach equilibrium, making it less ideal for short procedures; however, its low cost makes it a good option for longer surgeries. Desflurane Desflurane provides very rapid onset and recovery due to low blood solubility. This makes it a popular anesthetic for short procedures. It has a low volatility, which requires administration via a special heated vaporizer. Like isoflurane, it decreases vascular resistance and perfuses all major tissues very well. Desflurane has significant respiratory irritation like isoflurane so it should not be used for inhalation induction. Its degradation is minimal and tissue toxicity is rare. Higher cost occasionally prohibits its use. Sevoflurane Sevoflurane has low pungency or respiratory irritation. This makes it useful for inhalation induction, especially with pediatric patients who do not tolerate IV placement. It has a rapid onset and recovery due to low blood solubility. Sevoflurane has low hepatotoxic potential, but 5 compounds formed from reactions in the anesthesia circuit (soda lime) may be nephrotoxic with very low fresh gas flow that allows longer chemical reaction time. Nitrous oxide Nitrous oxide (“laughing gas”) is a nonirritating potent sedative that is unable to create a state of general anesthesia. It is frequently used at concentrations of 30% to 50% in combination with oxygen to create moderate sedation, particularly in dentistry. Nitrous oxide does not depress respiration and maintains cardiovascular hemodynamics as well as muscular strength. Nitrous oxide can be combined with other inhalational agents to establish general anesthesia, which lowers the required concentration of the combined volatile agent. This gas admixture further reduces many unwanted side effects of the other volatile agent that impact cardiovascular output and cerebral blood flow. Nitrous oxide is poorly soluble in blood and other tissues, allowing it to move very rapidly in and out of the body. This can be problematic in closed body compartments because nitrous oxide can increase the volume (exacerbating a pneumothorax) or pressure (sinus or middle ear pressure); it replaces nitrogen in various air spaces faster than the nitrogen leaves. Its speed of movement allows nitrous oxide to retard oxygen uptake during recovery, thereby causing “diffusion hypoxia.” This can be overcome by delivering high concentrations of inspired oxygen during recovery. Ether Diethyl ether (ether) is one of the old anesthetic methods used for anesthesia. It is a highly volatile and inflammable liquid. The vapors of ethers cause irritation causing increased bronchial secretions; therefore, atropine is used in the preanesthetic medication. However, ether is a potent anesthetic. Due to this property, it reduces the dose of competitive neuromuscular blockers while on administration it produces adequate muscle relaxation. For ether anesthesia, no major equipment is required and it is relatively simple. Since it is a highly lipophilic substance, recovery is slow. Respiration and blood pressure do not get affected during the anesthesia. Use of ether is limited only whereas it is completely banned from use in developed countries. Halothane Halothane is a noninflammable, nonirritant, photosensitive volatile agent with a sweet smell. It is a liquid at room temperature and it is administered with a special vaporizer at a concentration of 2% to 4% for induction followed by 0.5% to 1% for maintenance. A precise control of the concentration of halothane is essential as it can cause direct effect on heart by interfering with calcium, thus causing depression of myocardial contractility. Dose-dependent fall in blood pressure is known during halothane anesthesia. It directly suppresses respiratory centers, thereby reducing ventilatory response to blood carbon dioxide levels. Decreased alveolar ventilation can cause increase in arterial CO2 levels without compensatory increase in ventilation. Therefore, continuous arterial gas monitoring is essential during its usage. Halothane dilates cerebral blood vessels and increases cerebral blood flow which in turn causes increased intracranial pressure, especially in patients with other susceptible comorbid conditions in the brain. It causes relaxation of skeletal muscle and potentiates the actions of competitive 6 neuromuscular blockers. Halothane causes uterine smooth muscle relaxation. Although it is useful for the manipulation of fetus orientation, it is not used due to its action of inhibiting uterine contraction during parturition. More than 60% of the inhalationally administered halothane is eliminated through lungs on recovery within 24 hours. The rest of halothane is metabolized by liver. Trifluoroacetic acid is a major metabolite which can cause hepatic injury (halothane-induced hepatic necrosis). This syndrome of hepatic necrosis is reported in 1 in 10,000 patients and it is referred to as “halothane hepatitis.” Malignant hyperthermia In a very small percentage of susceptible patients, exposure to halogenated hydrocarbon anesthetics (or succinylcholine) may induce malignant hyperthermia (MH), a rare life-threatening condition. MH causes a drastic and uncontrolled increase in skeletal muscle oxidative metabolism, overwhelming the body’s capacity to supply oxygen, remove carbon dioxide, and regulate temperature, eventually leading to circulatory collapse and death if not treated immediately. Strong evidence indicates that MH is due to an excitation–contraction coupling defect. Burn victims and individuals with muscular dystrophy, myopathy, myotonia, and osteogenesis imperfecta are susceptible to MH-like events and caution should be taken. Susceptibility to MH is often inherited as an autosomal dominant disorder. Should a patient exhibit symptoms of MH, dantrolene is given as the anesthetic mixture is withdrawn, and measures are taken to rapidly cool the patient. Dantrolene blocks release of Ca2+ from the sarcoplasmic reticulum of muscle cells, reducing heat production and relaxing muscle tone. It should be available whenever triggering agents are administered. In addition, the patient must be monitored and supported for respiratory, circulatory, and renal problems. Use of dantrolene and avoidance of triggering agents such as halogenated anesthetics in susceptible individuals have markedly reduced mortality from MH. INTRAVENOUS ANESTHETICS IV anesthetics cause rapid induction of anesthesia often occurring in 1 minute or less. It is the most common way to induce anesthesia before maintenance of anesthesia with an inhalation agent. IV anesthetics may be used as single agents for short procedures or administered as infusions to help maintain anesthesia during longer surgeries. In lower doses, they may be used solely for sedation. A. Induction After entering the blood, a percentage of drug binds to plasma proteins, and the rest remains unbound or “free.” The degree of protein binding depends upon the physical characteristics of the drug, such as the degree of ionization and lipid solubility. Unbound, lipid-soluble, nonionized molecules cross into the brain most quickly. Like inhalational anesthetics, the exact mode of action of IV anesthetics is unknown; however, GABA likely plays a large role. 7 B. Recovery The recovery phase is short as these drugs are rapidly redistributed from the CNS into the various compartments of the body. After initial flooding of the CNS and other vessel-rich tissues with nonionized molecules, the drug diffuses into other tissues with less blood supply. With secondary tissue uptake, predominantly skeletal muscle, plasma concentration of the drug falls. This allows the drug to diffuse out of the CNS. This initial redistribution of drug into other tissues leads to the rapid recovery seen after a single IV dose of the induction agent. The final elimination occurs via kidneys or liver. Metabolism and plasma clearance become important only following infusions and repeat doses of a drug. Adipose tissue makes little contribution to the early redistribution of free drug following a bolus, due to its poor blood supply. However, following repeat doses or infusions, equilibration with fat tissue forms a drug reservoir, often leading to delayed recover Propofol Is an IV sedative/hypnotic used for induction and/or maintenance of anesthesia. It is widely used and has replaced thiopental as the first choice for induction of general anesthesia and sedation. Because propofol is poorly water soluble, it is supplied as an emulsion containing soybean oil and egg phospholipid, giving it a milk-like appearance. Onset: Induction is smooth and occurs 30 to 40 seconds after administration. Plasma levels decline rapidly as a result of redistribution, followed by a more prolonged period of hepatic metabolism and renal clearance. The initial redistribution half-life is 2 to 4 minutes. The pharmacokinetics of propofol are not altered by moderate hepatic or renal failure. Actions: Although propofol depresses the CNS, it occasionally contributes to excitatory phenomena, such as muscle twitching, spontaneous movement, yawning, and hiccups. Transient pain at the injection site is common. Propofol decreases blood pressure without significantly depressing the myocardium. It also reduces intracranial pressure, mainly due to decreased cerebral blood flow and oxygen consumption. It has less of a depressant effect than volatile anesthetics on CNS-evoked potentials, making it useful for surgeries in which spinal cord function is monitored. It does not provide analgesia, so supplementation with narcotics is required. Propofol is commonly infused in lower doses to provide sedation. The incidence of postoperative nausea and vomiting is very low secondary to its antiemetic properties. Barbiturates Thiopental is an ultra short–acting barbiturate with high lipid solubility. It is a potent anesthetic but a weak analgesic. Barbiturates require supplementary analgesic administration during anesthesia. When given IV, agents such as thiopental and methohexital quickly enter the CNS and depress function, often in less than 1 minute. However, diffusion out of the brain can also occur very rapidly because of redistribution to other tissues. These drugs may remain in the body for relatively long periods, because only about 15% of a dose entering the circulation is metabolized by the liver per hour. Thus, metabolism of thiopental is much slower than its redistribution. Barbiturates tend to decrease blood pressure, which may cause a reflex tachycardia. They 8 decrease intracranial pressure through reductions in cerebral blood flow and oxygen consumption. Methohexital is still commonly used for electroconvulsive therapy. Benzodiazepines The benzodiazepines are used in conjunction with anesthetics for sedation and amnesia. The most commonly used is midazolam. Diazepam and Lorazepam are alternatives. All three facilitate amnesia while causing sedation, enhancing the inhibitory effects of various neurotransmitters, particularly GABA. Minimal cardiovascular depressant effects are seen, but all are potential respiratory depressants (especially when administered IV). They are metabolized by the liver with variable elimination half-lives, and erythromycin may prolong effects of midazolam. Benzodiazepines can induce a temporary form of anterograde amnesia in which the patient retains memory of past events, but new information is not transferred into long-term memory. Therefore, important treatment information should be repeated to the patient after the effects of the drug have worn off. Opioids Because of their analgesic property, opioids are commonly combined with other anesthetics. The choice of opioid is based primarily on the duration of action needed. The most commonly used opioids are fentanyl and its congeners, sufentanil and remifentanil, because they induce analgesia more rapidly than morphine. They may be administered intravenously, epidurally, or intrathecally (into the cerebrospinal fluid). Opioids are not good amnestics, and they can all cause hypotension and respiratory depression, as well as nausea and vomiting. Opioid effects can be antagonized by naloxone. Etomidate is a hypnotic agent used to induce anesthesia, but it lacks analgesic activity. Its water solubility is poor, so it is formulated in a propylene glycol solution. Induction is rapid, and the drug is short- acting. Among its benefits are little to no effect on the heart and systemic vascular resistance. Etomidate is usually only used for patients with cardiovascular dysfunction or patients who are acutely critically ill. It inhibits 11-β hydroxylase involved in steroidogenesis, and adverse effects may include decreased plasma cortisol and aldosterone levels. Etomidate should not be infused for an extended time, because prolonged suppression of these hormones is dangerous. Injection site pain, involuntary skeletal muscle movements, and nausea and vomiting are common. Ketamine A short-acting anti-NMDA receptor anesthetic and analgesic, induces a dissociated state in which the patient is unconscious (but may appear to be awake) with profound analgesia. Ketamine stimulates central sympathetic outflow, causing stimulation of the heart with increased blood pressure and CO. It is also a potent bronchodilator. Therefore, it is beneficial in patients with hypovolemic or cardiogenic shock as well as asthmatics. Conversely, it is contraindicated in hypertensive or stroke patients. The drug is lipophilic and enters the brain very quickly. Like the barbiturates, it redistributes to other organs and tissues. Ketamine has become popular as an 9 adjunct to reduce opioid consumption during surgery. Of note, it may induce hallucinations, particularly in young adults, but pretreatment with benzodiazepines may help. Ketamine may be used illicitly, since it causes a dream-like state and hallucinations similar to phencyclidine (PCP) NEUROMUSCULAR BLOCKERS Neuromuscular blockers are crucial to the practice of anesthesia and used to facilitate endotracheal intubation and provide muscle relaxation when needed for surgery. Their mechanism of action is via blockade of nicotinic acetylcholine receptors on the skeletal muscle cell membrane. These agents include cisatracurium, mivacurium, pancuronium, rocuronium, succinylcholine, and vecuronium. LOCAL ANESTHETICS Local anesthetics provide a reversible regional loss of sensation. They reduce pain and thereby facilitate surgical procedures. Local anesthetics block nerve conduction of sensory impulses and in higher concentrations block motor impulses from the periphery to the CNS. Sodium ion channels are blocked to prevent the transient increase in permeability of the nerve membrane to Na+ that is required for an action potential. When propagation of action potentials is prevented, sensation cannot be transmitted from the source of stimulation to the brain. Cocaine is a naturally occurring compound indigenous to the Andes Mountains, West Indies, and Java. It was the first anesthetic to be discovered and is the only naturally occurring local anesthetic; however, it is not used clinically because of its adverse effects and abuse potential. All other anesthetics are synthetically derived. Procaine, the first synthetic derivative of cocaine, was developed in 1904. All local anesthetics have a similar chemical structure, which consists of three components: aromatic portion, intermediate chain, and amine group. Structurally, all local anesthetics include a lipophilic group joined by an amide or ester linkage to a carbon chain, which, in turn, is joined to a hydrophilic group. The degree of lipid solubility of each anesthetic is an important property because the lipid solubility enables its diffusion through the highly lipophilic nerve membrane. The extent of an anesthetic’s lipophilicity is directly related to its potency. All local anesthetics are weak bases that require the addition of hydrochloride salt in order to be water soluble and therefore injectable. Salt equilibrates between an ionized form and a nonionized form in aqueous solution. Equilibration is crucial because, although the ionized form is injectable, it is the nonionized base which has the lipophilic properties and is responsible for their diffusion into the nerve cell membrane. The duration of action of an anesthetic is determined by their protein-binding capacity to anesthetic receptors along the nerve cell membrane. The intermediate chain, which connects the aromatic and amine portions, is composed of either an ester or an amide linkage. This intermediate chain can be used in classifying local anesthetics into esters and amides. Ester-type local anesthetics have a very short plasma half-life. They are metabolized by plasma butyrylcholinesterase. In patients with decreased or atypical cholinesterase, the plasma levels of these anesthetics may be higher than usual. Amide-type localanesthetics are metabolized at varying rates and to a varying extent by hepatic isozymes and are excreted in an unchanged form by the kidney. The rate of metabolism 10 is decreased in case of liver impairment or by drugs interfering with the microsomal enzyme system. The most widely used local anesthetics are bupivacaine, lidocaine, mepivacaine, ropivacaine, and tetracaine. Delivery techniques of local anesthetics Various delivery techniques broaden the clinical applicability of local anesthetics. These techniques include topical administration, infiltration, perineural, and neuraxial (spinal, epidural, or caudal) blocks. Small, unmyelinated nerve fibers for pain, temperature, and autonomic activity are most sensitive. These are as follows. 1. Regional anesthesia: It makes a specific part of the body numb to relieve pain or allow surgical procedures to be done. Local anesthetics are safer than general or systemic anesthetics; therefore, they are preferred whenever possible. 2. Surface anesthesia: It is used during ocular tonometry, intubation, and endoscopic procedures. Tetracaine 2% and lidocaine2% to 4% are used. The anesthetic effect is superficial and does not last long. 3. Infiltration anesthesia: The local anesthetic is injected directly into the skin or deeper structures to carry out incision, excision, hydrocoele, herniorraphy, incision and drainage of an abscess, etc. Lignocaine and bupivacaine are generally used for infiltration anesthesia. 4. Field block: The local anesthetic is injected subcutaneously proximal to the site where anesthesia is desired. All nerves distal to the site of injection are blocked. Lidocaine (1% to 2%) is used for intermediate duration of anesthesia in dental extraction, operation on forearms, legs, herrniorraphy, etc. 5. Nerve block: The local anesthetic is injected around a nerve plexus or a nerve trunk. This results in a large area of anesthesia. Thus even with a small amount of drug, a large area of anesthesia can be produced. Lidocaine is most commonly used apart from bupivacaine. Intercostal, sciatic, brachial plexus, facial, and phrenic are commonly performed nerve blocks. 6. Spinal anesthesia: A single dose of the local anesthetic is injected into the subarachnoid space in the lower end of the spinal cord (lumbar region). The site of action is nerve roots, resulting in loss of pain sensation and paralysis of the lower abdomen and hind limbs. Lidocaine, bupivacaine, and tetracaine are most commonly used for spinal anesthesia. The duration of anesthesia depends on the concentration and the drug used. Spinal anesthesia is associated with various complications such as respiratory paralysis, hypotension, headache, nausea, vomiting, bradycardia, and septic meningitis. It is used during operations on lower limbs, pelvis, lower abdomen, etc. 7. Epidural anesthesia: The local anesthetic is injected into the epidural space where it acts upon the nerve roots and relieves pain from large areas of the body by stopping pain signals traveling along the nerves in the spine. Epidural anesthesia is similar to but not the same as spinal anesthesia. In epidural anesthesia, local anesthetic is administered continuously through an 11 epidural catheter that does not reach the CSF and provides long-term anesthesia and pain relief. The local anesthetics most commonly used for this procedure are lidocaine and bupivacaine. Epidural anesthesia is used during labor to relieve pain or during a cesarean section. It can also be used to reduce the amount of general anesthesia needed during some operations and can provide pain relief postoperatively. In knee and hip replacement surgery, epidural anesthesia can be used in place of a general anesthetic. Actions All local anesthetics cause vasodilation, which leads to a rapid diffusion away from the site of action and short duration when these drugs are administered alone. By adding the vasoconstrictor epinephrine, the rate of local anesthetic absorption and diffusion is decreased. This minimizes systemic toxicity and increases the duration of action. However, epinephrine should not be coadministered for nerve block in extremities such as fingers and toes as vasoconstriction of end arteries may lead to ischemia and necrosis. It should be used with caution in patients with thyrotoxicosis or cardiovascular disease and in labor. Hepatic function does not affect the duration of action of local anesthesia because that is determined by redistribution rather than biotransformation. Some local anesthetics have other therapeutic uses (for example, lidocaine is an IV antiarrhythmic). Onset, potency, and duration of action The onset of action of local anesthetics is influenced by several factors including tissue pH, nerve morphology, concentration, pKa, and lipid solubility of the drug. Of these, the pKa is most important. Local anesthetics with a lower pKa have a quicker onset, since more drug exists in the unionized form at physiologic pH, thereby allowing penetration of the nerve cell membrane. Once at the nerve membrane, the ionized form interacts with the protein receptor of the Na+ channel to inhibit its function and achieve local anesthesia. The pH may drop in infected sites, causing onset to be delayed or even prevented. Potency and duration of these agents depend mainly on lipid solubility, with higher solubility correlating with increased potency and duration of action. Metabolism Biotransformation of amides occurs primarily in the liver. Prilocaine, a dental anesthetic, is also metabolized in the plasma and kidney, and one of its metabolites may lead to methemoglobinemia. Esters are biotransformed by plasma cholinesterase (pseudocholinesterase). Patients with pseudocholinesterase deficiency may metabolize ester local anesthetics more slowly. At normal doses, this has little clinical effect. Reduced hepatic function predisposes patients to toxic effects, but should not significantly increase the duration of action of local anesthetics. Allergic reactions Patient reports of allergic reactions to local anesthetics are fairly common, but often times reported “allergies” are actually side effects from the coadministered epinephrine. True allergy 12 to an amide local anesthetic is exceedingly rare, while the ester procaine is more allergenic and has largely been removed from the market. Allergy to one ester rules out use of another ester, because the allergenic component is the metabolite para-aminobenzoic acid, produced by all esters. By contrast, allergy to one amide does not rule out the use of another amide. A patient may be allergic to other compounds in the local anesthetic, such as preservatives in multidose vials. Local anesthetic systemic toxicity Toxic blood levels of a local anesthetic may be due to repeated injections or could result from a single inadvertent IV injection. Each drug has a weight-based toxic threshold that should be calculated. This is especially important in children, the elderly, and women in labor (who are more susceptible to local anesthetics). Aspiration before every injection is imperative. The signs, symptoms, and timing of local anesthetic systemic toxicity (LAST) are unpredictable. One must consider the diagnosis in any patient with altered mental status, seizures, or cardiovascular instability following injection of local anesthetic. Treatment for LAST may include seizure suppression, airway management, and cardiopulmonary support. Administering a 20% lipid emulsion infusion (lipid rescue therapy) is a valuable asset. ANESTHETIC ADJUNCTS Adjuncts are a critical part of the practice of anesthesia and include drugs that affect gastrointestinal (GI) motility, postoperative nausea and vomiting (PONV), anxiety, and analgesia. Adjuncts are used in collaboration to help make the anesthetic experience safe and pleasant. Gastrointestinal medications H2-receptor antagonists (for example, ranitidine) and proton-pump inhibitors (for example, omeprazole) help to reduce gastric acidity in the event of an aspiration. Nonparticulate antacids (sodium citrate/citric acid) are given occasionally to quickly increase the pH of stomach contents. These drugs are used in the obstetric population going to surgery, along with other patients with reflux. Finally, a dopamine receptor antagonist (metoclopramide) can be used as a prokinetic agent to speed gastric emptying and increase lower esophageal sphincter tone. Medications for PONV PONV can be a significant problem during and after surgery for both the clinician and the patient. Risk factors for PONV include female gender, nonsmoker, use of volatile and nitrous anesthetics, duration of surgery, and postoperative narcotic use. 5-HT3 receptor antagonists (for example, ondansetron) are commonly used to prevent PONV and are usually administered toward the end of surgery. Caution is advised in patients with long QT intervals on ECG. An anticholinergic and antihistamine (promethazine) can also be used; however, sedation, delirium, and confusion can complicate the postoperative period, especially in the elderly. Glucocorticoids such as dexamethasone can be used to reduce PONV. The mechanism is unclear, but because of a longer onset, these agents are usually given at the start of surgery. The neurokinin-1 antagonist aprepitant has also been shown to reduce PONV. Lastly, transdermal scopolamine is given 13 preoperatively to patients with multiple risk factors or to a patient with a history of PONV. Caution is advised because it can produce central anticholinergic effects. Anxiety medications Anxiety is a common part of the surgical experience. Benzodiazepines (midazolam, diazepam), α2 agonists (clonidine, dexmedetomidine), and H1-receptor antagonists (diphenhydramine) can be used to alleviate anxiety. Benzodiazepines also elicit anterograde amnesia, which can help promote a more pleasant surgical experience. Analgesia While opioids are a mainstay in anesthesia for pain control, multimodal analgesia is becoming more common due to the long-term risks of opioid consumption in surgical patients. Nonsteroidal anti-inflammatory drugs (ketorolac, celecoxib) are common adjuncts to opioids. Caution should be used in coagulopathy, GI mucosal and platelet aggregation susceptible patients. Paracetamol can be used both PO and IV, but caution is advised in impaired hepatic function. Analogs of GABA (gabapentin, pregabalin) are becoming more common as pretreatment to reduce opioid consumption both during and after surgery. They also have multiple uses in neuropathic pain and addiction medicine. The NMDA antagonist ketamine is used to reduce overall opioid consumption both intra- and postoperatively. 14