The Dynamics of Disease Transmission PDF
Document Details
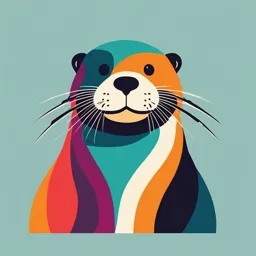
Uploaded by VirtuousXenon
Liceo de Cagayan University
Tags
Summary
This document details the transmission of human disease. It explores direct and indirect transmission methods, and the role of factors such as the environment, host, and agent in disease development. It also discusses different types of diseases, including both infectious and noninfectious conditions by discussing the interaction of genetic, behavioral, and environmental factors.
Full Transcript
The Dynamics of Disease Transmission Human disease does not arise in a vacuum. It results from an interaction of the host (a person), the agent (e.g., a bacterium), and the environment (eg., polluted air). Although some diseases are largely genetic in origin, virtually all disease results from an i...
The Dynamics of Disease Transmission Human disease does not arise in a vacuum. It results from an interaction of the host (a person), the agent (e.g., a bacterium), and the environment (eg., polluted air). Although some diseases are largely genetic in origin, virtually all disease results from an interaction of genetic, behavioral, and environmental factors, with the proportions differing for different diseases. Many of the underlying principles governing the transmission of disease are most clearly demonstrated using communicable diseases as a model. Hence this chapter primarily uses such diseases as examples in reviewing these principles. However, the concepts discussed are also applicable to diseases that are not infectious in origin (e.g., second-hand smoke causing cancer). Disease has been classically described as the result of the epidemiologic triad shown in Fig. 2.1. According to this diagram, it is the product of an interaction of the human host, an infectious or other type of agent, and the environment that promotes the exposure. A 20 vector, such as the mosquito or the deer tick, may be involved. For such an interaction to take place, the host must be susceptible. Human susceptibility is determined by a variety of factors including genetic background and behavioral, nutritional, and immunologic characteristics. The immune status of an individual is determined by many factors including prior experience both with natural infection and with immunization. The factors that can cause human disease include biologic, physical, and chemical factors as well as other types, such as stress or behavioral risks, which may be harder to classify (Table 2.1). Modes of Transmission Diseases can be transmitted directly or indirectly. For example, a disease can be transmitted from person to person (direct transmission) by means of direct contact (as in the case of sexually transmitted infec-tions). Indirect transmission can occur through a common vehicle such as a contaminated air or water supply or by a vector such as the mosquito. Some of the modes of transmission are shown in Box 2.1. Fig. 2.2 is a classic photograph showing droplet dispersal after a sneeze. It vividly demonstrates the potential for an individual to infect a large number of people in a brief period of time. As Mims has pointed out: An infected individual can transmit influenza or the common cold to a score of others in the course of an innocent hour in a crowded room. A venereal infection also must spread progressively from person to person if it is to maintain itself in nature, but it would be a formidable task to transmit venereal infection on such a scale.? Thus different organisms spread in different ways, and the potential of a given organism for spreading and producing outbreaks depends on the characteristics of the organism, such as its rate of growth, the route by which it is transmitted from one person to another, and the number of susceptible persons in the community. Fig. 2.3 is a schematic diagram of human body surfaces as sites of microbial infection and shedding. The alimentary tract can be considered as an open tube that crosses the body, and the respiratory and urogenital systems are shown as blind pockets. Each offers an opportunity for infection. The skin is another important portal of entry for infectious agents, primarily through scratches, bites, or injury. Agents that often enter through the skin include streptococci or staphylococci and fungi such as tinea (ringworm). Two points should be made in this regard: First, the skin is not the exclusive portal of entry for many of these agents, and second, infections can be acquired through more than one route. The same routes also serve as points of entry for noninfectious disease-causing agents. For example, environmental toxins can be ingested, inspired during respiration, or absorbed directly through the skin. The clinical and epidemiologic characteristics of many infectious and noninfectious conditions often relate to the site of the exposure to an organism or to an environmental substance and to its portal of entry into the body: Clinical and Subclinical Disease It is important to recognize the broad spectrum of disease severity. Fig. 2.4 shows the iceberg concept of disease. Just as most of an iceberg is under water and hidden from view with only its tip visible, so it is with disease: only clinical illness is readily apparent (as seen under Host Response on the right of Fig, 2.4). However, infections without clinical illness are important, particularly in the web of disease transmission, although they are not clinically apparent. In Fig. 2.4, the corresponding biologic stages of pathogenesis (biologic mechanisms) and disease at the cellular level are seen on the left. The iceberg concept is important because it is not sufficient to count only the clinically apparent cases we see; for example, most cases of polio in prevac-cine days were subclinical—that is, many people who contracted polio infection were not clinically ill. Nevertheless, they were still capable of spreading the virus to others. As a result, we cannot understand and explain the spread of polio unless the pool of inapparent cases (subclinical) is recognized. From the viewpoint of inapparent disease, this situation is not any different in many noncommunicable diseases. Although these diseases are not spread from person to person, many individuals, for example, can live a long time with inapparent chronic kidney disease, and it is only when they experience a clinical complication that a diagnosis of chronic kidney disease is made. Fig. 2.5 shows the spectrum of severity for several diseases. Most cases of tuberculosis, for example, are inapparent. However, because inapparent cases can transmit the disease, such cases must be identified and treated to control the further spread of the disease. In measles, many cases are of moderate severity and only a few are inapparent. At the other extreme, without intervention, rabies has no inapparent cases, and most untreated cases are fatal. Thus we have a spectrum of severity patterns that vary with the disease. Severity appears to be related to the virulence of the organism (how efficient the organism is at producing disease) and to the site in the body where the organism multiplies. All of these factors, as well as such host characteristics as the immune response, must be appreci- ated to understand how disease spreads from one individual to another. As clinical and biologic knowledge has increased over the years, so has our ability to distinguish different stages of disease. These include clinical and nonclinical disease. CLINICAL DISEASE Clinical disease is characterized by signs and symptoms. NONCLINICAL (INAPPARENT) DISEASE Nonclinical disease may include the following: 1. Preclinical disease: Disease that is not yet clinically apparent but is destined to progress to clinical disease. 2. Subclinical disease: Disease that is not clinically apparent and is not destined to become clinically apparent. This type of disease is often diagnosed by serologic (antibody) response or culture of the organism. 3. Persistent (chronic) disease: A person fails to "shake off the infection, and it persists for years, at times for life. In recent years, an interesting phenomenon has been the manifestation of symptoms many years after an infection was thought to have been resolved. Some adults who recovered from poliomyelitis in childhood report severe chronic fatigue and weakness; this has been called postpolio syndrome in adult life. These have thus become cases of clinical disease, albeit somewhat different from the initial illness. 4. Latent disease: An infection with no active multiplication of the agent, as when viral nucleic acid is incorporated into the nucleus of a cell as a provirus. In contrast to persistent infection, only the genetic message is present in the host, not the viable organism Carrier Status A carrier is an individual who harbors the organism but is not infected as measured by serologic studies (no evidence of an antibody response) or shows no evidence of clinical illness. This person can still infect others, although the intectivity is generally lower than with other infections. Carrier status may be of limited duration or may be chronic, lasting for months or years. One of the best-known examples of a long-term carrier was Mary Mallon, better known as Typhoid Mary, who carried Salmonella typhi and died in 1938. Over a period of many years, she worked as a cook in the New York City area, moving from household to household under different names. She was considered to have caused at least 10 typhoid fever outbreaks that included 51 cases and 3 deaths. Endemic, Epidemic, and Pandemic Three other terms must be defined: endemic, epidemic, and pandemic. Endemic is defined as the habitual presence of a disease within a given geographic area. It may also refer to the usual occurrence of a given disease within such an area (sometimes referred to as the "background rate of disease"). Epidemic is defined as the occurrence in a community or region of a group of illnesses of similar nature, clearly in excess of normal expectancy and derived from a common or a propagated source (Fig. 2.6). Pandemic refers to a worldwide epidemic. How do we know when we have an excess over what is expected? Indeed, how do we know how much to expect? There is no precise answer to either question. Through ongoing surveillance, we may determine what the usual or expected level may be. With regard to excess, sometimes an "interocular test" may be convinc-ing: the difference is so clear that it hits you between the eyes. Two examples will show how pandemics and fear of pandemics relate to the development of public policy. Patients with chronic kidney disease are often anemic, which is commonly corrected by injection of erythropoiesis-stimulating agents (ESAs); these are genetically engineered forms of the human erythropoietin hormone. The drug manufacturers pay doctors millions of dollars every year in return for prescribing this anemia medication, which led to extensive off-label use and the overutilization of ESAs in the United States (Fig. 2.7). In 2006, two clinical trials were published in the New England Journal of Medicine that raised concerns about the safety of using ESAs for anemia correction to optimal levels in patients with chronic kidney disease, as neither study anticipated these results. The first clinical trial, Cardiovascular Risk Reduction by Early Anemia Treatment with Epoetin Beta (CREATE),' showed that early and complete correction of anemia (to a target hemoglobin level in the normal range) failed to reduce the incidence of cardiovascular events as compared with the partial correction of anemia. The second trial, Correction of Hemoglobin and Outcomes in Renal Insufficiency (CHOIR), ' showed that a higher target hemoglobin value of 13.5 g/dL was associated with increased risk of death, myocardial infarction, hospitalization for congestive heart failure, and stroke, all without an improvement in quality of life as compared with a lower hemoglobin target of 11.3 g/dL (Fig. 2.8). As a result, in 2007, the US Food and Drug Administration issued a black box warning adding significant restrictions on the use of ESAs.' The black box warning includes the following: (1) prescribers should use the lowest dose of ESA that will gradually increase the hemoglobin concentration to the lowest level sufficient to avoid the need for red blood cell transfusion and (2) ESAs increase the risk for death and for serious cardiovascular events when administered to target a hemoglobin of greater than 12 g/dL. The second example involves an issue that arose in 2011 related to laboratory research into the H5N1, or "bird flu," virus (Fig, 2.9). Although transmission of naturally occurring H5N1 has been primarily limited to persons having direct contact with infected animals, in the unusual cases where people do acquire the infection from animals the disease is often very severe with a high mortality. There has therefore been serious concern that certain mutations in the virus might increase transmissibility of the virus to humans and could therefore result in a human pandemic. In order to understand fully the possibility of such a mutation and the potential for preventing it, two government-funded laboratories, one at Erasmus Medical Center in the Netherlands and a second at the University of Wisconsin-Madison in the United States, created genetically altered H5N1 strains that could be transmitted between mammals (ferrets) through the air. After reviewing the two studies, the US National Science Advisory Board for Biosecurity, for the first time in its history, recommended against publishing the details of the methodologies used in these studies. The board cited potential misuse by "those who would seek to do harm" by participating in bioterrorist activity: Other scientists, however, including members of an expert panel assembled by the World Health Organization (WHO), disagreed, stating that the work was important to public health efforts to prevent a possible pandemic in humans. In January 2012, a moratorium on some types of H5N1 research was self-imposed by the researchers to allow time for discussion of these concerns by experts and by the public. The results of the two studies were subsequently published in May and June of 2012.46.7 The major unresolved issue is whether the potential benefits to society from the results of these types of studies outweigh the risks from the uncontrolled spread of mutated virus, resulting from either lapses in biosafety in the laboratory (accidental release of the virus) or bioterrorist activity (intentional release of the virus). Scientists and policy makers are obliged to develop methods for assessing the risks and benefits of conducting different types of experimental research. In addition, these events illustrate that censorship and academic freedom in science remain highly relevant issues today. Disease Outbreaks Let us assume that a food becomes contaminated with a microorganism. If an outbreak occurs in the group of people who have eaten the food, it is called a common-vehicle exposure, because all the cases that occurred were in persons exposed to the suspected contaminated food. The food may be served only once—for example, at a catered luncheon—resulting in a single exposure to the people who eat it, or the food may be served more than once, resulting in multiple exposures to people who eat it more than once. When a water supply is contaminated with sewage because of leaky pipes, the contamination can be either periodic, causing multiple exposures as a result of changing pressures in the water supply system, which may cause intermittent contamina-tion, or continuous, in which case a constant leak leads to persistent contamination. The epidemiologic picture that is manifested depends on whether the exposure is single, multiple, or continuous. For purposes of this discussion, we will focus on the single-exposure, common-vehicle outbreak because the issues discussed are most clearly seen in this type of outbreak. What are the characteristics of such an outbreak? First, such outbreaks are generally explosive— that is, there is a sudden and rapid increase in the number of cases of the disease or condition in a popula-tion. (Interestingly, single-exposure common-vehicle epidemics of noncommunicable diseases, such as the epidemic of leukemia following the explosion of an atomic bomb in Hiroshima and Nagasaki, also seem to follow the same pattern.) Second, the cases are limited to people who share the common exposure. This is self-evident, because in the first wave of cases we would not expect the disease to develop in people who were not exposed unless there was another independent source of the disease in the community. Third, in a food-borne outbreak, cases rarely occur in persons who did not eat the food—that is, those who acquire the disease from a primary case who ate the food. The reason for the relative rarity of such secondary cases in this type of outbreak is not well understood. In the United States, the leading cause of food-borne-related illness is contamination with norovirus (from the Norwalk virus family). Globally, norovirus results in a total of $4.2 billion in direct health care system costs and $60.3 billion in societal costs per year.® Over recent decades, a growing number of outbreaks of acute gastroenteritis (AGE) have occurred aboard cruise ships. The Centers for Disease Control and Prevention (CDC) has reported that rates of AGE among passengers on cruise ships have decreased from 27.2 cases per 100,000 travel days in 2008 to 22.3 in 2014, while the rate among crew members was essentially unchanged.' This could potentially be attributed to the production of operational manuals or specific guidelines providing hygiene standards, increasing awareness among passengers and crew members, communicable disease surveillance programs, and preventive procedures in addition to regulatory enforcement and strict inspections through the CDCs Vessel Sanitation Program (VSP), which monitors outbreaks on cruise ships and works to prevent and control transmission of illness aboard these ships. (Data from each outbreak are available on their website, hup://www.cdc.gov/nceh/ vsp/.) In areas with a high prevalence of norovirus, particularly the recombinant GIl.2 type, such as in the provinces of Guangdong and Jiangsu, China, outbreaks of AGE continue to occur frequently. For example, on December 14, 2014, a student in third grade vomited in the classroom and washroom several times and was considered the earliest suspected case of norovirus. Over the following 3 days, 27 more cases were reported, which were mainly located on the fourth floor (12 cases) and third floor (9 cases) of the school building. Fig. 2.10 shows the epidemic curve with the number of cases each day. The first peak of the outbreak was on December 17, which was starting to taper off when implementation of control measures such as quarantine and disinfection were followed. However, a few days later, on December 25, the attack rate peaked again, with cases occurring mainly on the second floor (12 cases) and third floor (5 cases). In order to aggressively contain the outbreak, the school was closed temporarily and the outbreak came to an end on December 31. Immunity and Susceptibility The amount of disease in a population depends on a balance between the number of people in that population who are susceptible and therefore at risk for the disease and the number of people who are not susceptible or immune and therefore not at risk. They may be immune because they have had the disease previously (and have antibodies) or because they have been immunized. They also may not be susceptible on a genetic basis. Clearly if the entire population is immune, no epidemic can develop. But the balance is usually struck somewhere in between immunity and susceptibility, and when it moves toward susceptibility, the likelihood of an outbreak increases. This has been observed particularly in formerly isolated populations who were later exposed to disease. For example, in the 19th century, Panum observed that measles occurred in the Faroe Islands in epidemic form when infected individuals entered the isolated and susceptible population." In another example, severe outbreaks of streptococcal sore throats developed when new susceptible recruits arrived at the Great Lakes Naval Station.'2 Herd Immunity Herd immunity is defined as the resistance of a group of people to an attack by a disease to which a large proportion of the members of the group are immune. If a large percentage of the population is immune, the entire population is likely to be protected, not just those who are immune. Why does herd immunity occur? It happens because disease spreads from one person to another in any community. Once a certain proportion of people in the community are immune, the likelihood is small that an infected person will encounter a susceptible person to whom he can transmit the infection; more of his encounters will be with people who are immune. The presence of a large proportion of immune persons in the population lessens the likelihood that a person with the disease will come into contact with a susceptible individual. Why is the concept of herd immunity so important? When we carry out immunization programs, it may not be necessary to achieve 100% immunization rates to immunize the population successfully. We can achieve highly effective protection by immunizing a large part of the population; the remaining part will be protected because of herd immunity. For herd immunity to exist, certain conditions must be met. The disease agent must be restricted to a single host species within which transmission occurs, and that transmission must be relatively direct from one member of the host species to another. If we have a reservoir in which the organism can exist outside the human host, herd immunity will not operate because other means of transmission may be available. In addi-tion, infections must induce solid immunity. If immunity is only partial, we will not build up a large proportion of immune people in the community. What does this mean? Herd immunity operates if the probability of an infected person encountering every other individual in the population ("random mixing") is the same. But if a person is infected and all of his or her interactions are with people who are susceptible (i.e., there is no random mixing of the population), he or she is likely to transmit the disease to other susceptible people. Herd immunity operates optimally when populations are constantly mixing together. This is a theoretical concept because, obviously, populations are never completely randomly mixed. All of us associate with family and friends, for example, more than we do with strangers. However, the degree to which herd immunity is achieved depends on the extent to which the population approaches a random mixing. Thus we can interrupt the transmission of disease even if not everyone in the population is immune as long as a critical percentage of the population is immune. What percentage of a population must be immune for herd immunity to operate? This percentage varies from disease to disease. For example, in the case of measles, which is highly communicable, it has beenestimated that 94% of the population must be immune before the chain of transmission is interrupted. With decreasing childhood immunization rates in the United States associated with parental concerns regarding the risk of autism spectrum disorder, measles outbreaks are becoming more common. A total of 125 measles cases with rash occurred in a 6-week period; among these cases 110 were California residents (45% unvac-cinated), of whom 35% had visited one or both Disney theme parks between December 17 and 20, 2014, the suspected source of exposure. Of the secondary cases, most (26/34) were close contacts. An additional 15 cases associated with the Disney theme parks were reported in seven additional states." Let us consider poliomyelitis immunization and herd immunity. From 1951 to 1954, an average of 24,220 cases of paralytic poliomyelitis occurred in the United States each year. Two types of vaccine are available. The oral polio vaccine (OPV) protects not only those who are vaccinated but also others in the community through secondary immunity, produced when the vaccinated individual spreads the active vaccine virus to contacts. In effect, the contacts are immunized by the spread of virus from the vaccinated person. If enough people in the community are protected in this way, the chain of transmission is interrupted. However, even inactivated poliovirus vaccine (IPV), which does not produce secondary immunity (does not spread the virus to susceptibles), can produce herd immunity if enough of the population is immunized. Even those who are not immunized will be protected because the chain of transmission in the community has been interrupted From 1958 to 1961, only IPV was available in the United States. Fig. 2.11A shows the expected number of cases each year if the vaccine had protected only those who received the vaccine. Fig. 2.11B shows the number of polio cases actually observed. Clearly far fewer cases occurred than would have been expected from the direct effects of the vaccine alone. The difference between the two curves represents the effect of herd immunity from the vaccine. Thus nonimmunized individuals can gain some protection from either the OPV or IPV. Incubation Period The incubation period is defined as the interval from receipt of infection to the time of onset of clinical illness (the onset of recognizable symptoms). If you become infected today, the disease with which you are infected may not develop for a number of days or weeks. During this time, the incubation period, you feel completely well and show no signs of the disease. Why does disease not develop immediately at the time of infection? What accounts for the incubation period? It may reflect the time needed for the organism to replicate sufficiently until it reaches the critical mass needed for clinical disease to result. It probably also relates to the site in the body at which the organism replicates-whether it replicates superficially, near the skin surface, or deeper in the body (e.g., in the gut). The dose of the infectious agent received at the time of infection may also influence the length of the incubation period. With a large dose, the incubation period may be shorter. The incubation period is also of historical interest because it is related to what may have been the only medical advance associated with the Black Death (plague) in Europe. In 1374, when people were terribly frightened of the Black Death, the Venetian Republic appointed three officials who were responsible for inspecting all ships entering the port and for excluding ships that had sick people on board. It was hoped that this intervention would protect the community. In 1377, in the Italian seaport of Ragusa, travelers were detained in an isolated area for 30 days (trentini giorni) after arrival to see whether infection developed. This period was found to be insufficient, and the period of detention was lengthened to 40 days (quarante giorni). This is the origin of the word quarantine. How long would we want to isolate a person? We would want to isolate a person until he or she is no longer infectious to others (having passed through the suspected incubation period). When a person is clinically ill, we generally have a clear sign of potential intectiousness. An important problem arises before the person becomes clinically ill-that is, during the incubation period. If we knew when he or she became infected and also knew the general length of the incubation period for the disease, we would want to isolate the infected person during this period (and perhaps a few days extra to be especially cautious) to prevent transmission of the disease to others. In most situations, however, we do not know that a person has been infected, and we may not know until signs of clinical disease become manifest. In addition, we may not know the distribution of the incubation period. This leads to an important question: Is it worthwhile to quarantine—isolate—a patient, such as a child with chickenpox? The problem is that, during at least part of the incubation period, when the person is still free of clinical illness, he or she can transmit the disease to others. Thus we have people who are not (yet) clinically ill but who have been infected; they are unaware of their infection status and are able to transmit their disease. For many common childhood diseases, by the time clinical disease develops in the child, he or she has already transmitted the disease to others. Therefore isolating such a person at the point at which he or she becomes clinically ill will not necessarily be effective. On the other hand, isolation can be very valuable. In September 2012, health officials in Saudi Arabia first reported a severe acute respiratory illness with symptoms of fever, cough, and shortness of breath. The causative organism was shown to be the Middle East Respiratory Syndrome Coronavirus (MERS-CoV), which has an incubation period of about 5 or 6 days. MERS-CoV likely came from infected camels in the Arabian Peninsula and spread through person-to-person close contact, with health care personnel at higher risk of infection if universal precautions were not adhered to. All MERS-CoV cases that have been identified had a positive history of someone living in or traveling to countries in or near the Arabian Peninsula. Another outbreak of MERS-CoV occurred in the Republic of Korea in 2015 and was also linked to a returning traveler from the Arabian Peninsula. As of May 2017, WHO has reported that there had been 1952 laboratory-confirmed cases of infection with MERS-CoV from 27 countries, of whom 693 (36%) had died. Fig. 2.12 shows the epidemic curve of the confirmed global cases of MERS-CoV reported to WHO as of May 5, 2017. (Note that, unlike the epidemic curve for common vehicle epidemics, the curve for person-to-person spread is multimodal.) An outbreak of MERS-CoV in the Republic of Korea was seen in 2015 but was rather contained, whereas the epidemic remains active in Saudi Arabia. A major contributor to the Korean control of the epidemic was probably the strong infection control measures implemented early on for diagnosing and isolating probable MERS-CoV cases and for reducing interpersonal contacts of travelers with a history of travel to highly affected areas. Different diseases have different incubation periods. A precise incubation period does not exist for a given disease; rather, a range of incubation periods is characteristic of that disease. Fig. 2.13 shows the range of incubation periods for several diseases. In general the length of the incubation period is characteristic of the infective organism. The incubation period for infectious diseases has its analogue in noninfectious diseases. Thus, even when an individual is exposed to a carcinogen or other environmental toxin, the disease is often manifest only alter months or even years. For example, mesothelioma resulting from asbestos exposure may occur 20 to 30 years alter the exposure. The incubation period for noninfectious diseases is often referred to as the latency period. Fig. 2.14 is a graphic representation of an outbreak of Salmonella typhimurium at a medical conference in Wales in 1986. Each bar represents the number of cases of disease developing at a certain point in time after the exposure; the number of hours since exposure is shown along the horizontal axis. If we draw a line connecting the tops of the bars, it is called the epidemic curve, which is defined as the distribution of the times of onset of the disease. In a single-exposure, common-vehicle epidemic, the epidemic curve represents the distribution of the incubation periods. This should be intuitively apparent: if the infection took place at one point in time, the interval from that point to the onset of each case is the incubation period in that person. As seen in Fig. 2.14, involving Salmonella typhimu-rium, there was a rapid, explosive rise in the number of cases within the first 16 hours, which suggests a single-exposure, common-vehicle epidemic. In fact, this pattern is the classic epidemic curve for a single- exposure common-vehicle outbreak (Fig. 2.15, left). The reason for this configuration is not known, but it has an interesting property: if the curve is plotted against the logarithm of time rather than against time itself, the curve becomes a normal curve, which has useful statistical properties (see Fig. 2.15, right). If plotted on log-normal graph paper, we obtain a straight line, and estimation of the median incubation period is facilitated. Armenian and Lilienfeld'* showed that a log-normal curve is also typical of single-exposure common-vehicle epidemics of noninfectious diseases. The three critical variables in investigating an outbreak or epidemic are as follows: 1. When did the exposure take place? 2. When did the disease begin? 3. What was the incubation period for the disease? If we know any two of these, we can calculate the third. Attack Rate An attack rate is defined as: Number of people at risk in whom a certain illness develops/Total number of people at risk The attack rate is useful for comparing the risk of disease in groups with different exposures. The attack rate can be specific for a given exposure. For example, the attack rate in people who ate a certain food is called a food-specific attack rate. It is calculated by: In general, time is not explicitly specified in an attack rate because the exposure is common and the illness is acute; given what is usually known about how long after an exposure most cases develop, the time period is implicit in the attack rate. A person who acquires the disease from that exposure (e.g., from a contaminated food) is called a primary case. A person who acquires the disease from exposure to a primary case is called a secondary case. The secondary attack rate is therefore defined as the attack rate in susceptible people who were not exposed to the suspected agent who have been exposed to a primary case. It is a good measure of person-to-person spread of disease after the disease has been introduced into a population, and it can be thought of as a ripple moving out from the primary case. We often calculate the secondary attack rate in family members of the index case. The secondary attack rate also has application in noninfectious diseases when family members are examined to determine the extent to which a disease clusters among first-degree relatives of an index case (heritability or clustering within families), which may yield a clue regarding the relative contributions of genetic and environmental factors to the cause of a disease. Exploring Occurrence of Disease The concepts outlined in this chapter form the basis for exploring the occurrence of disease. When a disease appears to have occurred at more than an endemic (usual) level and we wish to investigate its occurrence, we ask: Who was attacked by the disease? When did the disease occur? Where did the cases arise? It is well known that disease risk is affected by all of these factors. WHO The characteristics of the human host are clearly related to disease risk. Factors such as sex, age, and race as well as behavioral risk factors (e.g., smoking) may have major effects. Gonorrhea As shown in Fig. 2.16, rates of gonorrhea have historically been higher in men than in women, and this sex difference is observed at least as far back as 1960 (not shown in this graph). Because women are more likely to be asymptomatic, the disease in women has probably been underreported. Rates had been leveling off in both men and women over the past few decades, but since 2013, higher rates of gonorrhea have been observed in men than in women. Such increases in rates among men could be either attributed to increased transmission or increased case ascertain-ment (e.g., through increased extragenital screening) among gay, bisexual, and other men who have sex with men. Pertussis The incidence of pertussis ("whooping cough") in the United States peaked in 2004; the rate reached 8.9 cases per 100,000 population, more than twice that reported in 2003. In 1994, the rate was 1.8. The number of cases in 2004 was the highest reported since 1959. Although childhood pertussis vaccine coverage is high in the United States, pertussis continues to cause morbidity. Some of this increase may result from improved diagnostics as well as recognition and reporting of cases. As seen in Fig. 2.17, the lowest rates for pertussis in the United States were observed in 1991. Although incidence rates showed two more peaks in 2008 and 2009, they subsequently declined until 2016. Of note, infants aged less than 1 year, who are at the greatest risk for death, continue to have the highest reported rate of pertussis. Pertussis occurrence is clearly related to age (Fig- 2.18). Although the highest rate of pertussis was in infants less than 6 months of age (99 per 100,000 population), the number of reported cases was highest in children ages 11 to 19. Approximately half of reported pertussis cases in 2014 and 2015 occurred in 10- to 19-year-olds and in adults over the age of 20 years. Although the specific cause of this phenomenon is unknown, it could result from a waning of protection 5 to 10 years after pertussis immunization. WHEN Certain diseases occur with a certain periodicity. For example, aseptic meningitis peaks at consistent yearly rates (Fig. 2.19). Often, there is a seasonal pattern to the temporal variation. For example, diarrheal disease is most common during the summer months, and respiratory disease is most common during the winter months. The question of when is also addressed by examining trends in disease incidence over time. For example, in the United States, both the incidence of and deaths from acquired immunodeficiency syndrome (AIDS) increased for many years, but it began to decline in 1996, largely as a result of new therapy and health education efforts. WHERE Disease is not randomly distributed in time or place. For example, Fig. 2.20 shows the geographic distribution of Lyme disease in the United States in 2015, with each dot representing one case of Lyme disease. There is a clear clustering of cases along the Northeast coast, in the north-central part of the country, and in the Pacific Coast region. The states in which established enzootic cycles of Borrelia burgdorferi, the causative agent, have been reported accounted for 95% of the cases. The distribution of the disease closely parallels that of the deer tick vector. A dramatic example of spread of disease is seen with West Nile virus (WNV) in the United States.'5 WNV was first isolated and identified in 1937 in the West Nile region of Uganda, and for many years it was found only in the Eastern Hemisphere. The basic cycle of the disease is bird-mosquito-bird. Mosquitoes become infected when they bite infected birds. When mosquitoes that bite both birds and humans become infected, they pose a threat to people. Most human infections are subclinical, but approximately 1 of 150 infections in recent years has resulted in meningitis or encephalitis.The risk of neurologic disease is significantly increased in people older than 50 years of age. Other symptoms include fever, nausea and vomiting, rash, headache, and muscle weakness. The case-fatality rate, or the proportion of people who develop the disease (cases) who then die of the disease, can be as high as 14%. Advancing age is a major risk factor for death from WNV, with one study reporting death nine times as frequently in older compared with younger patients. Treatment is supportive, and prevention is largely addressed through mosquito control and the use of insect repellents and bed nets. Tracking the distribution of the disease depends on surveillance for human cases and on monitoring birds and animals for the disease and deaths from the disease. Surveillance is discussed in further detail in Chapter 3. WNV was first identified in New York City in 1999. from 1999 to 2015. During the same reporting period, the WNV disease epidemic peaked during the month of September every year (Fig. 2.22). Much remains to be learned about this disease to facilitate treatment, prevention, and control. Outbreak Investigation The characteristics just discussed are the central issues in virtually all outbreak investigations. The steps for investigating an outbreak generally follow this pattern (Box 2.2). CROSS-TABULATION When confronted with several possible causal agents, as is often the case in a food-borne disease outbreak, a very helpful method for determining which of the possible agents is suspected to be the cause is called cross-tabulation. This is illustrated by an outbreak of food-borne streptococcal disease in a Florida jail reported some years ago by the CDC.16 In August 1974, an outbreak of group A β- hemolytic streptococcal pharyngitis (sore throat) affected 325 of 690 inmates. On a questionnaire administered to 185 randomly selected inmates, 47% reported a sore throat between August 16 and August 22. Based on a second questionnaire, food-specific attack rates for items that were served to randomly selected inmates showed an association between two food items and the risk of developing a sore throat: a beverage and an egg salad served at lunch on August 16 (Table 2.2). In Table 2.2, for each of the suspected exposures (beverage and egg salad), the attack rate was calculated for those who ate or drank the item (were exposed) and those who did not eat or drink the item (were not exposed). For both the beverage and the egg salad, attack rates are clearly higher among those who ate or drank the item than among those who did not. However, this table does not permit us to determine whether the beverage or the egg salad accounted for the outbreak. In order to answer this question, we use the technique of cross-tabulation. In Table 2.3, we again examine the attack rates in those who ate egg salad compared with those who did not, but this time we look separately at those who drank the beverage and those who did not. Looking at the data by columns, we see that both among those who ate egg salad and among those who did not, drinking the beverage did not increase the incidence of streptococcal illness (75.6% vs. 80% and 26.4% vs. 25%, respectively). However, looking at the data in the table rows, we see that eating the egg salad increased the attack rate of the illness, both in those who drank the beverage (75.6% vs. 26.4%) and in those who did not (80% vs. 25%). Thus, the egg salad is clearly implicated as the source of the infections. Further discussion of the analysis and interpretation of cross-tabulation can be found in Chapter 15. This example demonstrates the use of cross-tabulation in a food-borne outbreak of an infectious disease, but the method has broad applicability to any condition in which multiple etiologic factors are suspected. It is discussed further in Chapter 15. Sometimes multiple agents are responsible for an outbreak. An example is a cruise-ship outbreak of gastrointestinal illness that occurred on the same day as a rainstorm, resulting in billions of liters of storm runoff being contaminated with sewage that had been released on the lake where the cruise took place. The cross-tabulation showed that passengers consuming ice had an attack rate more than twice as high as the rate among those who did not consume ice. Stool specimens were positive for multiple agents, including Shigella sonnei and Giardia." Conclusion This chapter reviewed some basic concepts that underlie the epidemiologic approach to acute communicable diseases. Many of these concepts apply equally well to noncommunicable diseases that at this time do not appear to be primarily infectious in origin. Moreover, for an increasing number of chronic diseases originally thought to be noninfectious, infection seems to play some role. Thus hepatitis B infection is a major cause of primary liver cancer. Papillomaviruses and Helicobacter pylori infections are necessary for the development of cervical and gastric cancers, respectively. Epstein-Barr virus has been implicated in Hodgkin disease. The boundary between the epidemiology of infectious and noninfectious diseases has blurred in many areas. In addition, even for diseases that are not infectious in origin, inflammation may be involved, the patterns of spread share many of the same dynamics, and the methodologic issues in studying them are similar. Many of these issues are discussed in detail in Section Il.