Advances in Controlled Release Fertilizers: Cost-Effective Coating Techniques and Smart Stimuli-Responsive Hydrogels PDF
Document Details
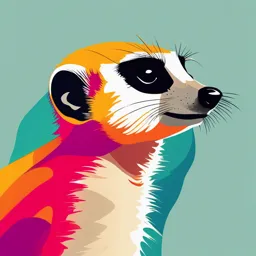
Uploaded by pepinos
Mohammed VI Polytechnic University
2023
Houssameddine Mansouri, Hamid Ait Said, Hassan Noukrati, Abdallah Oukarroum, Hicham Ben youcef, and François Perreault
Tags
Related
- Polymer-based and stimulus-responsive carriers for controlled release of agrochemicals PDF
- Controlled Release Fertilizers for Climate-Smart Agriculture PDF
- Kinetics, Absorption, and Diffusion of Crosslinked Chitosan Hydrogels PDF
- Potassium Release Kinetics & Water Retention of Controlled-Release Fertilizers PDF
- pH-Responsive Eco-Friendly Chitosan-Chlorella Hydrogel Beads PDF
- Controlled Release Tablets - L7 PDF
Summary
This review examines advancements in controlled-release fertilizers (CRF) and their potential to improve fertilizer efficiency in agriculture. It discusses cost-effective coating strategies, smart stimuli-responsive coatings, and the challenges of current applications, alongside highlighting the environmentally friendly aspects and highlighting promising avenues for future research.
Full Transcript
REVIEW www.advsustainsys.com Advances in Controlled Release Fertilizers: Cost-Effective Coating Techniques and Smart Stimuli-Responsive Hydrogels Houssameddine Mansouri, Hamid A...
REVIEW www.advsustainsys.com Advances in Controlled Release Fertilizers: Cost-Effective Coating Techniques and Smart Stimuli-Responsive Hydrogels Houssameddine Mansouri, Hamid Ait Said, Hassan Noukrati, Abdallah Oukarroum, Hicham Ben youcef, and François Perreault* situation since they operate as a direct To meet the needs of a rapidly expanding global population, farmers will need source of nutrients for the soil. Chem- more fertilizers than ever before to maintain a steady supply of affordable, ical fertilizers are now extensively ap- nutritious food. The formulation of controlled release fertilizers (CRF) to plied by farmers all over the world since synchronize nutrient release according to the demand of plants has emerged they can supply all the vital elements that plants require for development. These as a viable solution to the current problems associated with the poor nutrient nutrients are categorized into macronu- usage efficiency of fertilizers. Yet, the greatest obstacle that still stands in the trients and micronutrients, based on how way of broad use of CRF in agriculture is their expensive manufacturing costs. much they are needed for plant growth. The first section of this analysis focuses on broad topics related to CRF. Because they are required in larger quan- Afterward, the differences between several cost-effective raw materials and tities, macronutrients, namely nitrogen (N), potassium (K), and phosphorus (P), some of the production techniques used to make CRF are examined. have been the focus of manufacturing ef- Furthermore, the emerging field of “smart” coating materials, such as forts by the fertilizer industry. stimuli-responsive coatings, which can accurately tailor nutrients delivery to Despite the increase in agricultural the demands of the vegetation, is discussed, and the most important research productivity enabled by intensive agricul- work that could lead to their extensive use in agriculture is pointed out. The tural practices, chemical fertilizers still purpose of this review is to provide a strong assessment of CRF’s have a nutrient use efficacy (NUE) of less than 30%, with phosphorus fertiliz- development over the past several years by highlighting innovations and ers having an even lower NUE of less providing in-depth analysis of prevailing patterns to better understand the than 20%. The excess fertilizer that future of agriculture. is not quickly absorbed by plants will become unavailable for plant use due to microbiological, chemical, or physical reactions (Figure 1). This poor NUE 1. Introduction can lead to major environmental consequences. For example, leaching of nitrogen as nitrous oxide and nitrates, caused by the Nutrients are required for plants to grow and complete their life excess use of nitrogen fertilizers, leads to the eutrophication of cycle. Those nutrients are present in soil naturally, but their quan- aquatic ecosystems and the emission of greenhouse gases that tity will fade with time. Synthetic fertilizers are crucial in this can accelerate global climate changes. Those climate changes H. Mansouri, H. Ait Said, A. Oukarroum, H. Ben youcef H. Mansouri, F. Perreault High Throughput Multidisciplinary Research Laboratory (HTMR) School of Sustainable Engineering and the Built Environment Mohammed VI Polytechnic University (UM6P) Arizona State University Benguerir 43150, Morocco Tempe, AZ 85287-3005, USA E-mail: [email protected] H. Noukrati High Institute of Biological and Paramedical Sciences (ISSB-P) Mohammed VI Polytechnic University (UM6P) Benguerir 43150, Morocco The ORCID identification number(s) for the author(s) of this article can be found under https://doi.org/10.1002/adsu.202300149 A. Oukarroum AgroBioSciences Plant Stress Physiology Laboratory (AgBS) © 2023 The Authors. Advanced Sustainable Systems published by Mohammed VI Polytechnic University (UM6P) Wiley-VCH GmbH. This is an open access article under the terms of the Benguerir 43150, Morocco Creative Commons Attribution License, which permits use, distribution and reproduction in any medium, provided the original work is properly F. Perreault cited. Department of Chemistry University of Quebec in Montreal DOI: 10.1002/adsu.202300149 CP 8888, Succ. Centre-Ville, Montreal, QC H3C 3P8, Canada Adv. Sustainable Syst. 2023, 7, 2300149 2300149 (1 of 19) © 2023 The Authors. Advanced Sustainable Systems published by Wiley-VCH GmbH 23667486, 2023, 9, Downloaded from https://onlinelibrary.wiley.com/doi/10.1002/adsu.202300149 by Cochrane Mexico, Wiley Online Library on [01/11/2024]. See the Terms and Conditions (https://onlinelibrary.wiley.com/terms-and-conditions) on Wiley Online Library for rules of use; OA articles are governed by the applicable Creative Commons License www.advancedsciencenews.com www.advsustainsys.com Figure 1. A,B) Loss of excess conventional fertilizers in soil. In (A) excess N fertilizer is lost in the environment through run-off, ammonia volatilization, leaching of nitrates, and nitrous oxide emissions. In (B) excess P fertilizer is either lost by run-off and leaching, immobilized into organic form, adsorbed into inorganic form, or precipitated into mineral form. in turn affect agriculture through a decrease in crops yield, a future research on next generation fertilizers that, by reducing reduction in their nutritional quality, or an increased frequency fertilizer use and increasing agricultural yield, can play an im- of extreme weather events detrimental to plants’ growth. The portant role in achieving several of the sustainable development development of new techniques to help increase fertilizers’ effi- goals defined by the European Union, such as reducing hunger ciency, both in terms of food production and environmental pro- and malnutrition, promoting responsible production and con- tection, is thus critical to achieve more sustainable agricultural sumption in the agricultural field, and decrease the greenhouse practices. gas emissions associated with fertilizer production and applica- The low NUE observed for plants in agricultural soils is in part tion. due to the nature of conventional fertilizers which, once applied, will release a large amount of nutrients in the early stage of plant 2. Controlled Release Fertilizers: Classification, growth, leaving too little at later stages. To overcome this issue, Synthesis, and Mechanisms of Release controlled release fertilizers (CRF) have been explored as an ap- proach to deliver fertilizers in a way that match the rate required The CRFs are specially designed products that release active fer- by the plant, which would avoid losses caused by leaching, evap- tilizing nutrients in a controlled, slow fashion in synchronization oration, and other factors related to the weather. However, to with the progressive demands of plants for nutrients. The ini- accurately control the release of such fertilizers, a number of chal- tial efforts in the development of CRFs started in the 1960s and lenges and factors that determine their applicability and perfor- were primarily focused on sulfur and polyethylene as coating ma- mance still need additional research and investigation. For exam- terials. Since then, many polymer materials and natural coating ple, there is a pressing need for more research into the processes agents were explored, along with a wide range of advanced mate- that can trigger and govern the release rate of nutrients by CRF, as rials such as multifunctional super-absorbents or nanocomposite well as the primary environmental factors (such as temperature, structures. In this section we will discuss the distinct categories moisture, microbes, acidity, and soil type) that influence them. of CRF, present their synthesis methods, and highlight the differ- In this review, we present the most recent research on the de- ent mechanisms for the release of nutrients. velopment of CRF in an effort to highlight the most promising approaches and materials that may be exploited to coat fertilizers 2.1. Classification of CRF and achieve controlled release. We will highlight the techniques and practices that can provide greater performance with less coat- The different categories of CRF, as described by Shaviv et al., ing material, as well as any potential difficulties or issues related are presented in Figure 2. These categories are: 1) low solubility with their application. We will also explore the promising field of organic-N compounds, which can be further classified as either stimuli-responsive hydrogels, which can better match nutrient biologically decomposing products like urea-formaldehyde (UF) release to the needs of the crops and identify the key areas that or chemically (mostly) degrading compounds like isobutyledene- still require research before they can be successfully applied in diurea, 2) low-solubility inorganic compounds, which are com- the agricultural sector. This paper will give recommendations for monly found in forms such as metal ammonium phosphates Adv. Sustainable Syst. 2023, 7, 2300149 2300149 (2 of 19) © 2023 The Authors. Advanced Sustainable Systems published by Wiley-VCH GmbH 23667486, 2023, 9, Downloaded from https://onlinelibrary.wiley.com/doi/10.1002/adsu.202300149 by Cochrane Mexico, Wiley Online Library on [01/11/2024]. See the Terms and Conditions (https://onlinelibrary.wiley.com/terms-and-conditions) on Wiley Online Library for rules of use; OA articles are governed by the applicable Creative Commons License www.advancedsciencenews.com www.advsustainsys.com point, most nutrients have been dissolved and released, which reduces the osmotic equilibrium and thus the release rate. More specifically, in the case of coated CRF, a multi-stage dif- fusion model was suggested. After spreading coated fertilizers in the soil, irrigation water penetrates the coating and condenses on the solid fertilizer core, causing the dissolution of nutrients. As osmotic pressure rises, the granule swells and exhibits two reactions. When osmotic pressure exceeds the resistance of vul- nerable coatings (e.g., sulfur or modified sulfur), the coating will burst and the core nutrient immediately liberated. On the other hand, if the layer resists the increasing pressure, the core fertil- izer is gradually released, driven by a concentration or pressure gradient, or a combination of both. The solubility and availabil- ity of the nutrient in the soil determine the speed at which fertil- Figure 2. Classification of CRF into low solubility organic and inorganic compounds, and compounds with a physical barrier to control the release izers will diffuse through the polymer layer and into the soil. The of the core nutrient (coatings and matrices). Text in bold to highlight the rate of dissolution of the nutrient is controlled by the concentra- only categories covered in this review. tion difference between the soil and the CRF’s core. Microor- ganisms can also play an important role in the fertilizer release (MgNH4 PO4 ) or partially acidulated phosphates rock, and 3) CRF process for several CRFs. Microorganisms in the soil can produce with a physical barrier that controls the release, which can be in enzymes or acids that degrade the polymeric coating layer, gradu- the form of a continuous matrix where the soluble active ingredi- ally releasing the nutrients into the soil. Therefore, the coating ent is spread, or nutrient granules surrounded by a hydrophobic layer must be strong enough to prevent the core from expanding polymer. because of absorbing water and be able to resist microbial degra- Compared to low-solubility macronutrient formulations, dation in order to maximize the longevity of the fertilizer granule which simply slows down the release kinetic to increase their inside the soil. retention in soils, using an external barrier to control the release also provides an opportunity to develop functional coatings that control the release within the specific bounds that match 3. Coated CRF: Definition, Coating Techniques, and the plant’s nutritional needs. Therefore, this review will be Cost-Effective Coating Practices focusing on CRF materials using a physical barrier, a matrix, or an external coating to control the release. Coated CRF can be Coated CRFs are conventional soluble fertilizer products that, af- divided into two subgroups: inorganic, mineral-based coatings ter granulation, prilling, or crystallization are provided a water- such as sulfur and organic coatings using polymers, thermoplas- insoluble covering layer to regulate moisture absorption and tics, or resins. These coatings can be either hydrophobic, such hence solubilization rate and nutrient release. Coating mate- as polyolefin or rubber, or gel-forming polymers, also known as rials may be broken down into two groups: inorganic materials hydrogels. The nature of the physical barrier determines how like sulfur, bentonite, or phosphogypsum; and organic polymers, nutrients will be released from the CRF and therefore dictates which can be either synthetic polymers such as polyurethane, its potential applications in the field. polyethylene, and alkyd resin or natural polymers, such as starch, chitosan, or cellulose. However, sulfur coated CRF can cause soil acidity if sulfur is 2.2. Mechanisms of Release of CRF converted into sulfuric acid after application. Moreover, while CRF created from natural materials decomposes rapidly in the Each CRF granule has a distinct three-stage sigmoidal and non- soil, CRF made from synthetic materials such as polyurethane linear release profile (Figure 3); a lag phase where there is no and polyethylene generate volatile organic compounds and have evidence of release, a stage of continuous release, and a decay a detrimental effect on the soil as a result. This kind of poly- phase in which the release rate declines considerably. In the first mer coatings can also break down into microplastics over its step, soil water, primarily as vapor, wets the coating, resulting lifetime, generating another contaminant of emerging concern in the formation of fissures as the water penetrates to the core due to their persistence. Therefore, although the use of CRF has and allows the fertilizer to dissolve. No fertilizer is discharged at many positive effects in agriculture, such as lower fertilizer use this stage due to the vapor pressure differential. The lag may be and decreased nutrient pollution, the choice of the material must caused by the time needed to fill the internal voids with the nec- be made carefully as to not impact negatively the soil quality over essary volume of water or by a steady state between water intake time. and solute exiting. In the following step, as water keeps entering, more material fertilizer is dissolved and the osmotic pressure in the core increases, allowing fertilizers to be gradually released 3.1. Coating Techniques through gaps in the polymer coating (Figure 4). Diffusion of nu- trients to the soil is maintained if the granule’s solution remains Polymer-coated CRF can be produced using several types of com- saturated. Once the osmotic pressure surpasses a set level, the mercially available coating processes. Examples of such tech- coating layer collapses and the fertilizer bursts out. In the decay niques are the rotating drum, pan coater, and fluidized bed coater Adv. Sustainable Syst. 2023, 7, 2300149 2300149 (3 of 19) © 2023 The Authors. Advanced Sustainable Systems published by Wiley-VCH GmbH 23667486, 2023, 9, Downloaded from https://onlinelibrary.wiley.com/doi/10.1002/adsu.202300149 by Cochrane Mexico, Wiley Online Library on [01/11/2024]. See the Terms and Conditions (https://onlinelibrary.wiley.com/terms-and-conditions) on Wiley Online Library for rules of use; OA articles are governed by the applicable Creative Commons License www.advancedsciencenews.com www.advsustainsys.com Figure 3. Schematic representation of the temporal development of soil nutrient availability, as supplied by CRF and conventional fertilizers. As a result of their burst release, the conventional fertilizers release most of the nutrients during the first growth stages of the plant. The CRF release follows a sigmoidal curve involving three phases: an initial lag period, followed by a linear release, and last, a decay release phase. (Table S1, Supporting Information). Rotary pan or drum coat- applicability, it is important to use coating materials that are easy ing is one of the most common techniques used to make com- to apply, inexpensive, and environmentally benign to ensure the mercial CRF due to its simplicity: the rotary pan technique in- broad use of coated CRF. volves rotating a pan or a drum containing fertilizer granules and spraying them with a polymer solution using nozzles, which will form a coating upon drying. Warm air can be blown over 3.2.1. Economic Benefits of Coated CRF the wet coatings to reduce the drying time. This simple process has been in use for producing CRF commercially; however, it is Coated CRF are designed to ensure that the late nutrient release known to have a low coating efficiency and, as a result, a lot of is matching in time with the nutrient demands of crops, mak- coating material is wasted to obtain a good film on the surface ing a single application able to meet the nutrient requirements of the granules. To reduce solution losses during the coating for the entire season. That way, the use of CRF can reduce spread- process, CRF can be produced using a fluidized bed technique. ing costs by allowing for an earlier application that avoids the “an- Since fluidization allows for precise regulation and adaptability, nual spring rush,” when access to the field is challenging. Like- it improves the efficiency of the operation. Spraying the coating wise, single basal application of CRF can lower the demand for material into a supporting air stream causes the coating material additional short-season top dressing applications during critical to create a fluidized bed. Once the fertilizer is added, the coat- periods, such as for rice paddies, which reduces labor expenses. ing substance covers the granule, resulting in a new compound Since financial benefit is the main driver for farmers when se- with gradual nutrient release. A schematic representation of lecting a management practice, Zhang et al. demonstrated that the main coating processes used for CRF production is shown in a single application of blending urea (BU) which is composed of Figure 5. both coated controlled-release urea and uncoated common urea saved half of the labor expenses, which balanced the higher fertil- izer N prices. Thus, the farmer’s financial earnings were not af- 3.2. Cost-Effective Coating Materials and Practices: Making fected by the implementation of BU and could potentially been Innovation Affordable Again in fact improved. Through a 2 years field trials using aromatic brown rice, Shivay et al., showed that 0.5% boron-coated urea, Industry is increasingly focusing on new ways to augment the 5.0% sulfur-coated urea, and 2.5% zinc-coated urea provide bet- productivity of agricultural systems while decreasing production ter yields than non-coated prilled urea. These treatments max- costs, which has led to the adoption of novel alternative materi- imized returns and benefit–cost ratios, as shown in the Figure 6. als in agriculture. Agricultural fertilizers are being used on a However, despite these proven benefits of CRF fertilizers, they massive scale, which provides a strong financial reason for inno- are not commonly used in Europe outside of gardening and for vative, cost-effective formulations to be developed. For large scale the fertilization of lawns and ornamental plants. Adv. Sustainable Syst. 2023, 7, 2300149 2300149 (4 of 19) © 2023 The Authors. Advanced Sustainable Systems published by Wiley-VCH GmbH 23667486, 2023, 9, Downloaded from https://onlinelibrary.wiley.com/doi/10.1002/adsu.202300149 by Cochrane Mexico, Wiley Online Library on [01/11/2024]. See the Terms and Conditions (https://onlinelibrary.wiley.com/terms-and-conditions) on Wiley Online Library for rules of use; OA articles are governed by the applicable Creative Commons License www.advancedsciencenews.com www.advsustainsys.com Figure 4. Schematic representation of the different processes through which CRF releases nutrients. A) The coating layer failed, owing to the osmotic pressure induced by water penetration exceeding its pressure resistance threshold, resulting in a burst release. B) Gradual release of nutrients from the core through the expanding pores caused by the water penetrating the coating, resulting in increased internal osmotic pressure. C) Water absorption by the hydrogel coating, which swells to slowly release the dissolved nutrients under osmotic pressure (deswelling). Figure 5. Schematic illustration of the two most common coating techniques. A) The rotary pan/drum; B) The fluidized bed process used to form polymeric coatings on granular fertilizers. The movement of the air current causes the grains to swirl and hover around. A nozzle is used to coat fertilizer granules by spraying coating material over them, producing coated CRFs. Adv. Sustainable Syst. 2023, 7, 2300149 2300149 (5 of 19) © 2023 The Authors. Advanced Sustainable Systems published by Wiley-VCH GmbH 23667486, 2023, 9, Downloaded from https://onlinelibrary.wiley.com/doi/10.1002/adsu.202300149 by Cochrane Mexico, Wiley Online Library on [01/11/2024]. See the Terms and Conditions (https://onlinelibrary.wiley.com/terms-and-conditions) on Wiley Online Library for rules of use; OA articles are governed by the applicable Creative Commons License www.advancedsciencenews.com www.advsustainsys.com Figure 6. Costs of coating prilled urea with borax, sulfur, and zinc, as well as an economic assessment of net return and benefit:cost ratio per fertilizer treatment for a 1 hectare aromatic rice crop (Shivay et al. 2019) *At the rate of 7% of fertilizer prices (USD ha−1 ). Prevailing prices of fertilizer materials during 2013–14: i) Borax (12% boron) at the rate of 2.0 USD kg−1 , ii) sulfur dust (90% S) at the rate of 0.50 USD kg−1 , iii) ZnSO4 ·7H2 O (20% Zn) at the rate of 0.50 USD kg−1 ; iv) ZnO (80% Zn) at the rate of 1.49 USD kg−1 , v) prilled urea at the rate of 0.087 USD kg−1 (Note: Prilled urea at the rate of 883 USD tonne−1 ; 24.97 USD for an application of 130 kg ha−1 ). 3.2.2. CRF Production Cost Factors This cost reduction with scale-up can also be expected for chemical coating techniques, which for CRF is mostly used to The higher production costs of CRF compared to conventional make hydrogel-based coatings for controlled release or stimuli- fertilizers is one of the most important factors that limit their responsive fertilizers. For chemical coatings, the use of organic widespread adoption. Because of the prices of coating materi- solvents during hydrogel synthesis can be an important aspect als and the inefficiency in the coating practices, the production of the synthesis’ costs. Reusability of the solvent should there- costs of fertilizers may be increased by 10 to 30 times when or- fore be an important selection criterion for hydrogel-based CRF. ganic polymer coatings are added. However, improvements in The polymerization step is a critical component for this, since for the CRF coating processes could help mitigate this higher pro- some hydrogel synthesis, like solution polymerization synthesis, duction cost. Production methods for CRF can be divided into the solvent cannot be recovered but, for other approaches such chemical and physical techniques with the latter being the most as inverse suspension polymerization, which relies on perform- used today because they are simpler and less expensive to op- ing the polymerization reaction in monomer droplets dispersed erate. However, physical coating methods use higher amount of in a non-miscible medium such as water or oil, the solvent can be raw coating material in order to obtain homogeneous coating lay- recovered and purified for reuse. The use of inexpensive, bio- ers on the fertilizer granules, which raises the overall production based raw materials (discussed in the next section) as well as in- cost. An important aspect of the cost efficiency of CRF coating novative green chemistry techniques to avoid organic solvents is therefore maximizing the use of coating material. Techniques are potential avenues of development to improve the affordability such as fluidized bed coating can help minimize solution loss of hydrogel-based CRF coatings. and make the physical coating method more affordable, but this approach usually has a high capital cost and is not ideal for gran- ules of increasing sizes. Production size also matters for the 3.2.3. Cost-Effective Coating Materials and Production Methods CRF costs. For the moment, CRF production is often realized in small batches (1000 to 5000 kg) with select raw granular mate- Fertilizers can be coated with a variety of materials to obtain con- rial sizes to ensure optimal coating quality, which increases the trolled release properties. The choice of coating material, along production costs. This cost is likely to be mitigated as CRF be- with the production method, will be determining factors to CRF comes more abundant in the global market and production vol- costs and eventual marketability. Moreover, using bio-based and umes increased. biodegradable coatings is becoming an increasing area of interest Adv. Sustainable Syst. 2023, 7, 2300149 2300149 (6 of 19) © 2023 The Authors. Advanced Sustainable Systems published by Wiley-VCH GmbH 23667486, 2023, 9, Downloaded from https://onlinelibrary.wiley.com/doi/10.1002/adsu.202300149 by Cochrane Mexico, Wiley Online Library on [01/11/2024]. See the Terms and Conditions (https://onlinelibrary.wiley.com/terms-and-conditions) on Wiley Online Library for rules of use; OA articles are governed by the applicable Creative Commons License www.advancedsciencenews.com www.advsustainsys.com Table 1. Overview of cost-effective coating materials and techniques. Coating Material Cost-effective technique Research findings Ref. Castor oil-based Water polishing Water polishing DAP grains lowered the angle of repose by 2.48–10.57% and the polyurethane specific surface area by 5.70–48.76%. The release rate of water polished coated DAP granules is 5.36 times slower. Castor oil-based Wax-based surface modification Wax surface modification lowered DAP particle surface area and repose angle. polyurethane Prior research used 9 wt% coating material to release P for 25 days, while wax-surface–modified coated DAP granules released P for 93.4 days using 4 wt% coating material. Cassava starch modified Heated pre-coat solution N release time from uncoated urea granules in water was 63.33 s, rising with with di-sodium pre-coat solution temperature from 50 to 80 °C, reaching 209 s at 80 °C. tetraborate Recycled polystyrene Large tablets size Same release rate as the commercial polymer coated urea (PCU) with 70–80% foam less coating material. Coating large urea tablets with recycled polystyrene foam is 7–8 times cheaper than commercial PCU. Recovered lignin from Lignin retrieval from Kraft and Acetylated lignin-coated urea granules released 36.3% for Kraft lignin and 45.3% industrial wastewater Sulfite black liquors for Sulfite lignin after 7 days, while commercial sulfur-coated urea released 59%. Acetylation of lignin using acetic acid and sodium metabisulfite Biochar Microwave irradiation Biochar-hydrogel–coated urea released 20.03% N after 30 days. 40% cost reduction at lab-scale compared to conventional polymerization. Vegetable Oil polymer Oil-based polyurethane films After 70 days, 80% of the fertilizer content is released when using an Oil–PCU coating film with an isocyanate index of 1.58 After 180 days, the degradation percentage of five polyurethane films with different isocyanate indices ranged from 10.23% to 29.63% for fertilizer production since, in addition to regulating nutrient The pulp and paper Industry are another large source of use- release, there is a need to cut down on the emission of potentially ful waste in the form of lignin. Behin and Sadeghi experimented hazardous chemicals during CRF production and use. Table 1 with modified lignin as a coating for urea fertilizer particles. Kraft provides an overview of novel potentially cost-effective materials and sulfite black liquors, as sources of sulfate and sulfite lignin, used for the synthesis of CRF coatings. were modified using an acetylation reaction to improve their hy- Residue materials from industries like agriculture are a drophobicity. Then, by coating urea granules with the acetylated promising source of low-cost materials to explore given the scale lignin, a release of 36.3% and 45.3% after 7 days for the Kraft of agricultural production. For example, biochar, as a bio-waste, and sulfite lignin, respectively, compared to a release of 59% for is an interesting candidate due to its low cost and widespread sulfur coated urea. These improved rates suggest that recov- availability. Chemical synthesis of biochar using microwave irra- ered lignin from industrial wastewater can be used to achieve diation was recently introduced as a rapid, high-efficiency, low- good performance in CRF. Waste polymers can also be recycled energy, and low-pollution bulk heating option to create biochar– into new coating materials for fertilizers. For example, Yang et al. hydrogel composites.[24,25] Microwave irradiation, unlike tradi- used recycled polystyrene foam, either as is or blended with wax tional polymerization synthesis, does not involve the use of or- or polyurethane, as a coating material for the coating of urea gran- ganic solvents or any other byproducts. Using urea coated with a ules. This approach was estimated to not only reduce the cost of biochar–hydrogel composite, only 20.03% of N was released af- urea coating compared to commercial polymer coated urea but ter 30 days. Another type of agricultural materials usable for CRF also provide a novel solution to the recycling of plastic products. synthesis are oils, as demonstrated by Feng et al., who prepared Beyond the nature of the coating material, coating costs can a fully vegetable oil–based polyols using epoxidized soybean oil be reduced by reducing the amount of coating needed for con- and oleic acid as raw materials. These polyols were then used to trolled release. A study by Tian et al. showed a surface modi- form polyurethane films for CRF coatings. Based on the iso- fication technique based on abrasive particles that reduced the cyanate index of the polyurethane, coating degradation varied be- micro/nanoscale surface morphology of urea particles and im- tween 10% and 30% after 180 days in soil. When used to coat urea proved the coating adherence and uniformity. By reducing the fertilizers, the resulting CRF took up to 70 days to release 80% surface roughness of the urea particles, the amount of coating of its cumulative capacity, showing that these oil–polyurethane material needed to produce coated urea CRF was reduced by up coating have good prospects for controlled nutrient release. In to 28.6% for an equal duration of release. Water polishing is addition to these good performances of CRFs derived from another way to improve to reduce the roughness of fertilizer parti- agricultural waste, the opportunity to valorize the waste back cles and therefore reduce the amount of coating material needed. into the agricultural cycle is interesting for a circular economy Lu et al. used water polishing to treat the surface of diammo- perspective. nium phosphate (DAP) granules, which decreased their specific Adv. Sustainable Syst. 2023, 7, 2300149 2300149 (7 of 19) © 2023 The Authors. Advanced Sustainable Systems published by Wiley-VCH GmbH 23667486, 2023, 9, Downloaded from https://onlinelibrary.wiley.com/doi/10.1002/adsu.202300149 by Cochrane Mexico, Wiley Online Library on [01/11/2024]. See the Terms and Conditions (https://onlinelibrary.wiley.com/terms-and-conditions) on Wiley Online Library for rules of use; OA articles are governed by the applicable Creative Commons License www.advancedsciencenews.com www.advsustainsys.com surface area by 5.70–48.76% (based on the water % used) and Cross-linking reactions using ionic interactions or complex coac- therefore reduced the amount of surface to cover with the coat- ervation are based on molecules or polyelectrolytes with oppos- ing material. When applied to maize crops, the fertilizer granules ing charges. Ionic cross-linking is achieved by the introduction of coated with a castor oil–based polyurethane after water polishing counterions to initiate an in situ gelation reaction, which will be had a release rate 5.36 times slower than non-polished coated influenced by factors such as ionic strength, pH, type of coun- granules. In a similar approach, using large fertilizer tablets terion, and functional charge density of the solution. Alginate also reduces the specific surface area of the fertilizer material, is a common example of an ionic cross-linked hydrogel when resulting in less coatings needed. Large urea tablet (12 mm in it is mixed with divalent cations. On the other hand, com- diameter and 8.5 mm thick) had the same release rate as a com- plex coacervation is the rapid aggregation of two polyelectrolytes mercial polymer coated urea particles (diameter of 0.5–2 mm) with opposing charges, resulting in a liquid–liquid phase sepa- but used 70% to 80% less coating material. Substantial sav- ration in an aqueous medium. Some examples of this type ings can therefore be gained simply by better understanding and of physical hydrogels are those made by coacervating chitosan optimizing the amount of coating needed for CRF fabrication. and xanthan. Polymers of the same charge can also be cross- linked through hydrogen bonding; for example, poly(acrylic acid) and poly(methacrylic acid) can make stable hydrogels in the pres- 4. Matrices CRF: Definition, and Loading ence of poly(ethylene glycol) by creating hydrogen bonds between Techniques the carboxyl group (─COOH) of acrylic acids and the oxygenated groups of poly(ethylene glycol). Hydrogen bonding for cross- Water-soluble fertilizers with physical barriers that regulate nu- linking is of particular interest for hydrogels that possess self- trient release take the form of a matrix of active fertilizer nutrients healing properties due to the reversible nature of the bonds as spread throughout a coating material that impedes fertilizer dis- the microenvironment of the polymer changes. solution. Both hydrophobic matrices, like polyolefin and rubber, Thermally crosslinked hydrogels form after cooling a heated and gel-forming hydrophilic matrices, commonly referred to as biopolymer solution. During cooling, an interchain connection hydrogels, can be used as a controlled release matrix. is made when two or more molecules align (whether in parallel or perpendicular to the axis of the chain length), which cross- 4.1. Hydrophilic Matrices: Hydrogels CRF links the biopolymer into a hydrogel. The polysaccharides agar and carrageenan are two common examples of thermally cross- Hydrogels are a form of cross-linked polymer with hydrophilic linked hydrogels. A similar process occurs during the crys- groups attached to the polymeric backbone that can absorb sig- tallization process generated by freeze–thaw cycles. During the nificant amounts of water without dissolving. By itself, this freeze–thaw process, the crystallization maximizes the density of high-water adsorption capacity is attractive for agriculture appli- polymer by decreasing the chain gap in the polymer, which al- cations, knowing that farmers in arid and desert places across lows the chains to align and crosslink with one another to create the world have limited access to water during the dry season but, a network structure. After going through a series of freeze–thaw at the same time, considerable precipitation is lost during this cycles, the hydrogel formed ends up with a porous morphology period. Therefore, beyond their use to control nutrient release, due to the voids left by the melting particles. Variations in poly- hydrogels may also decrease water losses due to evaporation and mer solution, frequency of freeze–thaw rounds, freezing interval, reduce irrigation periodicity. In the design of CRF, hydrogels and freezing degree all affect the freeze–thawed hydrogel’s me- are used for their high water absorption capacity, high crosslink- chanical characteristics. The cryogelation of xanthan is an ex- ing gel fraction, low toxicity, affordability, outstanding swelling ample of an hydrogel generated by freeze–thaw cycling. These and storage stability, and the ability to rewater after drying. thermal cross-linking benefits from not using any added chem- Moreover, the hydrogel is resistant to physical damage or other icals; however, the energy required for the temperature change stress that would damage most coatings and result in the unde- may be prohibitive unless heating is already required to make sirable burst release of nutrients. Research on using hydrogels the hydrogel solution. as CRFs is summarized in the Table 2 below. Chemical cross-linking relies on covalent interaction between polymer chains to create permanent chemical hydrogels. Dif- ferent chemical cross-linking techniques have been used in the 4.1.1. Cross-Linking Methods of Hydrogels literature, including functional groups crosslinking, free radi- cal polymerization, irradiation induced crosslinking, and enzyme Because hydrogels are usually derived from water soluble com- mediated crosslinking. For CRF synthesis, the primary meth- pounds, cross-linking the hydrogel matrix is essential to preserve ods used have been based on free radical polymerization synthe- its structure as a coating when used in the field. The addition sis and functional group cross-linking. Free radical polymeriza- of cross-links between polymer chains influences the material’s tion is based on the formation of a free radical site by an initia- physical characteristics, the nature of which is determined by the tor, which results in the accumulation of monomers in a chain- degree of cross-linking as well as the crystallinity of the poly- like pattern. Free radical polymerization can be triggered by mer. Several cross-linking techniques are available to be used for a variety of initiators, such as chemical redox reagents, ther- hydrogel formation, such as electrostatic, thermal, or chemical mal decomposition, electro- or sono-chemical activation, ioniza- cross-linking. tion radiation, or photopolymerization with visible or ultravio- Hydrogels can be cross-linked electrostatically through ionic let light. The type of initiator to be used depends on the types interactions, complex coacervation, or hydrogen bonding. of monomers to be cross-linked as well as the properties of the Adv. Sustainable Syst. 2023, 7, 2300149 2300149 (8 of 19) © 2023 The Authors. Advanced Sustainable Systems published by Wiley-VCH GmbH 23667486, 2023, 9, Downloaded from https://onlinelibrary.wiley.com/doi/10.1002/adsu.202300149 by Cochrane Mexico, Wiley Online Library on [01/11/2024]. See the Terms and Conditions (https://onlinelibrary.wiley.com/terms-and-conditions) on Wiley Online Library for rules of use; OA articles are governed by the applicable Creative Commons License www.advancedsciencenews.com www.advsustainsys.com Table 2. Overview of hydrogel-based CRF studies. Polymer Active Maximum Release time Plant Agronomic tests findings Ref. ingredient swelling capacity Gelatin Ammonium 218% 50 h in water Cucumber Hydrogels CRF increased seed nitrate germination from 70% to 100%, and (AN) enhanced stem elongation by 42%. Tomato Hydrogels CRF increased seed germination from 76% to 94%, and enhanced stem elongation by 41%. Wheat straw Urea 862 g g−1 16.3%, 60.2%, and 78.5% within – – 1, 5, and 10 days in soil corncob-g-poly(acrylic Urea 1156 g g−1 56.6% was released after 30 days Cotton Hydrogels CRF increased cotton seedlings acid)/bentonite in soil plant height, root length, fresh weight, and dry weight by 37.76, 33.48, 44.07, and 64.29%, respectively, compared to commercial urea. Hydrogels CRF increased cotton germination rate to 86.67% compared to commercial urea (66.67%). Hydroxy propyl methyl Urea 15.6 g g−1 87.01% release in soil in 44 days – – cellulose, PVA, glycerol and blended paper Double hydrogel network Urea 107.9 g g−1 45.6%, 73%, and 87.8% within – – of sodium alginate 0.5, 2, and 4 h in water. and polymerization of 39.8%, 68.7%, and 79.5% within 𝛽-cyclodextrin, acrylic 0.5, 2, and 4 days in soil. acid, and acrylamide in the presence of halloysite NaCMC and Zinc 635% for 50.4% and 20.5% on the third Wheatgrass Zinc-loaded NaCMC and CG hydrogels carrageenan (CG) CG and day, 71.4% and 38.7% on the increase wheatgrass plant height, 110% for fifth day, and 96.1% and 66.7% germination rate, fresh weight, and dry NaCMC on the eight day in soil for CG weight. and CMC hydrogels, respectively Acrylic acid Urea 909 g g−1 0.18, 0.37, 0.58, 0.81, 1.06, 1.59, Maize Low application rate of the hydrogel 2.67, and 3.71 wt % within 1, 3, (6, with en- properties of the hydrogel. The CNF also have moderate ther- hanced stability with increasing pH. Sodium carboxymethyl mal expansion, high crystallinity, and hydrophilicity, and an eas- cellulose (CMC), a water-soluble anionic cellulose derivative, is ily adjustable surface chemistry, all of which can be of interest also frequently used to form hydrogel, particularly in the form of for CRF synthesis. Shaghaleh et al. successfully synthesized gel beads. To obtain a pH-responsive hydrogel from CMC for a novel N pH-responsive/sustained release fertilizer composite the release of NPK, Olad et al. grafted sulfonated carboxymethyl based on wheat straw aminated-cellulose nanofibers and cationic cellulose with acrylic acid in the presence of polyvinypyrrolidone poly(acrylamide-co-2-aminoethyl methacrylate hydrochloride) by and silica nanoparticles. This hydrogel material was shown to direct AN fertilizer encapsulation. While the results revealed Adv. Sustainable Syst. 2023, 7, 2300149 2300149 (12 of 19) © 2023 The Authors. Advanced Sustainable Systems published by Wiley-VCH GmbH 23667486, 2023, 9, Downloaded from https://onlinelibrary.wiley.com/doi/10.1002/adsu.202300149 by Cochrane Mexico, Wiley Online Library on [01/11/2024]. See the Terms and Conditions (https://onlinelibrary.wiley.com/terms-and-conditions) on Wiley Online Library for rules of use; OA articles are governed by the applicable Creative Commons License www.advancedsciencenews.com www.advsustainsys.com that N release was lowest in a neutral pH medium, it was high- polymer can be made to vary in response to temperature and est in a lower pH condition, where N supply was maintained for release active ingredients. Hydrophilic and hydrophobic do- 90 days in a neutral-irrigated soil growing rice. In another study, mains coexist within the structure of thermosensitive polymers. CNFs were mixed with SA and PVA, and then crosslinked in the As a result of temperature fluctuations in the interaction among presence of NPK fertilizer. This SA/CNF/PVA composite re- both hydrophilic and hydrophobic portions in the polymer and tained more water in both deionized water and mixed soil, and water components, a sol–gel phase transition takes place in these exhibited a pH-sensitive swelling behavior, with a rising trend kinds of materials. The phase transitions of some polymers are in equilibrium swelling ability from 3 to 6, stability from 6 to 8, characterized by a lower critical solution temperature (LCST), and a reduction at greater pH levels from 8 to 11. These studies below which they are in a soluble and hydrated state but be- emphasized the potential of CNFs as a component for designing comes hydrophobic and precipitates above this temperature (sol– advanced functional hydrogel fertilizers. gel transition), while the phase transitions of certain other poly- Combining the hydrophilic properties of acrylic polymers with mers are characterized by an upper critical solution tempera- the biodegradable nature of starch-based composites can allow ture (UCST), where the opposite transformation actually hap- for CRF materials that will not become persistent in the soil. pens (gel-to-sol). Polymer networks in temperature-sensitive However, care must be taken when altering the crosslinking of hydrogels show swelling responses that can be either positive, starch-based hydrogels if biodegradability is the desirable prop- where the gel material swells as temperature rises, or negative, erty, as extensive crosslinking and substitutions in starches can where the gel material contracts as temperature rises. The reduce their biodegradability. Dudu et al. synthesized a novel LCST or UCST of the hydrogels may be adjusted by tuning the N,N-dimethylacrylamide—maleic acid (MA)—Starch (St)-based ratio of hydrophilic to hydrophobic segments. superabsorbent (DMSt1 ) and tested its abilities as a controlled Hydrogels having an interpenetrating polymer network release fertilizer in lettuce growing. Dudu et al. used HCl/NaOH (IPN) of polymer chains like polyacrylamide and PAA or to make negative and positive surface modifications of DMSt1 , poly(acrylamide-co-butyl methacrylate) crosslinked with N,N′- labeled DMSt2 and DMSt3 , respectively. The latter had the great- methlenebisacrylamide are among the few known instances est maximum swelling rate of 37.38% in deionized water envi- of UCST-type of polymers. As an example, examined an ronment. The anionic and cationic characteristics of the DMSt1 innovative thermo-sensitive semi-IPNs formed by incorporat- changed and the hydrogel displayed varied swelling behaviors at ing cellouronic acid sodium, which is derived from bagasse different pH levels after being treated with acid and base. The pith, into a poly(acrylamide-co-diallyldimethylammonium chlo- DMSt1 , DMSt2 , and DMSt3 -based hydrogels were also shown to ride) (poly(AM-co-DAC)) network. These semi-IPN gels dis- have excellent water uptake potential, with equilibrium swelling played UCST-type temperature sensitivity and excellent water- values of 7163.7% at pH 10, 15708% at pH 10, and 27838%, re- absorbency. The UCST could be adjusted to higher temperatures spectively, at pH 8. This water retention ability resulted, during by increasing the ratio of H-bonds to zwitterionic pairs and the pot experiments with lettuce plants, in a decrease in membrane total interchain connections between the two polymers. However, damage index compared to the control sample, showing that the when urea and other fertilizer salts (i.e., KH2 PO4 , NH4 NO3 , and hydrogels, in addition to nutrient release, can also prevent water MgSO4 ) were introduced to the solution, the UCST was found deprivation, in crops. to decrease, emphasizing the need to consider the release envi- Some possibilities for customizing nutrient release to the rhi- ronment’s chemistry when designing the phase transition tem- zosphere microenvironment can be found by comparing the pH peratures of CRF. The LCST thermosensitive hydrogels were window of stimulus response hydrogels to the pH gap between also explored by Feng et al., who used surface-initiated atom- bulk soil and plant’s rhizospheres. Several investigations have re- transfer radical polymerization to produce a “smart” fertilizer ported hydrogels that are stable at near-neutral pH (about 6 to from poly(N,N-dimethylaminoethyl methacrylate) (PDMAEMA) 8), but that expand more rapidly at lower pH levels.[71,73,79] These brushes grafted onto polydopamine-coated ammonium zinc pH ranges may coincide with the soil and rhizosphere conditions phosphate. They showed that temperatures below the LCST seen in some crops (as noted in Table 3), indicating the poten- can increase the rate of nutrient release whereas temperatures tial usefulness of adopting stimuli-responsive techniques. Some above the LCST decreased nutrient release. These findings sug- crops, however, have narrow pH windows between the bulk soil gested that fertilizer made with PDMAEMA brushes can be de- and the roots, which may necessitate additional optimization of signed to possess controlled–release capabilities. the hydrogel chemistry. In addition, a better understanding of Polyurethane is another polymer that can be engineered to the changes found in the rhizosphere needs to be provided be- manifest reversible changes in chemical and physical character- fore implementing such solutions for a specific crop, as the dif- istics upon exposure to temperature variations.[87–89] One exam- ferences between the bulk soil and the pH microenvironments of ple of polyurethane used as a thermo-responsive coating mate- the rhizosphere are likely to change with the season, soil chem- rial for CRF application is the study by Qiao et al., who em- istry, plant growth cycle, or physiological status. ployed polycaprolactone (PCL) with varying molecular weights (PCL500, PCL1000, PCL2000, and PCL3000) as raw materi- als to produce six types of polyurethane-based CRF coatings 4.2.2. Thermo-Responsive Hydrogels by reacting polyether polyol (PPG)/PCL blends with methy- lene diphenyl diisocyanate (MDI). Results demonstrated that Thermo-sensitive carrier systems have a lot of potential in agri- PPG/PCL2000-based PUCF blends can copolymerize with MDI culture since the temperature of the environment is always to generate a block copolymer with a two-phase structure (crys- changing. The physicochemical characteristics of the hydrogel talline/amorphous). The CRFs coated with the block copolymer Adv. Sustainable Syst. 2023, 7, 2300149 2300149 (13 of 19) © 2023 The Authors. Advanced Sustainable Systems published by Wiley-VCH GmbH 23667486, 2023, 9, Downloaded from https://onlinelibrary.wiley.com/doi/10.1002/adsu.202300149 by Cochrane Mexico, Wiley Online Library on [01/11/2024]. See the Terms and Conditions (https://onlinelibrary.wiley.com/terms-and-conditions) on Wiley Online Library for rules of use; OA articles are governed by the applicable Creative Commons License www.advancedsciencenews.com www.advsustainsys.com of PPG/PCL2000 at a molar ratio of 8 to 2 showed a sustained N Products that can include two or more sensitive groups release time of more than 30 days. The N release rate increased (temperature and pH, redox and enzyme, enzyme and pH, by 22.3 times per hour as the temperature rose from 32 to 33 °C, etc.) are called multi-responsive carriers. Using salicylic revealing a clear thermo-responsive behavior. These findings acid as a standard agrochemical, Hou et al. developed redox- emphasize the potential of using polyurethane to control nutrient and enzyme-responsive macrospheres by self-assembling 𝛽- release based on the temperature environment, warranting fur- cyclodextrin–modified zeolite and ferrocenecarboxylic acid– ther investigation, alongside rigorous plant growth assessments, grafted carboxymethyl cellulose. Salicylic acid liberation from to establish its benefit as a CRF coating material. macrospheres was increased in the presence of hydrogen per- For temperature-responsive hydrogels, targeting the seasonal oxide (oxidant) and cellulase (enzyme), with comparable release shifts in soil temperature may be the main interest for the de- rates of 85.2% and 80.4%, respectively, after 12 h, compared to sign of CRFs. Indeed, seasonal shifts in temperature are observ- the control, non-responsive sample (12.6% salicylic acid release). able in many parts of the world. The soil temperature shift will These results suggest that dual-responsive macrospheres may be also be depth dependent, being most pronounced in the soil’s up- a useful carrier for the controlled release of agrochemicals. per layers. By adding CRF to the soil in the off-season, and wait- Table 4 provides an overview of the different materials ing for them to become active, as desired, nutrient release can be and approaches for the design of stimuli-responsive CRF or timed to coincide with a certain growing season. As a result, this agrochemical-delivery systems. As discussed above, pH- and stimuli-responsive CRF could be employed to cut down on costs temperature-responsive hydrogels are the most actively explored associated with human labor during peak, high-demand periods for fertilizer delivery, due to their clear relationship with soil of the growing season. For other stimuli-responsive CRFs, such chemistry and its alteration by the plant’s metabolic activity. Com- as those made from pH-responsive materials, it is necessary to bining these types of CRFs with other functionalities to make have a firm grasp on how soil properties evolve on a seasonal ba- them responsive to multiple stimuli at the same time may offer sis in a given region. an opportunity to further refine the nutrient delivery and there- fore avoid competition with the soil components in fertilizer use. This is likely to result to an increase in production cost for these 4.2.3. Other Stimuli-Responsive Hydrogels more advanced stimuli-responsive CRFs, as their design become more refined and precise. However, considering that several of Many chemical and biological processes in cells rely on en- the materials proposed for the fabrication of stimuli-responsive zymes, which are crucial biological catalysts. In terms of sub- CRFs are natural materials such as cellulose, chitosan, or alginate strate specificity and selectivity, enzyme-catalyzed reactions are (see Table 4), there is a possibility to advance this next-generation unmatched. Making enzyme-responsive polymer complexes of CRFs in a way that mitigate the costs and environmental im- by covalently linking enzymatic substrates to amphiphilic copoly- pacts. mers is a typical approach. Since insects and their larvae have and release enzymes, most of enzyme-responsive hydrogels are being applied for the targeted delivery of insecticides. For 5. Conclusion and Future Outlooks example, created a new enzyme-responsive emamectin ben- zoate microcapsule by cross-linking silica with carboxymethyl Implementing CRF has a lot of potential as a strategy to improve cellulose using epichlorohydrin. The silica–epichlorohydrin– NUE in agriculture. However, due to their greater production carboxymethylcellulose microcapsules demonstrated outstand- costs, their broad application is still limited. The choice of coat- ing cellulase stimuli-responsive characteristics, maintained in- ing materials and methodology for a cost-effective CRF produc- secticidal activity against Myzus percae while protecting the ac- tion remains to be defined; however significant progress in the tive ingredient, epichlorohydrin, against light- and temperature- synthesis of novel CRF materials based on improved coating pro- induced degradation. However, as of yet, enzyme responsive hy- tocols or using low-cost, renewable coating materials have high- drogels have not been investigated for nutrients delivery in soils. lighted several promising avenues to improve the performance of It has been shown that redox-responsive hydrogels can alter CRF and reduce their production costs. Furthermore, innovation their color, fluorescence reactivity, or chiral structure in response in this niche sector of CRF can also be attained by stimuli respon- to external redox reactions or electric field stimulus by shifting sive CRF, which not only enables passive controlled nutrient re- the self-assembly phase of the gel component. To regulate lease via diffusion but also regulates this process to only occur in the release of agrochemicals and capture heavy metal ions at response to certain environmental stimuli. This strategy allows the same time, Hou et al. developed a redox-responsive hydro- for greater control of fertilizer release to meet crop uptake. Re- gel where cystamine was used as a crosslinker in a CMC matrix search in this area has begun to emerge for pH- and temperature- to produce a flexible material. A decrease in agrochemical losses responsive CRF; however, other stimuli more closely related to following application to crops was attributed to the 3D network the plant’s activity, such as enzyme-responsive hydrogels, have generated by CMC during the crosslinking process. Toxic metal not been explored yet. Finally, while CRF are generating a lot of leaching was decreased when redox-responsive hydrogels were interest on their ability to reduce fertilizer use, improve nutrient applied to soils contaminated with Cu2+ and Hg2+ by complex- use by the plants, and reduce environmental impacts, more in- ing the metal ions through their thiol groups. Agrochemicals in- depth financial and cost-benefit analyses are still needed to really corporated in hydrogels were delivered when the responsive net- identify the type of crops or soil environments where the switch works were decomposed by the reducer. from conventional fertilizers to CRF would be beneficial. Adv. Sustainable Syst. 2023, 7, 2300149 2300149 (14 of 19) © 2023 The Authors. Advanced Sustainable Systems published by Wiley-VCH GmbH Table 4. Summary of different stimuli responsive fertilizer or agrochemical delivery systems. Polymer Synthesis method Type of stimuli Active ingredient Research finding Ref. Wheat straw aminated-cellulose nanofibers In situ radical pH-responsive N fertilizer Low N release in neutral pH. copolymerization High N release in low pH (release maintained for 90 days). Cellulose nanofibers and sodium alginate Ionic crosslinking pH-responsive NPK Increased swelling ratio at pH from 3 to 6. Stable swelling ratio at pH from 6 to 8 Adv. Sustainable Syst. 2023, 7, 2300149 Decreased swelling ratio at pH from 8 to 11. www.advancedsciencenews.com Sodium alginate In situ free-radical graft pH-responsive NPK Increased swelling ratio at pH from 2 to 6. copolymerization Stable swelling ratio at pH from 5 to 7. Decreased swelling ratio at pH above 7. Chitosan In situ hydrogelation pH-responsive Urea At pH above 6, the hydrogel maintained its structural integrity for up to 15 days. Increased stability with increasing pH. Dimethylacrylamide/maleic acid/starch Redox polymerization pH-responsive Urea Positive surface modification of DMSt1 had the greatest swelling ratio (27838% at pH 8). 2300149 (15 of 19) Sulfonated carboxymethyl cellulose Graft copolymerization pH-responsive NPK Hydrogels swell at pH = 8 while shrinking at pH = 2 Hydrogels swelled in denatured water and shrank in 0.1 m NaCl. Poly(acrylamide-co-diallyldimethylammonium Free radical Thermo-responsive Urea, KH2 PO4 , NH4 NO3 , From 0.005 to 0.05 mol L−1 , the UCST decreased from 20 to 15 chloride copolymerization (UCST) MgSO4 °C in salt media. Poly(N,N-dimethylaminoethyl methacrylate) Atom-transfer radical Thermo-responsive Polydopamine-coated At 25 °C, pH 4.0, 7.0, and 10.0 solutions release P at 59.22%, polymerization (LCST) ammonium zinc 47.36%, and 40.89%, compared to 68.98%, 56.26%, and phosphate 34.21% at 40 °C. At 25 °C, pH 4.0, 7.0, and 10.0 solutions release Zn at 57.07%, 46.84%, and 38.54%, compared to 70.36%, 55.13%, and 31.67% at 40 °C. At 25 °C, pH 4.0, 7.0, and 10.0 solutions release NH4 + at 63.85%, 54.13%, and 48.79%, compared to 75.71%, 64.34%, and 42.36% at 40 °C. polyether polyol/polycaprolactone One-step copolymerization Thermo-responsive N fertilizer N release duration >30 days. polyurethane The N release rate increased by 22.3 times per hour when temperature increased from 32 to 33 °C Carboxymethyl cellulose – Enzyme-responsive Epichlorohydrin Cellulase stimuli-responsive. Insecticidal activity against Myzus percae. Carboxymethyl cellulose – Redox-responsive Naphthylacetic acid, Redox-release of agrochemical triggered by reducer. 6-benzyladenine Heavy metal ions (Cu2+ and Hg2+ ) capture Carboxymethyl cellulose Graft copolymerization Enzyme- and Salicylic acid Redox-responsive release rate of 85.2% triggered by hydrogen redox-responsive peroxide. Enzyme-responsive release rate of 80.4% triggered by cellulase www.advsustainsys.com © 2023 The Authors. Advanced Sustainable Systems published by Wiley-VCH GmbH 23667486, 2023, 9, Downloaded from https://onlinelibrary.wiley.com/doi/10.1002/adsu.202300149 by Cochrane Mexico, Wiley Online Library on [01/11/2024]. See the Terms and Conditions (https://onlinelibrary.wiley.com/terms-and-conditions) on Wiley Online Library for rules of use; OA articles are governed by the applicable Creative Commons License 23667486, 2023, 9, Downloaded from https://onlinelibrary.wiley.com/doi/10.1002/adsu.202300149 by Cochrane Mexico, Wiley Online Library on [01/11/2024]. See the Terms and Conditions (https://onlinelibrary.wiley.com/terms-and-conditions) on Wiley Online Library for rules of use; OA articles are governed by the applicable Creative Commons License www.advancedsciencenews.com www.advsustainsys.com Supporting Information M. Wesolowska, J. Rymarczyk, R. Góra, P. Baranowski, C. Slawinski, M. Klimczyk, G. Supryn, L. Schimmelpfennig, Int. Agrophys. 2021, 35, Supporting Information is available from the Wiley Online Library or from 11. the author. B. R. Dos Santos, F. B. Bacalhau, T. D. S. Pereira, C. F. Souza, R. Faez, Carbohydr. Polym. 2015, 127, 340. L. Zhang, Z. Liang, Y. Hu, U. Schmidhalter, W. Zhang, S. Ruan, X. Acknowledgements Chen, J. Cleaner Prod. 2021, 287, 125572. Y. S. Shivay, V. Pooniya, M. Pal, P. C. Ghasal, R. Bana, S. L. Jat, Global This work was supported by OCP Group and the University of Mohamed Challenges 2019, 3, 1900013. VI Polytechnique (UM6P) through Specific Agreement number 2. Y. Liu, J. Wang, H. Chen, D. Cheng, Sci. Total Environ. 2022, 846, 157303. D. Skrzypczak, K. Mikula, G. Izydorczyk, R. Taf, A. Gersz, A. Witek- Krowiak, K. Chojnacka, in Smart Agrochemicals for Sustainable Agri- Conflict of Interest culture (Eds: K. Chojnacka, A. Saeid), Elsevier, New York 2022, pp. The authors declare no conflict of interest. 81–102. Y. Wu, C. Brickler, S. Li, G. Chen, Polym. Test. 2021, 93, 106996. P. Wen, Z. Wu, Y. Han, G. Cravotto, J. Wang, B. C. Ye, ACS Sustainable Chem. Eng. 2017, 5, 7374. Keywords G. D. Feng, Y. Ma, M. Zhang, P. Y. Jia, L. H. Hu, C. G. Liu, Y. H. Zhou, Prog. Org. Coat. 2019, 133, 267. agriculture, controlled release fertilizers, cost-effective coatings, stimuli- J. Behin, N. Sadeghi, Int. J. Recyl. Org. Waste Agric. 2016, 5, 289. responsive hydrogels H. Tian, L. Zhang, J. Dong, L. Wu, F. Fang, Y. Wang, H. Li, C. Xie, W. Li, Z. Wei, Z. Liu, M. Zhang, J. Cleaner Prod. 2022, 369, 133331. Received: April 11, 2023 H. Lu, H. Tian, M. Zhang, Z. Liu, Q. Chen, R. Guan, H. Wang, Sci. Revised: June 3, 2023 Rep. 2020, 10, 5763. Published online: July 9, 2023 Y. C. Yang, M. Zhang, Y. Li, X. H. Fan, Y. Q. Geng, J. Agric. Food Chem. 2012, 60, 11229. H. Lu, H. Tian, Z. Liu, M. Zhang, C. Zhao, Y. Guo, R. Guan, Q. Chen, X. Yu, H. Wang, L. Zheng, ACS Omega 2019, 4, 20402. S. Iqbal, U. Riaz, G. Murtaza, M. Jamil, M. Ahmed, A. Hussain, Z. M. Y. Naz, S. A. Sulaiman, B. Ariwahjoedi, K. Z. K. Shaari, Surf. Eng. Abbas, in Microbiota and Biofertilizers, Springer, New York 2021, pp. 2015, 31, 486. 1–15. E. M. Ahmed, J. Adv. Res. 2015, 6, 105. H. Savoy, PB1637 Fertilizers and Their Use, The University of Ten- M. Guo, M. Liu, F. Zhan, L. Wu, Ind. Eng. Chem. Res. 2005, 44, 4206. nessee Agricultural Extension Service, Knoxville, TN 1999. D. Davidson, F. X. Gu, J. Agric. Food Chem. 2012, 60, 870. M. A. Iqbal, Nano-Fertilizers for Sustainable Crop Production under R. Michalik, I. Wandzik, Polymers 2020, 12, 2425. Changing Climate: A Global Perspective, IntechOpen, London 2019. D. W. Davidson, M. S. Verma, F. X. Gu, SpringerPlus 2013, 2, M. E. Trenkel, Slow- and Controlled-Release and Stabilized Fertilizers: An 318. Option for Enhancing Nutrient Use Efficiency in AgricultureControlled- M. Pulat, N. Yoltay, presented at ICRES 2018: International Conference release and Stabilized Fertilizers in Agriculture, 2nd ed., International on Research in Education and Science, Marmaris, Turkey, April 2018. Fertilizer Industry Association (IFA), Paris 2010. R. Liang, H. Yuan, G. Xi, Q. Zhou, Carbohydr. Polym. 2009, 77, 181. J. G. Arbuckle, L. W. Morton, J. Hobbs, Environ. Behav. 2015, 47, 205. P. Wen, Y. Han, Z. Wu, Y. He, B. C. Ye, J. Wang, Arabian J. Chem. 2017, I. Iavicoli, V. Leso, D. H. Beezhold, A. A. Shvedova, Toxicol. Appl. Phar- 10, 922. macol. 2017, 329, 96. S. A. Kareem, I. Dere, D. T. Gungula, F. P. Andrew, A. M. Saddiq, E. F. M. E. Trenkel, Controlled-release and Stabilized Fertilizers in Agriculture, Adebayo, V. T. Tame, H. M. Kefas, J. Joseph, D. O. Patrick, Gels 2021, International Fertilizer Industry Association, Paris 1997. 7, 262. A. Shaviv, Adv. Agron. 2001, 71, 1. Y. Shen, H. Wang, W. Li, Z. Liu, Y. Liu, H. Wei, J. Li, Int. J. Biol. Macro- B. Azeem, K. Kushaari, Z. B. Man, A. Basit, T. H. Thanh, J. Controlled mol. 2020, 164, 557. Release 2014, 181, 11. G. O. Akalin, M. Pulat, J. Polym. Res. 2020, 27, 6. D. Lawrencia, S. K. Wong, D. Y. S. Low, B. H. Goh, J. K. Goh, U. R. D. Cheng, Y. Liu, G. Yang, A. Zhang, J. Agric. Food Chem. 2018, 66, Ruktanonchai, A. Soottitantawat, L. H. Lee, S. Y. Tang, Plants 2021, 5762. 10, 238. M. Mahinroosta, Z. Jomeh, A. Allahverdi, Z. Shakoori, Mater. Today A. Shaviv, S. Raban, E. Zaidel, Environ. Sci. Technol. 2003, 37, 2257. Chem. 2018, 8, 42. I. Ganetri, Y. Essamlali, O. Amadine, K. Danoun, S. Aboulhrouz, M. P. Vinchhi, S. U. Rawal, M. M. Patel, in Drug Delivery Devices and Ther- Zahouily, Controlling Factors of Slow or Controlled-Release Fertilizers, apeutic Systems (Ed: E. Chappel), Elsevier, New York 2021, pp. 395– Elsevier, New York 2021. 419. T. Jahns, H. Kaltwasser, J. Polym. Environ. 2000, 8, 11. O. Jeon, D. W. Wolfson, E. Alsberg, Adv. Mater. 2015, 27, 2216. V. R. Remya, J. S. George, S. Thomas, in Controlled Release Fertilizers W. Hennink, C. van Nostrum, Adv. Drug Delivery Rev. 2002, 54, 13. for Sustainable Agriculture (Eds: F. B. Lewu, T. Volova, S. Thomas, K. W. Zhao, Q. Wei, B. Guo, Synthesis of Functional Hydrogels, Frontiers R. Rakhimol), Elsevier, New York 2021, pp. 183–194. Media, Lausanne, Switzerland 2020. B. Wei, J. Jiang, C. Gao, L. Zhang, Y. Zhan, S. Jiang, Y. Li, S. Sun, J. Xie, M. Borgogna, E. Marsich, I. Donati, S. Paoletti, A. Travan, Hydrogels, X. Fan, Ind. Crops Prod. 2021, 173, 114096. Jenny Stanford Publishing, Singapore 2016. U. Surendran, M. Jayakumar, P. Raja, G. Gopinath, P. V. Chellam, M. Akter, M. Bhattacharjee, A. K. Dhar, F. B. A. Rahman, S. Haque, T. Chemosphere 2023, 318, 137946. U. Ur Rashid, S. M. F. Kabir, Gels 2021, 7, 30. B. Beig, M. B. K. Niazi, Z. Jahan, A. Hussain, M. H. Zia, M. T. Mehran, P. Giannouli, E. R. Morris, Food Hydrocolloids 2003, 17, 495. J. Plant Nutr. 2020, 43, 1510. R. Parhi, Ulum-i Daroei 2017, 7, 515. Adv. Sustainable Syst. 2023, 7, 2300149 2300149 (16 of 19) © 2023 The Authors. Advanced Sustainable Systems published by Wiley-VCH GmbH 23667486, 2023, 9, Downloaded from https://onlinelibrary.wiley.com/doi/10.1002/adsu.202300149 by Cochrane Mexico, Wiley Online Library on [01/11/2024]. See the Terms and Conditions (https://onlinelibrary.wiley.com/terms-and-conditions) on Wiley Online Library for rules of use; OA articles are governed by the applicable Creative Commons License www.advancedsciencenews.com www.advsustainsys.com J. Maitra, V. K. Shukla, Am. J. Polym. Sci. 2014, 4, 25. A. Singh, D. J. Sarkar, S. Mittal, R. Dhaka, P. Maiti, A. Singh, T. Raghav, K. Pal, A. T. Paulson, D. Rousseau, in Modern Biopolymer Science (Eds: D. Solanki, N. Ahmed, S. B. Singh, J. Appl. Polym. Sci. 2019, 136, S. Kasapis, I. T. Norton, J. B. Ubbink), Elsevier, New York 2009, pp. 47332. 519–557. Q. F. Guan, H. B. Yang, Z. M. Han, Z. C. Ling, C. H. Yin, K. P. Yang, Y. D. K. Ho, D. T. Nguyen, T. Thambi, D. S. Lee, D. P. Huynh, J. Polym. X. Zhao, S. H. Yu, ACS Nano 2021, 15, 7889. Res. 2019, 26, 7. H. Shaghaleh, Y. A. Hamoud, X. Xu, S. Wang, H. Liu, J. Cleaner Prod. M. Bustamante-Torres, D. Romero-Fierro, B. Arcentales-Vera, K. 2022, 368, 133098. Palomino, H. Magaña, E. Bucio, Gels 2021, 7, 182. S. Liu, Q. Wu, X. Sun, Y. Yue, B. Tubana, R. Yang, H. N. Cheng, Int. J. R. A. Ramli, Polym. Chem. 2019, 10, 6073. Biol. Macromol. 2021, 172, 330. M. Teodorescu, A. Lungu, P. O. Stanescu, Ind. Eng. Chem. Res. 2009, C. Elvira, J. F. Mano, J. San Román, R. L. Reis, Biomaterials 2002, 23, 48, 6527. 1955. A. P. Blum, J. K. Kammeyer, A. M. Rush, C. E. Callmann, M. E. Hahn, J. Bendoraitiene, E. Lekniute-Kyzike, R. Rutkaite, Int. J. Biol. Macromol. N. C. Gianneschi, J. Am. Chem. Soc. 2015, 137, 2140. 2018, 119, 345. M. C. Camara, E. V. R. Campos, R. A. Monteiro, A. D. E. S. Pereira, P. T. E. Dudu, D. Alpaslan, N. Aktas, J. Polym. Res. 2022, 29, 524. L. De Freitas Proença, L. F. Fraceto, J. Nanobiotechnol. 2019, 17, 100. S. Chatterjee, P. C.-l. Hui, in Hydrogels - Smart Materials for Biomed- C. W. Purnomo, H. Saputra, in Controlled Release Fertilizers for Sus- ical Applications (Eds: L. Popa, M. V. Ghica, C.-E. Dinu-Pîrvu), Inte- tainable Agriculture, Elsevier, New York 2021, pp. 95–110. chOpen, London 2019. G. V. Lowry, A. Avellan, L. M. Gilbertson, Nat. Nanotechnol. 2019, 14, H. Katono, A. Maruyama, K. Sanui, N. Ogata, T. Okano, Y. Sakurai, J. 517. Controlled Release 1991, 16, 215. P. Hinsinger, C. Plassard, C. Tang, B. Jaillard, Plant Soil 2003, 248, 43. H. Zhang, X. Gao, K. Chen, H