A History of Evolutionary Thought PDF
Document Details
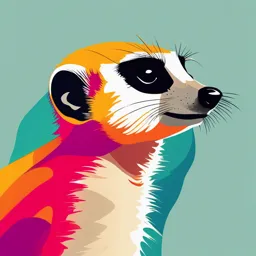
Uploaded by AppreciatedArcticTundra8766
Saskatchewan Distance Learning Centre
Joylin Namie
Tags
Related
- Lecture 12: Adaptation & EvoDevo PDF
- Smoking Prevalence & Music: Biological Rationales (PDF)
- Biological Evolution PDF
- Biological Psychology Summary (Tilburg University, 2023-2024) PDF
- Psychobiology Summary - Biological Psychology - Tilburg University 2023-2024 PDF
- Campbell Biology, 12th Edition Unit 5 PDF
Summary
This book explores the history of evolutionary thought, from ancient Greek philosophers to modern scientific discoveries. It discusses key concepts like natural selection and the Modern Synthesis within the context of biological anthropology. Readers will gain an understanding of how our understanding of evolution has developed.
Full Transcript
Home Read Sign in Search in book … Want to create or adapt books like this? Learn more about how Pressbooks supports open publishing practices. CONTENTS...
Home Read Sign in Search in book … Want to create or adapt books like this? Learn more about how Pressbooks supports open publishing practices. CONTENTS EXPLORATIONS: AN OPEN INVITATION TO BIOLOGICAL ANTHROPOLOGY, 2ND EDITION 2. A History of Evolutionary Thought Joylin Namie, Ph.D., Truckee Meadows Community College Learning Objectives Identify and describe the major developments in scientific thought that led to the discovery of evolutionary processes. Explain how natural selection works and results in evolutionary change over time. Explain what is meant by the “Modern Synthesis” and its impacts on evolutionary thought. Previous: Introduction to Biological Anthropology Next: Molecular Biology and Genetics Discuss the teaching of human evolution in the U.S. and abroad. The Beginnings of Evolutionary Thinking Throughout our evolutionary history, humans have developed an understanding of the natural world as they interacted with and extracted resources from it.To survive, our earliest ancestors possessed an understanding of the physical environment, including weather patterns, animal behavior, edible and medicinal plants, locations of water, and seasonal cycles. Many ancient cultures, including those of the Americas (Dunbar-Ortiz 2014), Mesopotamia, and Egypt, left writings, hieroglyphics, and stories passed down through oral tradition detailing their understanding of the natural environment, human and zoological anatomy, botany, and medical practices (Moore 1993). There are also over 2,000 years of organized thought and writing regarding evolution, including contributions from Greek, Roman, and Islamic scholars. Three examples of note are included here. The Greek philosopher Aristotle (384–322 BCE) studied the natural world, publishing several volumes on animals based on systematic observations, rather than attributing what he observed to divine intervention, as his contemporaries were doing (Figure 2.1). Aristotle’s system for the biological classification of nearly 500 species of animals was based on his own observations and dissections, interviews with specialists such as beekeepers and fishermen, and accounts of travelers. His nine book History of Animals, published in the 4th century BC (n.d.), was one of the first zoological taxonomies ever created. Aristotle’s primary contribution to the classification of biological species was to recognize that natural groups are based on structure, physiology, mode of reproduction, and behavior (Moore 1993, 39). Figure 2.1a-b: Aristotle was the first to publish that a. octopuses can change their colors when disturbed and b. elephants use their trunks as a snorkel when crossing deep water. Credit: a. Octopus macropus by SUBnormali Team (originally from Yoruno) is under a CC-BY-SA 3.0 License. b. Elephant swimming, Botswana (cropped) by Jorge Láscar from Australia (uploaded by Peter D. Tillman) is under a CC BY 2.0 License. Aristotle’s History of Animals also placed animals in a hierarchy, ranking animals above plants due to what he claimed were their abilities to sense the world around them and to move. He also graded animals according to their modes of reproduction. Those giving birth to live young were placed above those who laid eggs. Warm-blooded animals ranked above invertebrates. This concept of “higher” and “lower” organisms was expanded upon by scholars in the Medieval period to form the Scala Naturae (Latin for “ladder of being”). This “Great Chain of Being,” depicting a hierarchy of beings with God at the top and minerals at the bottom (Figure 2.2), was thought by medieval Christians to have been decreed by God; in this Great Chain, humans were placed closer to God than other species. Aristotle’s works were rediscovered by Islamic scholars in the ninth century and translated into Arabic, Syriac, Persian, and later into Latin, becoming part of university curriculum in 13th- Figure 2.2: The Great Chain of Being century Europe (Lindberg 1992), allowing Aristotle’s works and by Didacus Valades. Credit: Great Chain of Being by Didacus Valades ideas to influence other thinkers for 2,000 years. (Diego Valades 1579), print from Rhetorica Christiana (via Getty Science also owes a debt to many Research), is in the public domain. Arabic scholars. One such Islamic scholar and writer, who built upon the ideas of Aristotle, was Abū ʿUthman ʿAmr ibn Baḥr al-Kinānī al- Baṣrī / al-Jāḥiẓ, known as Al-Jahiz (776–868 CE), who authored over 200 books (El-Zaher 2018; Figure 2.3). His most well-known work was the seven-volume Kitab al-Hayawan or Book of Animals, in which he described over 350 species in zoological detail. Importantly, Al-Jahiz introduced the idea and mechanisms of biological evolution 1,000 years before Darwin proposed the concept of natural selection in 1859 (Love 2020). For instance, Al-Jahiz wrote about the struggle for existence, the transformation of species over time, and environmental factors that influence the process, all ideas that were later espoused by western European scientists in the 19th century. Al-Jahiz wrote: Figure 2.3: An image from Kitāb al- ḥayawān (Book of the Animals) by Animals engage in a struggle for existing, and for resources, to Al-Jahiz. Credit: Al-Jahiz by Al-Jahiz avoid being eaten, and to breed. Environmental factors influence [in Kitāb al-ḥayawān (Book of the organisms to develop new characteristics to ensure survival, thus Animals), 15th century] is in the transforming them into new species. Animals that survive to public domain. breed can pass on their successful characteristics to their offspring. [Masood 2009] Another important early Islamic scientist is Ibn al- Haytham (965–1040), a 10th-century Islamic scholar who contributed a great deal to our understanding of optics and how human vision works (Figure 2.4; Lindberg 1992, 177–180). Born in what is now Iraq, al-Haytham was a scholar of many disciplines, including mathematics, physics, mechanics, astronomy, philosophy, and medicine. He authored some 200 books in his lifetime and was an expert on Aristotle’s natural philosophy, logic, and metaphysics. In relation to evolution, al-Haytham’s methodology of investigation—specifically, using experiments to verify theory—is similar to what later became known as the modern scientific method. He is most famous for discovering the laws of reflection and Figure 2.4: Drawing of Ibn al-Haytham. Credit: refraction over 1,000 years ago and inventing the camera Ibn al-Haytham by Sopianwar is under a CC obscura, which was incredibly important for the eventual BY-SA 4.0 License. development of photography. His work is credited for its influence on astronomy, mathematics, and optics, inspiring Galileo, Johannes Kepler, and Sir Isaac Newton (Tasci 2020). As an inspirational scientific figure, al- Haytham was celebrated in 2016 by UNESCO as a trailblazer and the founder of modern optics (Figure 2.5). An International Year of Light was named in his honor and a scholarly conference on his contributions was held to coincide with the 1,000th anniversary of the publication of his Kitāb al- Manāẓir (Book of Optics; UNESCO.org 2015). The writings of these Islamic scholars as well as similar scientific texts from other cultures were unknown to or unacknowledged by Western scientists until recently. Fortunately, many science teachers are now incorporating this content into their classes (Love 2020). Western European Evolutionary Thought Although there have been many different scientific traditions throughout world history, a new global discourse around science emerged in Western Europe in the 19th century. Europeans pointed to the continuing expansion of their colonial power, as well as their military and technological success, as evidence of the efficacy of Western science, which came to dominate on a global scale (Elshakry 2010). The movement toward a global science centered in Western Europe began with formulation of the Scientific Method. Figure 2.5: Diagram of the Human Eye by Ibn al-Haytham. The Scientific Method was first codified by Francis Bacon (1561–1626), an Credit: Diagram of the eye by Ibn English politician who was likely influenced by the methods of inquiry Al [Alhazen] Haitham (16th Century) has been modified established by Ibn al-Haytham centuries prior (Tbakhi and Amr 2007). (cropped) and is under a CC BY Bacon has been called the founder of empiricism for proposing a system 4.0 License. This image is for weighing the truthfulness of knowledge based solely on inductive available from Wellcome Images reasoning and careful observations of natural phenomena. Ironically, he 3044 (under the photo number died as a result of trying to scientifically observe the effects of cold on L0011969). the putrefaction of meat. On a journey out of London, he purchased a chicken and stuffed it with snow for observation, catching a chill in the process. One week later, he died of bronchitis (Urbach, Quinton, and Lea 2023). The second important development with regard to evolution was the concept of a species. John Ray (1627–1705), an English parson and naturalist, was the first person to publish a biological definition of species in his Historia Plantarum (History of Plants), a three volume work published in 1686, 1688, and 1704. Ray defined a species as a group of morphologically similar organisms arising from a common ancestor. However, we now define a species as a group of similar organisms capable of producing fertile offspring. In keeping with the scientific method, Ray classified plants according to similarities and differences that emerged from observation. He claimed that any seed from the same plant was the same species, even if it had slightly different traits. The modern period of biological classification began with the work of Carl von Linne (“Carolus Linnaeus”) (1707–1778), a Swedish scientist who laid the foundations for the modern scheme of taxonomy used today. He established the system of binomial nomenclature, in which a species of animal or plant receives a name consisting of two terms: the first term identifies the genus to which it belongs and the second term identifies the species. His original Systema Naturae, published in 1736, went through several editions. By the tenth edition in 1758, mammals incorporated primates, including apes and humans, and the term Homo sapiens was introduced to signify the latter (Paterlini 2007). Georges-Louis Leclerc, Comte de Buffon (1707–1788), was a prominent French naturalist whose work influenced prominent scientists in the second half of the 18th century. Buffon’s idea that species change over time became a cornerstone of modern evolutionary theory. His technique of comparing similar structures across different species, called comparative anatomy, is still in use today in the study of evolution. He published 36 volumes of Histoire Naturelle during his lifetime and heavily influenced two prominent French thinkers who were to have significant impacts on our understanding of evolution, Georges Cuvier and Jean-Baptiste Lamarck. Georges Cuvier (1769–1832) was a paleontologist and comparative anatomist (Figure 2.6). One of his first major contributions to the field of evolution was proof that some species had become extinct through detailed and comprehensive analyses of large fossil quadrupeds (Moore 1993, 111). The idea of extinction was not new, but it was challenging to demonstrate if a fossil species was truly extinct or still had living relatives elsewhere. It was also Figure 2.6: Cuvier with one of his drawings of a fossil challenging in that it ran counter to religious quadruped. Credit: Cuvier and a fossil quadruped original to beliefs of the time. The Bible’s Book of Explorations: An Open Invitation to Biological Anthropology (2nd ed.) is a collective work under a CC BY-NC-SA 4.0 License. Genesis was interpreted as saying that all [Includes Georges Cuvier 3 by François-André Vincent (artist), species had been created by God in the public domain; Mammoth skeleton in OpenClipart, public seven days it took to create the world and domain (CC0).] that all created species have survived to this day. Extinction was interpreted as implying imperfection, suggesting God’s work was flawed. Also, given that the Earth was calculated to have been created in 4004 B.C.E., based on biblical genealogies, there would not have been enough time for species to disappear (Moore 1993, 112). Cuvier was so knowledgeable in this field that he became famous for his ability to reconstruct what an extinct animal looked like from fragmentary remains. He demonstrated that fossil mammoths differed from similar living creatures, such as elephants. His many examples of fossils telling the stories of animals that lived and then disappeared were taken as incontrovertible proof of extinctions (PBS 2001). Where Cuvier went awry was his hypothesis of how extinction worked and its causes. As part of his study of comparative anatomy, Cuvier made observations of stratified layers of rock, or sediment, each containing different species. From this, he drew conclusions that species were “fixed” and did not evolve, but then went extinct, and that different assemblages of fossils occurred at different times in the past, as evidenced by the sedimentary layers (Moore 1993, 118). Cuvier explained this through a theory of catastrophism, which stated that successive catastrophic deluges (akin to Biblical floods) swept over parts of the Earth periodically, exterminating all life. When the waters receded from a particular region, lifeforms from unaffected regions would repopulate the areas that were destroyed, giving rise to a new layer of species that looked different from the layer below it. This theory implied that species were fixed in place and did not evolve and that the Earth was young. In fact, Cuvier postulated that the last catastrophe was a deluge he believed occurred five to six thousand years ago, paving the way for the advent of humans (Moore 1993, 118). Cuvier’s catastrophism became part of an ongoing and vociferous debate between two schools of geology. The catastrophists believed the present state of the earth was the consequence of a series of violent catastrophes of short duration, while the uniformitarians thought it was the result of slow acting geological forces that continue to shape the earth. James Hutton (1726–1797) was one prominent proponent of uniformitarianism. Based on evidence he found at sites in his native Scotland, Hutton argued that the Earth was much older than previously thought. Examining the geology of Siccar Point, a cliff site on the eastern coast of Scotland (Figure 2.7), Hutton concluded that the intersection of the vertical and horizontal rocks represented a gap in time of many millions of years, during which the lower rocks had been deformed and eroded before the upper layers were deposited on top. From this, Hutton argued sediments are deposited primarily in the oceans, where Figure 2.7: Siccar Point, Aberdeen, UK. Credit: Siccar Point by Anne Burgess is under a CC BY-SA 2.0 they become strata, or layers of sedimentary rock. License. Volcanic action uplifts these strata to form mountains, which are then subject to erosion from rain, rivers, and wind, returning sediment to the oceans (Moore 1993, 121). Hutton’s Theory of the Earth (1788) demanded vast periods of time (known as “deep time”) for such slow-working forces to shape the earth. At the time, he was heavily criticized for this view, as it contradicted the biblical version of the history of creation. Another Scotsman, who was to become a highly influential geologist and a close friend of Darwin, was Charles Lyell (1797–1875). Lyell was originally a lawyer who began his studies of Geology at Oxford under the tutelage of catastrophist William Buckland, from whom he diverged when Buckland tried to find physical evidence of Noah’s flood from the Christian Bible. Lyell was instead intent on establishing geology as a science based on Figure 2.8: The frontispiece from Charles Lyell’s observation. Building upon Hutton’s ideas (published Principles of Geology (2nd American edition, 1857), 50 years earlier), Lyell traveled throughout Europe, showing the origins of different rock types. Credit: Lyell Principles frontispiece by Charles Lyell (Principles documenting evidence of uniformitarianism. During of Geology, 2nd American edition, 1857) is in the public his travels, he cataloged evidence of sea level rise domain. and fall and of volcanoes positioned atop much older rocks. He also found evidence of valleys formed through erosion, mountains resulting from earthquakes, and volcanic eruptions that had been witnessed or documented in the past (University of California Berkeley Museum of Paleontology n.d.). Lyell also espoused the principle that “rocks and strata (layers of rock) increase in age the further down they are in a geological sequence. Barring obvious upheavals or other evidence of disturbance, the same principle must apply to any fossils contained within the rock. The lower down in a sequence of rocks a fossil is, the older it is likely to be (Wood 2005, 12).” Lyell published the first edition of his three-volume Principles of Geology in 1830–1833 (Figure 2.8). It established geology as a science, underwent constant revisions as new scientific evidence was discovered, and was published in 12 editions during Lyell’s lifetime. In it, he espoused the key concept of uniformitarianism—that “the present is the key to the past.” What this meant was that geological remains from the distant past can be explained by reference to geological processes now in operation and directly observable. Jean-Baptiste Lamarck (1744–1829) was the first Western scientist to propose a mechanism explaining why and how traits changed in species over time, as well as to recognize the importance of the physical environment in acting on and shaping physical characteristics. Lamarck’s view of how and why species changed through time, known as the “Theory of Inheritance of Acquired Characteristics,” was first presented in the introductory lecture to students in his invertebrate zoology class at the Museum of Natural History in Paris in 1802 (Burkhardt 2013). It was based on the idea that as animals adapted to their environments through the use and disuse of characteristics, their adaptations were passed on to their offspring through reproduction (Figure 2.9). Lamarck was right about the environment having an influence on characteristics of species, as well as about variations being passed on through reproduction. He simply had the mechanism wrong. Figure 2.9a-b: Inheritance of Acquired Characteristics and Natural Selection. Credit: Lamarckian Evolution (Figure 4.2A and 4.2B) original to Explorations: An Open Invitation to Biological Anthropology by Mary Nelson is under a CC BY-NC 4.0 License. Lamarck’s theory involved a three-step process. Step one involves an animal experiencing a radical change in its environment. Step two is the animal (either individual or species) responding with a new kind of behavior. Step three is how the behavioral change results in morphological (meaning physical) changes to the animal that are successfully passed on to subsequent generations (Ward 2018, 8). Lamarck’s most famous example was the proposition that giraffes actively stretched their necks to reach leaves on tall trees to eat. Over their lifetimes, the continuation of this habit resulted in gradual lengthening of the neck. These longer necks were then passed on to their offspring. Lamarck’s theory was disproved when evolutionary biologist August Weismann published the results of an experiment involving mice (Figure 2.10). Weismann amputated the tails of 68 mice and then successively bred five generations of them, removing the tails of all offspring in each generation, eventually producing 901 mice, all of whom had perfectly healthy long tails in spite of having parents whose tails were missing (Weismann 1889). Figure 2.10: Weismann’s mouse-tail experiment showing that offspring do not inherit traits that the parents acquired during their lifetimes. Credit: Weismann’s mouse-tail experiment (Figure 4.3) original to Explorations: An Open Invitation to Biological Anthropology by Mary Nelson is under a CC BY- NC 4.0 License. How giraffes actually ended up with long necks is a different story. In an environment where the food supply is higher off the ground, and perhaps less available to competing species, giraffes who happened to have slightly longer necks (due to random individual variation and genetic mutation) would be more likely to survive. These giraffes would then be able to reproduce, passing along the slight variation in neck length that would allow their offspring to do the same. Over time, individuals with longer necks would be overrepresented in the population, and neck lengths overall would increase among giraffes. Unfortunately, Lamarck’s ideas challenged the scientific establishment of the time and were rejected. He was discredited and harassed “to the point of loss of money, reputation, and then health” (Ward 2018, 9). The final piece in the evolutionary puzzle leading up to the theory of natural selection was put forth by Thomas Malthus (1766–1834), who published An Essay on Population in 1798. Malthus lived in England during the time of the Industrial Revolution. It was a time of great poverty and misery when many people migrated from the countryside to squalid, disease-ridden cities to work extremely long hours in dangerous conditions in factories, coal mines, and other industrial workplaces. Birth rates were high and starvation and disease were rampant. Malthus struggled to explain why. His answer was basically the idea of carrying capacity, an ecological concept still in use today. Malthus suggested the rate of population growth exceeded the rate of increase of the human food supply. In other words, people were outgrowing the available food crops. He also suggested that populations of animals and plants were naturally constrained by the food supply, resulting in reductions in population in times of scarcity, “restraining them within the prescribed bounds” (Moore 1993, 147). But, despite significant challenges, some individuals always survived. This was the key to later understandings of evolutionary change in species over time. The Journey to Natural Selection In Western European thought, the individual most closely associated with evolution is Charles Darwin (1809–1882). However, as one can see from the individuals and ideas presented in the prior section, he was not the first person to explore evolution and how it might work. In fact, Darwin built upon and synthesized many of the ideas—from geology to biology, ecology, and economy—discussed above. He was simply in the right place at the right time. If he had not worked out his ideas when he did, someone else would have. As a matter of fact, as noted below, someone else did, forcing Darwin to publicly reveal his theory. Darwin’s journey to the discovery of natural selection began during a childhood spent being curious, experimenting, and collecting natural specimens. When Darwin was 12 years old, his nickname was “Gas” because of the foul-smelling chemistry experiments he and his older brother, Erasmus, performed late into the evenings in their makeshift laboratory in the garden of their parent’s home (Costa 2017). Darwin was also a lifelong collector of biological and geological specimens, most famously beetles, at times going to great lengths in pursuit of a new specimen, as one of his personal letters relates, I will give a proof of my zeal: one day, on tearing off some old bark, I saw two rare beetles, and seized one in each hand; then I saw a third and new kind, which I could not bear to lose, so that I popped the one which I held in my right hand into my mouth. Alas! it ejected some intensely acrid fluid, which burnt my tongue so that I was forced to spit the beetle out, which was lost, as was the third one. [Darwin 2001 (1897), 50]. Darwin continued his observations and experiments during his formal education, culminating in his graduation from Cambridge in 1831, at which point he was invited to become a gentleman naturalist for a British Royal Navy surveying mission of the globe aboard the H.M.S. Beagle. It is worth noting that Darwin was only 22 years old and the captain’s third choice for the position (Costa 2017), but he proved extremely curious and methodical. The mission departed in December of 1831 and returned five years later (Figure 2.11). During this time, Darwin produced copious notebooks, observations, drawings, and reflections on the natural phenomena he encountered and the experiments he performed. Figure 2.11: Map of the voyage of the H.M.S. Beagle. Credit: Voyage of the Beagle-de by Succu is under a CC BY-SA 3.0 License. [Image Description]. Discussing all of Darwin’s work aboard the Beagle is beyond the scope of this chapter, but his primary interests were in cataloging new varieties of plant and animal life and examining the geology of the places the ship made landfall. Part of Darwin’s success with regard to both ventures was due to his extreme seasickness, which began before the ship even left Plymouth Harbor. It never let up, encouraging Darwin to go ashore at every available opportunity. “In fact, of the nearly five years of the voyage, Darwin was actually on board the ship for just a year and a half altogether” (Costa 2017, 18). During the voyage, the young Darwin tried to make sense of what he saw through the lens of the scientific paradigms he held when he left England, but he continually made observations that challenged these paradigms. For example, while the Beagle crewmen were charting the coast of Argentina, Darwin conducted fieldwork on land. There he observed species that were new to him, like armadillos. He also collected fossils, including those of extinct armadillos. Meaning, he had found both extant and extinct members of the same species in the same geographic location, which challenged the theory of catastrophism put forth by Cuvier, who argued that each variant of an animal, living or extinct, was its own distinct species (Moore 1993, 144). Darwin also observed geographic variation in the same species all along the east coast of South America, from Brazil to the southern tip of Argentina. He noted that some species were found in multiple localities and differed from place to place. Those living closer to each other exhibited only slight variations, while those living further apart might be cataloged as entirely different species if one did not know better. He made similar observations in the Galapagos Islands located off the northwest coast of Ecuador, with regard to giant tortoises and finches (Figure 2.12). A local resident of the islands explained to Darwin that each island had its own variety of tortoise and that locals could discern which island a tortoise came from simply by looking at it. Darwin noted other such examples in both plants and animals, meaning geographic variation was occurring on separate, neighboring islands. Figure 2.12: Variation in giant tortoises in the Galapagos Islands. Credit: Giant Tortoises of the Galapagos Islands original to Explorations: An Open Invitation to Biological Anthropology (2nd ed.) by Mary Nelson and Katie Nelson is under a CC BY-NC 4.0 License. Prevailing views of time argued that variations in living species, and even the fossil armadillos and the living armadillos, were the result of separate creation events. According to this view, each variation, no matter how slight, was a different species. Challenging these ideas would mean challenging not only catastrophism, but the Fixity of Species and other well-accepted ideas of the time. Darwin was aware that he was a young, unestablished naturalist. He was also aware of the ruin that befell Lamarck when his theories were rejected. Lastly, Lyell, who was a good friend of Darwin’s, rejected evolution altogether. It is no wonder that Darwin published a great deal about the geological and fossil data he collected when he returned from the voyage, but not his early hypotheses about evolution. Upon Darwin’s return to England, it took another twenty years of data collection and experimentation before he was ready to share his conclusions about evolution. Much of this work was conducted at Down House, his home of forty years, where he performed all sorts of experiments that laid the groundwork for his ideas about evolution. Darwin’s home was his laboratory, and he engaged the help of his children, neighbors, friends, and servants in collecting, dissecting, and experimenting. At one point in the 1850s, sheets of moistened paper covered with frogs eggs lined the hallways of the house, while flocks of sixteen different pigeon breeds cooed outside, glass jars filled with salt water and floating seeds filled the cellar, and the smell of dissected pigeon skeletons pervaded the air inside the house. There were also ongoing experiments in the yard, including piles of dissected flowers, beekeeping, and fenced-off plots of land where seedlings were under study. Darwin was a keen experimental scientist, observer, and a prolific writer and presenter of scientific papers. He regarded his work as “one long argument” that never really ended. In fact, Darwin published ten books after On the Origin of Species, addressing such far- ranging topics as animal behavior, orchids, and domestication, among others (Costa 2017). Darwin may not have published Origins in 1859 had it not been for receiving a paper in June of 1858 from Alfred Russel Wallace, an English naturalist working in Malaysia, espousing the same ideas. Wallace had sent the paper to Darwin asking if it was worthy of publication and requesting he forward it to Lyell and the English botanist, Joseph Hooker. Darwin wrote to Lyell and Hooker about Wallace’s paper, entitled On the Tendency of Varieties to Depart Indefinitely from the Original Type. In recognition that both Wallace and Darwin had arrived at the same discovery, a “joint” paper composed of four parts (none of them actually coauthored) was read to the Linnaean Society by Lyell, then secretary of the Society, on July 1, 1858, and published on August 20. Darwin published On the Origin of Species 15 months later. (The original composite paper read before the Linnaean society is available to read for free from the Alfred Russell Wallace Website, on the 1858 Darwin-Wallace paper page.) The Mechanism of Natural Selection On the subject of natural selection and how it works, let’s hear from Darwin himself from the original publication of On the Origin of Species (1859): A struggle for existence inevitably follows from the high rate at which all organic beings tend to increase. Every being, which during its natural lifetime produces several eggs or seeds, must suffer destruction during some period of its life, and during some season or occasional year, otherwise, on the principle of geometric increase, its numbers would quickly become so inordinately great that no country could support the product. Hence, as more individuals are produced than can possibly survive, there must in every case be a struggle for existence…It is the doctrine of Malthus applied with manifold force to the whole animal and vegetable kingdoms…There is no exception to the rule that every organic being naturally increases at so high a rate, that if not destroyed, the earth would soon be covered by the progeny of a single pair. …Owing to this struggle for life, any variation, however slight and from whatever cause proceeding, if it be in any degree profitable to an individual of any species…will tend to the preservation of that individual, and will be inherited by its offspring. The offspring, also, will thus have a better chance of surviving, for, of the many individuals of any species which are periodically born, but a small number can survive. I have called this principle, by which slight variation, if useful, is preserved, by the term Natural Selection. [Darwin 1859, 61–62] Let us take a moment here to explore the mechanism of natural selection in more detail. Before we begin, it is important to recognize that Darwin defined evolution as descent with modification, by which he meant that species share a common ancestor yet change over time, giving rise to new species. Descent with modification refers to the fact that offspring from two parents look different from each of their parents, and from each other, meaning they descend with slight differences (“modifications”). If you have ever observed a litter of puppies or a field of flowers and stopped to examine each individual closely, you have seen that each differs from the next, and none look exactly like their parents. These variations are random, not specific, and may or may not be present in the following generations. Darwin struggled to explain why some slight differences were preserved over time, while others were not. He turned to what he knew of animal breeding (artificial selection) for an explanation (Richards 1998). Darwin bred different breeds of pigeons at Down House, carefully documenting phenotypic differences across generations, including slight anatomical variations he observed through dissection. He also grew and crossbred species of flowers and dissected those too. Darwin was also very fond of hunting and of hunting dogs. In an early draft of his theory on speciation, he used greyhounds as an example of adaptation and selection, “noting how its every bone and muscle, instinct and habit, were fitted to run down hare (rabbits) (University of Cambridge n.d.).” In each case of plant and animal breeding Darwin observed, he noted that humans were selecting variants in each generation that had characteristics humans desired (i.e., sweetness of fruits, colors of flowers, fur type and color of animals). Breeders then continually bred plants and animals with the desired variants, over and over again. These small changes added up over time to create new species of plants and breeds of animals. Darwin also noted that artificial selection does not necessarily render plants or animals better adapted to their original environments. The characteristics humans desire often result in plants less likely to survive in the wild and animals more likely to suffer from certain behavioral or health problems. One has only to examine high rates of hip dysplasia in several modern breeds of dogs to observe what Darwin was referring to. From his studies of artificial selection, Darwin drew the conclusion that nature also acts upon variations among members of the same species. Instead of human intervention, the forces of nature, such as heat, cold, predation, disease, and now climate change, determine which offspring, with which variants, survive and reproduce. These individuals then pass down these favorable variants to their own offspring. In this way, nature selects for traits that are beneficial within a particular environment and selects against traits that are disadvantageous within a particular environment. Over many generations, populations of a species become more and more adapted (or, in evolutionary terms, “fit”) for their specific environments. Darwin named this process natural selection. This theory explained the variations in tortoises Darwin had observed years earlier in the Galapagos Islands (see Figure 2.12). Tortoises who lived on larger islands with lush vegetation to feed on were larger than those on smaller islands. They also had shorter necks and dome-shaped shells as their food was close to the ground. Tortoises on smaller, drier islands fed on cacti, which grew much taller. These tortoises had longer necks, longer front legs, and saddle-shaped shells, which allowed them to successfully stretch to reach the edible cactus pads that grew on the tops of the plants. How did these observable differences in the two tortoise populations emerge? Darwin would argue that, over time, small, random variations in the tortoises were differentially selected for by the distinct natural environments on different islands. In addition to the biogeographical evidence Darwin offered from his research aboard the Beagle, as well as the evidence he documented from the artificial selection of plants and animals, he also relied, where possible, on fossil evidence. One example, mentioned above, were the fossil findings of extinct armadillos in Argentina in the same locations as living armadillos. Unfortunately, as Darwin himself noted, the geological record was incomplete, most often missing the transitional fossil forms that bridge extinct and living species. That issue has since been resolved with scientific research in geochronology and paleontology, among other fields. It is now well-established that life is far more ancient than was believed in Darwin’s time and that these ancient forms of life were the ancestors to all life on this planet (Kutschera and Niklas 2004). What Darwin was Missing Although the theory of evolution by natural selection gained traction in scientific circles in the decades after Darwin’s publication of Origins, he was never able to discover the mechanisms that caused variation within members of the same species or the means by which traits were inherited. This began later in 1892 with the publication of The Germ-Plasm: A Theory of Heredity by August Weismann, the same Weismann of the mouse tail experiment presented earlier in this chapter. In his book, Weissman proposed a theory of germ-plasm, which was a precursor to the later discovery and understanding of DNA. Weismann specialized in cytology, a branch of biology devoted to understanding the function of plant and animal cells. Germ-plasm, he proposed, was a substance in the germ cells (what we would call gametes, or sex cells, today) that carried hereditary information. He predicted that an offspring inherits half of its germ-plasm from each of its parents, and claimed that other cells (e.g. somatic, or body, cells) could not transmit genetic information from parents to offspring. This thereby erased the possibility that acquired traits (which he argued resided in somatic cells) could be inherited (Zou 2015). This contribution to evolutionary theory was an important step toward understanding genetic inheritance, but a connection between genetics and evolution was still lacking. A series of lectures by a deceased Augustinian monk named Gregor Mendel (1822–1884), originally published in 1865, changed that perspective (Moore 1993, 285). Although Darwin was unknown to Mendel, he began a series of experiments with pea plants shortly after the publication of Darwin’s Origins, aiming to add to evolutionary understandings of heredity. As Mendel bred different generations of pea plants that had differences in seed shape and color, pod shape and color, flower position, and stem length, he documented consistent expression of some variations over others in subsequent generations. He meticulously documented the statistics of each crossing of plants and the percentages of phenotypes that resulted, eventually discovering the concept of dominance and recessiveness of characteristics. He also documented that there is no blending of inherited characteristics. For example, pea pod colors in the offspring of two parent plants, one with yellow pods and one with green, were either yellow or green, not yellowish green. Mendel also discovered that characteristics are inherited and expressed independently of each other, meaning the color of the pea pod was not necessarily expressed in conjunction with the pod being wrinkled or smooth. The recognition of the importance of Mendel’s work began with its rediscovery by Hugo de Vries and Carl Correns, both of whom were working on hypotheses regarding heredity in plants and had arrived at conclusions similar to Mendel’s. Both published papers supporting Mendel’s conclusions in 1900 (Moore 1993). Research into the inheritance of characteristics continued through the next three decades, and by the close of the 1930s, no major scientific questions remained regarding the transmission of heredity through genes, although what genes did and what chemicals they were made of were still under investigation. The Modern Synthesis refers to the merging of Mendelian genetics with Darwinian evolution that took place between 1930 and 1950. The basic principles of the synthetic theory were influenced by scientists working in many different fields, including genetics, zoology, biology, paleontology, botany, and statistics. Although there were differences of opinion among them, evolution came to be defined as changes in allele frequencies within populations. Genetic mutations, changes in the genetic code that are the original source of variation in every living thing, were believed to be random, the sources of phenotypic variation, and transmitted through sexual reproduction. These assertions were supported by a growing body of field and laboratory research, as well as new work in mathematics in the field of population genetics that defined evolution as numerical changes in gene frequencies within an interbreeding population from one generation to the next (Corning 2020). These changes in gene frequencies were argued to be the result of natural selection, mutation, migration (gene flow), and genetic drift, or random chance. Empirical research and mathematics demonstrated that very small selective forces acting over a relatively long time were able to generate substantial evolutionary change, including speciation (Plutynski 2009). Thus, the Modern Synthesis encompassed both microevolution, which refers to changes in gene frequencies between generations within a population, and macroevolution, longer-term changes in a population that can eventually result in speciation, wherein individuals from different populations are no longer able to successfully interbreed and produce viable offspring. Genetics and the Origin of Species, published in 1937 by Theodosius Dobzhansky (Figure 2.13), was a cornerstone of the modern synthesis, applying genetics to the study of natural selection in wild populations, appealing to both geneticists and field biologists. Dobzhansky was interested in speciation, particularly in finding out what kept one species distinct from another and how speciation occurred. His research involved fruit flies, the species Drosophila pseudoobscura. At the time he began in the 1920s, biologists assumed all members of the same species had nearly identical genes. Dobzhansky traveled from Canada to Mexico capturing wild members of D.pseudoobscura, discovering that different populations had different combinations of alleles (forms of a gene) that distinguished them from other populations, even though they were Figure 2.13: Theodosius Dobzhansky all members of the same species. What, then, led to the creation of (1943). Credit: Dobzhansky no Brasil em 1943 by unknown creator via Cely new species? Dobzhansky realized it was sexual selection. Members Carmo at Flickr is in the public of the same species are more likely to live among their own kind and domain. to recognize, and prefer, them as mates. Over time, as a result of random mutations, natural selection in a given environment, and genetic drift, meaning random changes in allele frequencies, members of the same population accumulate mutations distinct to their own gene pool, eventually becoming genetically distinct from other populations. What this means is that they have become a new species. From these studies, Dobzhansky and others developed the Bateson-Dobzhansky-Muller model, also known as Dobzhansky-Muller model (Figure 2.14). It is a model of the evolution of genetic incompatibility. Combining genetics with natural selection, the model is important in understanding the role of reproductive isolation during speciation and the role of natural selection in bringing it about. Due to sexual selection (mate preference), populations can become reproductively isolated from one another. Eventually, novel mutations may arise and be selected for in one or both populations, rendering members of each genetically incompatible with the other, resulting in two distinct species. Figure 2.14: The Dobzhansky-Muller Model: In the ancestral population the genotype is AABB. When two populations become isolated from each other, new mutations can arise. In one population uppercase A evolves into lowercase a, and in the other uppercase B evolves into lowercase b. When the two populations hybridize, it is the first time a and b interact with each other. When these alleles are incompatible, they represent Dobzhansky-Muller incompatibilities. Credit: Bateson-Dobzhansky-Muller model by OrientationEB is under a CC BY-SA 4.0 License. Evolution and Natural Selection Observable Today Although this chapter primarily focuses on the past, it is important to remember that natural selection and evolution are still ongoing processes. Climate change, deforestation, urbanization, and other human impacts on the planet are influencing evolution among many contemporary species of plants and animals. One such example occurs among crested anoles (Anolis cristatellus), small lizards of the Caribbean jungle that are increasingly making their home in cities (Figure 2.15). Figure 2.15: Puerto Rican Crested Anole photographed in Picard, Dominica. Credit: As urban sprawl continues across the planet, shrinking the Anolis cristatellus in Picard, Dominica-2012 02 availability of wilderness habitat, many wild species have 15 0339 by Postdif is under a CC BY-SA 3.0 come to make their homes in cities. “Urbanization has Unported License. dramatically transformed landscapes around the world— changing how animals interact with nature, creating “heat islands” with higher temperatures, and hurting local biodiversity. Yet many organisms survive and even thrive in these urban environments, taking advantage of new habitats created by humans (National Science Foundation 2023). A recent example of lizards in Puerto Rico demonstrates evolution happening quickly in both behavior and genes that has come about as a result of the pressures of urban life (Winchell et al. 2023). Crested anoles, who once lived only in forests, now scurry around towns and cities throughout the Caribbean. As a result of having to sprint across large open spaces, like hot streets and parking lots, they have developed longer limbs. City-living lizards also now sport longer toe pads with special scales that allow them to cling to smooth surfaces, like windows and walls (as well as the plastic patio furniture pictured in Figure 2.15), rather than to the rough surfaces of bark and rock that their forest-living relatives climb. These adaptations enhance their ability to escape predators and survive in cities. Researchers were curious to see if these changes were the result of genetic changes in urban populations, so they captured 96 male lizards in three Puerto Rican regions and compared their genomes to each other and to forest specimens in each location. They found that members of the three city-living populations were genetically distinct from each other, as well as from forest populations in their respective regions. In total, 33 genes in the urban lizards’ genomes were different from their forest-living counterparts and were linked to urbanization. These changes are estimated to have occurred just within the last 30 to 80 generations, suggesting that selective pressures related to survival in urban environments is strong. As study coauthor Kristen Winchell put it, “We are watching evolution as it is unfolding” (National Public Radio 2023). (If you are interested in hearing more about the study, see “How Lizards Adapt to Urban Living,” an episode of Science Sessions, a free podcast from the Proceedings of the National Academy of Sciences (PNAS 2023) featuring Dr. Winchell and her work.) Misconceptions About Evolution Through Natural Selection After many years of teaching about evolution and natural selection, it continues to surprise me how many misconceptions exist about how the process works. If you do a web image search for “human evolution,” the following image is likely to appear (Figure 2.16). Figure 2.16: An artist’s visual representation of the process of human evolution. Credit: Human evolution scheme by M. Garde is under a CC BY-SA 3.0 License. What is wrong with this picture? First, it implies that humans evolved from chimpanzees, which is incorrect. Although, as primates, we share a common ancestor very far back in time, we split from other primates, including our closest relatives, the nonhuman apes, several million years ago. This image also suggests that evolution is gradual and progressive; that it is intentional and directional; and that there is an end to it—a stopping point. As you will be learning, evolution takes place in fits and starts, depending on the physical environment, changes in climate, food supply, predation, reproductive success, and other factors. It is also not intentional, in the sense that there is no predetermined end; in fact, if environmental conditions change, species can evolve in different directions or even go extinct. Evolution also does not necessarily progress in the same direction over time. One example is the eel-like creature Qikiqtania wakei that lived 375 million years ago. It was originally a fish that evolved to walk on land, then evolved to live back in the water. Early tetrapods like Qikiqtania were likely spending more and more time out of the water during this period. The arrangement of bones and joints in their fins was starting to resemble arms and legs, which would have allowed them to prop themselves up in shallow water and survive on mudflats. Qikiqtania’s skeletal morphology, however, suggests that it then evolved from having rudimentary fingers and toes back to fins that allowed them to again swim in open water (Stewart et al. 2022). There is also the misperception that natural selection can create entirely new anatomical structures out of thin air in response to changes in environmental pressures. For example, when asked if they can think of ways in which modern humans are continuing to evolve biologically, students often postulate that, as a result of climate change, humans might rapidly develop gills, webbed hands and feet, and learn to breathe underwater in response to rising sea levels. Unfortunately, natural selection can only act on slight variations in anatomy that are already present, and we have no rudimentary physiological system for breathing underwater. Given that natural selection can only act upon existing variation, humans have evolved in such a way that many parts of our bodies are prone to injury. Our knees are one example. The anterior cruciate ligament (ACL) in our knees is “vulnerable to tearing in humans because our upright bipedal posture forces it to endure much more strain than it is designed to” (Lents 2018, 23). When our ancestors made the transition from quadrupedalism to upright walking, we shifted from four bent legs to two straight legs, relying more on our bones than our muscles to support our weight. This is functional for normal walking and running in a straight line, but sudden shifts in direction and momentum, combined with the sizes and weights of modern humans, result in tears in an ACL that is simply not strong enough to bear the stress. If evolution had the capability to engineer a knee from scratch, it would look quite different, and any ligaments involved would likely be larger, stronger, and more flexible. For an interesting look at what anatomically modern humans might look like if we had evolved to withstand the stresses our bodies undergo in our present environment, see “This is what the perfect body looks like – according to science,” which was proposed by biological anthropologist Alice Roberts (Harrison 2018). Another misperception about evolution is that some species are “more evolved” than others. Every species currently alive on the planet today is the result of millennia of natural selection that has rendered current members of that species well-adapted to their respective environments. Humans are no more “evolved” than fruit flies or yeast. What sets us apart are our cultural and technological abilities, which have allowed us to successfully survive in a wide variety of physical environments, many of which are now becoming too hot, too wet, or too dry to sustain human life without a great deal of technological intervention (IPCC 2022). There is also some confusion about what “fitness” actually means and a failure to grasp that it changes as environmental conditions change. Evolutionary “fitness” is different from physical fitness. “Fitness” in evolutionary terms refers to an individual’s ability to survive and reproduce viable offspring who also survive and reproduce. Evolutionary fitness and reproductive success are highly dependent on specific environmental conditions, which can shift over time, greatly affecting the relative fitness of individuals in a population. Recent research on the impacts of climate change on Figure 2.17: Adult male Common Whitetail dragonflies will serve to illustrate the point (Figure 2.17). Dragonfly, Libellula lydia. Credit: Common Whitetail Dragonfly – Plathemis lydia by Bruce Pictured here is a male dragonfly, who, you will notice, Marlin is under a CC BY-SA 2.5 License. has distinctive black markings on its wings. This is due to melanization. Males control breeding, and those with more ornamentation tend to attract more mates and to successfully ward off male competitors. Higher levels of melanization, however, have negative consequences for males in warming climates. The black markings absorb heat, elevating body temperatures, which can cause overheating, reduce male fighting ability, and even lead to death (Moore et al. 2021). Females are not as adversely affected because they spend more time in shaded areas, while males are more often flying in sunlit areas, fending off rivals. However, as highly melanized males become less viable, wing coloration is undergoing selection in males. In other words, what constitutes being “fit” for males has changed, favoring those who have fewer of the black markings and, therefore, are less negatively impacted by warming temperatures. Note that natural selection acts on individuals, “selecting” those who happen to be fit for particular environmental conditions at a particular point in time. Evolution, though, happens at the level of the population. If the climate continues to warm, populations of dragonflies who inhabit warming areas will increasingly exhibit less ornamentation in males. Lastly, natural selection can only act on characteristics that influence reproductive success. Deleterious traits that have nothing to do with one’s ability to reproduce and successfully rear offspring to reproductive age will continue to be passed on. For example, the author of this chapter is a natural redhead, and redheads are predisposed genetically to a number of conditions that can negatively affect health (Colliss Harvey 2015), but some of these conditions are not diagnosed until later in life. One example is Parkinson’s disease (Chen et al. 2017), which is a degenerative neurological disorder. The average age of diagnosis of Parkinson’s is 60 years of age, meaning redheads may encounter such a diagnosis well past childbearing age, having already passed on the genetic predisposition. Thus, Parkinson’s disease cannot be selected out from the redhead family tree. Dig Deeper: Teaching Evolution Around the World Evolution is recognized as a central organizing principle for all scientific disciplines and accepted without controversy among scientists and educated people around the world. The United States has historically been the exception (Lerner 2000). In some parts of the U.S. the teaching of evolution to K-12 students continues to evoke controversy, related to politics and religion. The problem is compounded by the degree of control individual states and local school boards exercise over curriculum in the nation’s public schools (Lerner 2000). The debate over teaching evolution in schools in the United States first came to a head