Mod 3. Gastrointestinal System PDF
Document Details
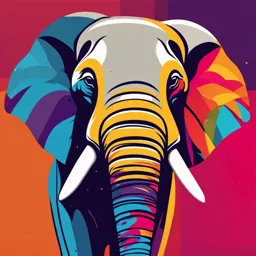
Uploaded by DependableMachuPicchu
Weber State University
Tags
Summary
This document provides an overview of the gastrointestinal (GI) system, including the digestive process, anatomy of the GI tract, mechanical and chemical digestion, and the role of accessory organs in digestion. It covers topics such as bolus, chyme, and peristalsis.
Full Transcript
3.1 The Gastrointestinal System Introduction Because eating is such a natural process, digesting food may also appear simple. However, many chemical, mechanical, physiological, and psychological factors are involved in the process. Nourishing the body begins with the ingestion of food and ends with...
3.1 The Gastrointestinal System Introduction Because eating is such a natural process, digesting food may also appear simple. However, many chemical, mechanical, physiological, and psychological factors are involved in the process. Nourishing the body begins with the ingestion of food and ends with the excretion of waste. The ultimate purpose of food intake is to fuel and nourish the body optimally. In order to extract any nutrients or Calories from food, the food has to undergo some significant alterations. These alterations take place in the digestive, or gastrointestinal, (GI) system and affect all of the other organ systems in the body in some way (see Figure 3.1). , , An Overview of the Digestive Process Let’s take a closer look at the anatomy of the digestive tract. We will focus on the steps in the metabolic processing of foods and their nutrients. Specifically, we will explore ingestion, digestion, absorption, transportation, utilization, cellular storage, and excretion. Once food has been chewed up, it is referred to as bolus. The bolus is swallowed, passes through the esophagus, and enters the stomach, where it is mixed with stomach acid, or gastric acid, which is largely hydrochloric acid, and other gastric secretions. As the smooth muscles around the stomach contract, the gastric contents are churned. This slushy mixture is called chyme. Food has to be systematically digested into its molecular components before absorption can occur. After digestion has taken place—that is, the large molecules that make up food have been broken down into molecules or chemical compounds small enough to enter the intestinal cells—the nutrients are absorbed into the bloodstream or the lymphatic system, transported to the various cells, taken up by the various cells making up the body systems, and metabolized. The solid waste that remains in the GI tract is compacted in the large intestine by removing the water. As the water is absorbed into the body, the solid waste product known as feces is formed and excreted. The cellular waste generated by metabolism is secreted back into the bloodstream and excreted via the respiratory system (in gaseous emissions containing carbon dioxide), urinary system (in aqueous secretions called urine), and the integumentary system (in aqueous and sebaceous secretions from the skin). So you can see that each step of the process plays a part in systematically dismantling the food into its nutrient components, metabolizing them, and excreting the waste (see Figure 3.2). Figure 3.2 The steps of digestion from intake to excretion. © 2016 Cengage Learning Mechanical and Chemical Digestion The mechanical and chemical processes that participate in digestion efficiently break down the large food molecules into their molecular units. For example, 98 percent of carbohydrates, including starch (polysaccharides), lactose, maltose, and sucrose, is digested; 95 percent of fats (triglycerides) is digested; and 92 percent of proteins (plant and animal) is digested. This efficient digestive system accomplishes digestion by coordinating the actions of many organs that contribute the needed chemical and mechanical processes. Mechanical Digestion The part of the digestive process that is mechanical uses muscles and nerves to create movement. Examples include chewing and muscular stomach contractions that mix and churn the chyme. Another mechanical process is peristalsis, a muscular wave action that is caused by smooth-muscle contractions in the digestive system. Peristalsis is controlled by the autonomic nervous system. It begins with swallowing, then propels bolus (chyme) along the GI tract. It is an automatic process, meaning it happens without you consciously thinking about it. In contrast, the ingestion, mastication (chewing), and swallowing of food are conscious processes. Chemical Digestion Chemical digestion refers to the use of chemical substances that are needed to break down food, such as enzymes, hormones, hydrochloric acid, and sodium bicarbonate. Chemical digestion, like peristalsis, is controlled unconsciously by the autonomic nervous system. Approximately 7.5 liters of digestive juices are secreted into the GI tract over the course of the day. Think of almost four 2-liter bottles of fluids, such as saliva, mucus, gastric juice, intestinal cell secretions, bile, and pancreatic secretions, pouring into the GI tract. Let’s take a closer look at the GI system’s anatomy. We will focus on the steps in the metabolic processing of foods and their nutrients. Specifically, we will explore ingestion, digestion, absorption, transportation, utilization, cellular storage, and excretion of food and food components. Anatomy of the Gastrointestinal System The GI tract is a tube like structure (often called the alimentary canal) that runs through the body, including the mouth, esophagus, stomach, small intestine, large intestine, colon and rectum (see Figure 3.3). The accessory organs of the GI system, though not part of the tubular passage, are directly involved in digestion. These accessory organs produce and/or secrete chemicals into the GI tract that facilitate the digestion of chyme into its molecular components. The accessory organs of digestion include the liver, gallbladder, and pancreas (see Figure 3.3). The accessory organs are signaled to participate in digestion by many different hormones. A select few of those hormones are discussed later in this module. Figure 3.3 The anatomy of the gastrointestinal (GI) tract. © 2016 Cengage Learning Journey Through the GI Tract The passage that food and drink make through the GI tract is long and winding, with many changes in the physiological environments. Let’s make the journey one segment at a time. Footnote The Mouth After food enters the mouth or ingested, it immediately begins to be altered. In the mouth, mechanical digestion and some chemical digestion occur. Mechanical digestion occurs with mastication—the grinding and chewing of food. Chemical digestion occurs from the enzymes in saliva. Approximately 1.5 liters of saliva are produced each day by the salivary glands. This saliva is composed largely of water to moisten food. The enzyme salivary amylase digests starch. After partial digestion of food occurs in the mouth, the food substance is called a bolus and is swallowed. The Esophagus Once the bolus is swallowed, it enters the portion of the tube-like passage called the esophagus. From there, it is propelled to the stomach by the action of peristalsis (see BioBeat 3.1). The Stomach The stomach is a large sac-like organ that plays an important role in digestion. A bizarre balance is maintained in this unique organ: The stomach has cells of the glandular epithelium (see Figure 3.4) that release hydrochloric acid and cells that release thick mucus that protects other stomach cells from the damaging acid. Further, there are three layers of muscle cells: horizontal, vertical, and oblique (see Figure 3.4). The bolus that has passed from the esophagus turns into a liquefied slurry as it is mixed and churned with all the gastric juices. Approximately 2 liters of gastric juice, composed of hydrochloric acid, enzymes, hormones, and mucus, are produced and used each day. The hydrochloric acid plays a big role in the chemical digestion of food. The acid denatures proteins and prepares chyme for enzymatic action. Some enzymes that digest protein, such as the protease enzyme pepsin, do their work in the stomach. The stomach also serves as a food reservoir and controls the slow release of chyme from the stomach into the small intestine. The release of small amounts of chyme is controlled by the autonomic nervous system and hormones (see BioBeats 3.1 and 3.2). Having small amounts of chyme entering the small intestine increases the efficiency of chemical digestion there. Figure 3.4 The musculature of the stomach. A diagram shows the musculature of the stomach. The illustration shows a crescent-shaped stomach connected to a tube like passage at top and bottom labeled esophagus and small intestine respectively. Sphincter is present between stomach and esophagus and stomach and small intestine. The bottom of stomach is filled by glandular epithelium which is further followed by few layers forming a V-shaped depression that represents longitudinal, horizontal, and oblique smooth-muscle layers. © 2016 Cengage Learning The Small Intestine The small intestine includes the duodenum, jejunum, and ileum. More digestion occurs in the small intestine, particularly in the duodenum, than in other segments of the intestine. Here, about 2 liters of intestinal juices per day—composed largely of mucus, enzymes, and hormones—are released to aid digestion. Some juices are released when the accessory organs—liver, gallbladder, and pancreas—are signaled. After digestion is complete, absorption of many different nutrients, such as monosaccharides, amino acids, fatty acids, vitamins, and minerals, must occur in order to deliver these essential nutrients to body cells. All the while, peristalsis continues to take place, propelling chyme and sloughed-off intestinal cells into the large intestine. BioBeat 3.1 Digestion and the Nervous System Much of the digestive process is controlled through the autonomic nervous system. Once you have chewed your food, you do not have to think about the chemical or mechanical actions of digestion; they are controlled automatically for the most part. Smooth muscles that line the GI tract wall contract and relax to propel the intestinal contents through the body. These muscles contract and relax without conscious guidance. The autonomic action that moves food along the entire GI tract is called peristalsis. The major concert of automatically controlled chemical secretions and actions begins in the stomach and continues through the first segment of the small intestine. Additionally, the release of hormones solicits the accessory organs’ fluids for further chemical digestion, including the bile that is produced by the liver and stored in the gallbladder, as well as pancreatic juices. The pancreatic juices contain many digestive enzymes plus sodium bicarbonate to neutralize gastric acid. The significant amount of chemical digestion that occurs in the small intestine produces smaller molecular components that are absorbed primarily in the first segment of the small intestine. Which parts of digestion are under your conscious control? Let’s take a deeper look at the accessory organs, digestion, absorption, transportation, utilization, and storage of nutrients before venturing into the large intestine and discussing excretion. Accessory Organs Involved in Aiding Digestion at the Duodenum Three accessory organs contribute to digestion: the liver, gallbladder, and pancreas (see Figure 3.5). The liver and the pancreas perform many vital functions for the entire body, some of which will be discussed later. For now we will focus on their roles in digestion. The liver produces approximately 0.5 liter of bile each day. Bile is composed largely of cholesterol-rich bile acids. The gallbladder stores the bile that is produced by the liver. The gallbladder’s sole role in the body is to store bile acids, which are used to aid fat digestion. Bile is released from the gallbladder when bound with the hormone CCK, causing the gallbladder to contract. The purpose of bile is to emulsify fat in the small intestine. The pancreas produces approximately 1.5 liters of pancreatic juice each day and secretes the juice containing enzymes for carbohydrate, protein, and fat digestion, as well as sodium bicarbonate for the neutralization of acidic chyme into the small intestine. Figure 3.5 The liver, gallbladder, and pancreas are the accessory organs that aid digestion. A two-part diagram shows accessory organs that aid digestion including liver, gallbladder, and pancreas. The first part of the diagram shows a human body outline with digestive system. The second part of the diagram shows a zoomed in image of organs responsible for chemical digestion. The diagram shows a cap like structure representing liver which is connected to crescent-shaped stomach on the right and pear shaped gallbladder on the left. A capillary tube-like bile duct from liver enters into flat pear shaped pancreas passing through C-shaped duodenum on the left and below the gallbladder. The bile duct further meets duct from pancreas and the layer surrounding these ducts show exocrine cells secreting pancreatic enzymes. Several cells collectively forming flower like structure represent endocrine cells secreting insulin and glucagon into bloodstream.Enlarge Image © 2016 Cengage Learning BioBeat 3.2 Coordinating Digestion: Hormones as Chemical Messengers A hormone is a chemical messenger produced and secreted from cells within endocrine glands or organs and released into the blood. Hormones specifically influence the processes within certain cells of the body in order to regulate metabolism. Some hormones coordinate the digestive processes. For example, hormones like gastrin, secretin, and cholecystokinin (CCK) are involved in chemical digestion. Gastrin stimulates the secretion of gastric juice. Secretin is released from duodenal cells and signals the pancreas to secrete pancreatic juice (made of digestive enzymes) and sodium bicarbonate and the liver to produce bile. Cholecystokinin is released from duodenal cells in response to the presence of fat-rich chyme. Cholecystokinin signals pancreatic secretions and the gallbladder to release bile. Footnote Can you create an analogy for the job of a hormone in a practical everyday situation? Digestion in the Small Intestine Fat digestion: Fat must be emulsified by bile that is produced by the liver and stored in the gallbladder (see Figure 3.6). When the gallbladder receives a signal to contract from CCK, bile is released into the duodenum. Bile allows fat to interact with fat-splitting lipase enzymes. Once fat is emulsified by bile and partially digested into glycerol, fatty acids, and/or monoglycerides by lipase, the tiny fat droplets are absorbed by the intestinal villi (epithelial cells) in the small intestine. Inside the cells, the fat droplets are packaged into chylomicrons (a temporary lipid-carrying protein). Chylomicrons are then released into the lymphatic system and later into the bloodstream. The chylomicrons are taken up by the liver and repackaged into usable lipoproteins, which are released again into the bloodstream for delivery to body cells. Figure 3.6 The emulsification of fat by bile. A three-part diagram labeled (A) through (C) shows the emulsification of fat by bile. Part (A) shows a container filled with water with a layer of fat on top. The water layer contains several C shaped structure representing enzymes. A text below reads: Fats and water tend to separate; enzymes are in the water and can’t get at the fat. Part (B) shows some other smaller C shaped structures representing bile. Few of this bile are attached with fat at the top. A text below reads: Bile (emulsifier) has affinity for fats and for water so it can bring them together. Part (C) shows enzymes inside water forming complex with emulsified fat. These emulsified fat are result of fat and bile complex. A text below reads: Small droplets of emulsified fat. The enzymes now have access to the fat, which is mixed in the water solution. © 2016 Cengage Learning Carbohydrate digestion: Salivary and pancreatic amylase enzymes cleave the alpha bonds in starch to release glucose. In the small intestine, maltase, sucrase, and lactase break down maltose, sucrose, and lactose, respectively. The monosaccharides and carbohydrate remnants are then absorbed into intestinal villi and then released into the bloodstream for delivery to body cells. Protein digestion: Proteins are broken into their amino acid constituents by protease enzymes, some of which are produced in the stomach and some in intestinal cells. The pancreatic cells produce large amounts of other protease enzymes. All protease enzymes break the peptide bonds that join amino acids together in proteins. Ultimately, amino acids are released. Amino acids are absorbed into intestinal villi and then released into the bloodstream. Vitamins and minerals: Vitamins and minerals are micronutrients that need to be released from food by digestion so they can be absorbed into the body via the intestinal villi epithelial cells. Unlike carbohydrate, protein, and fat, vitamins and minerals do not undergo digestion. Instead, vitamins and minerals are absorbed through a variety of mechanisms—many specific to the particular nutrients. Absorption of Fat Watch the animation illustrating how fat is absorbed into the bloodstream. Copyright © Cengage Learning. All Rights Reserved Protein Digestion in the GI Tract Watch the animation about how protein is digested in the GI Tract. Copyright © Cengage Learning. All Rights Reserved Mechanisms and Sites of Absorption in the Small Intestine The passage of digested food (nutrients) from the GI tract into the body is called absorption (see BioBeat 3.3). The small intestine surface anatomy includes the villi and microvilli (see Figure 3.7). The villi of the small intestinal wall are finger like projections that add an additional surface layer of more epithelium made by microvilli (also called the brush border). The microvilli significantly increase the surface area (one-third the size of a football field in an adult human) to enhance nutrient absorption. Figure 3.7 The anatomy of the small intestine. A set of 5 diagrams shows the anatomy of the small intestine. The first part of the diagram shows a crescent shaped stomach connected to pipe like small intestine at the bottom. The second part of the diagram shows a zoomed in image of the inner wall of the small intestine composed of few folds covered with finger like structure that represent villi. Muscle layers are located beneath the folds. A text to the left reads: The wall of the small intestine is wrinkled into thousands of folds and is carpeted with villi. The third part of the diagram shows a zoomed in microscopic image of wall of the small intestine. At the bottom of the image three thin pipe-like structures are placed beneath the folds including lymphatic vessel, vein, and artery from bottom to top respectively. The lymphatic vessel passes through the center of the folds and on the left of the vessel a pipe represents capillary. These folds are surrounded by bunch of villi and its single unit is a villus. A text pointing towards the hollow space between the folds reads: Between the villi, tubular glands secrete enzyme-containing intestinal juice. The fourth part of the diagram shows zoomed in image of three cells of a villus. Each cell is covered with microvilli at the top. Cell organs including mitochondria, Golgi complex, and nucleus are shown inside the cells. The fifth part of the diagram shows a photograph depicting a part of a human intestinal cell with microvilli.Enlarge Image © 2016 Cengage Learning Nutrient absorption takes place mostly in the duodenum and then the jejunum, and for some nutrients, in the ileum. The villi are supplied with their own blood and lymphatic vessels, so the nutrients can be absorbed and released into the body. Nutrients released by digestion are absorbed from the small intestine cavity, or lumen, into intestinal epithelial cells, then either passed to the bloodstream (water-soluble nutrients) or lymphatic vessels (fat-soluble nutrients). Eventually, the fat-soluble nutrients will be released into the blood stream. Once the nutrients are in the blood, they can be transported to all the various cells of the body. No matter if the nutrient is entering the microvilli, blood, lymph, or a cell, one of the three most common mechanisms of nutrient absorption—passive diffusion, facilitated diffusion, or active transport—is usually used (see Figure 3.8). Each nutrient, for the most part, specifically uses one of these transport mechanisms to enter or exit a cell: Passive diffusion: This transport mechanism is sometimes called simple diffusion, passive transport, or simple transport. It allows nutrients to cross cell membranes freely from the intestinal lumen into cells by a concentration gradient created by osmotic pressure. This means that the higher concentration of nutrients on one side of the membrane creates a pressure to force the nutrients across the membrane to the lower concentration (see Figure 3.8). After food intake, there are higher concentrations of nutrients in the intestinal lumen compared with the inside of intestinal cells, so the nutrients are driven into villi. Once the nutrients are in the villi, they will be released into the blood or lymphatic system, depending on their solubility, for delivery and use by body cells. Facilitated diffusion: This transport mechanism is sometimes called facilitated transport. The nutrient using this mechanism requires a specific carrier protein or receptor protein in order to cross the cell membrane. Therefore, it is a selective transport or absorption process. Nutrients that require facilitated diffusion bind to a specific receptor protein. The receptor protein is designed to selectively bind to the nutrient before the nutrient can gain entry into the cell. Once the nutrient is assisted into the villi by the receptor protein, it will be released into the blood or lymphatic system, depending on its solubility, for delivery and use by body cells. This mechanism of absorption allows better regulations for nutrient concentrations that enter the body. Active transport: This transport mechanism requires a selective carrier protein or receptor protein and adenosine triphosphate (ATP; energy) to cross the cell membrane. Therefore, it is a highly selective absorption process. Nutrients that require active transport bind to a specific receptor protein and can cross the cell membrane only if ATP is used. The receptor protein is designed to selectively bind to the nutrient, and the ATP is needed to allow the nutrient to get into the cell while opposing a concentration gradient. The combination of the receptor protein and the ATP is the only way for the nutrient to gain entry into the cell. Once the nutrient is assisted into the villi by the receptor protein and ATP, it will be released into the blood or lymphatic system, depending on its solubility, for delivery and use by body cells. This mechanism of absorption governs nutrient entry into the body at the highest level. Figure 3.8 The fundamental mechanisms of nutrient absorption across a cell membrane. A diagram shows the fundamental mechanisms of nutrient absorption across a cell membrane. The diagram shows three types of diffusion across a lipid bilayer. On the left vertical axis of diagram a concentration gradient is present that ranges from high to low from top to bottom. In the passive diffusion, some spherical nutrients cross lipid membrane from area of high concentration to area of low concentration. In the facilitated diffusion, nutrients cross cell membranes by binding to a specific carrier protein embedded inside the lipid membrane from area of high concentration to area of low concentration. In the active transport, nutrients cross cell membranes by binding to a specific carrier protein attached with A T P from area of low concentration to area of high concentration.Enlarge Image © 2016 Cengage Learning Pop-Up Tutor: Absorption in the Small Intestine Watch this tutorial video to increase your understanding of absorption in the small intestine. Copyright © Cengage Learning. All Rights Reserved. BioBeat 3.3 Nutrient Absorption in the Small Intestine When nutrients (vitamins, minerals, and breakdown products of carbohydrates, proteins, and fats from digestion) are absorbed into the body, the nutrients must pass from the inside of the gastrointestinal tract lumen into the epithelial cells in the small intestine villi. They pass into these cells by traveling through semipermeable cellular membranes. The three major mechanisms in which absorption occurs—passive diffusion, facilitated diffusion, and active transport—are involved in absorption of nutrients into the cells of the body or the transport across any cell membrane. For example, water and fatty acids are absorbed by passive diffusion, whereas facilitated diffusion is the usual mechanism needed to absorb fructose, riboflavin, and vitamin , and active transport is the usual mechanism needed to absorb glucose, amino acids, calcium, iron, and iodine. Protein carriers can speed up the absorption process. For example, the rate at which glucose enters a cell is 500 times slower with passive diffusion than with active transport. After nutrient absorption takes place by the small intestinal cells, the cells pass the nutrients directly (or after packaging/processing) into either the blood vessels for water-soluble nutrients or lymphatic vessels for fat-soluble nutrients. Can you relate the concept of diffusion and transport to your daily activity? Nutrient Transport Through the Body Pop-Up Tutor: Circulation Listen to Dr. Jessica Coppola discuss Circulation. Copyright © Cengage Learning. All Rights Reserved. The movement of nutrients throughout the body requires circulatory transport. There are two transportation (transport) systems: the blood and the lymph. The bloodstream provides the transport system for water-soluble nutrients, and the blood is the medium for transport. The lymph system provides the transport for fat-soluble nutrients. Lymph fluid fills the lymphatic vessels and flows through them with the help of the pumping action of the heart and the pulses of the cardiovascular system. The lymph is filtered through lymph nodes and the spleen, but the fat-soluble nutrients are eventually released into the bloodstream at the interface of a fast-flowing blood vessel. The lymphatic system is the initial transport system for fat-soluble nutrients, and the lymph fluid is the medium for transport. Utilization and Storage Inside the Body After nutrients are digested, absorbed, and transported inside the body to cells, they are used in metabolism for ATP production, growth, repair, and storage (see BioBeat 3.4). The type of metabolic reactions that the nutrient participates in could be anabolic, catabolic, or transfer (neither anabolic nor catabolic). If the nutrient is not needed for one of these purposes, it can be stored. The storage of nutrients in the body may be short, intermediate, or long-term as follows: Short-term storage: The substance is stored for seconds or minutes. An example is ATP in all cells. Intermediate storage: The substance is stored for hours. An example is glycogen in the liver. Long-term storage: The substance is stored for days, months, or years. An example is fat in adipose tissue; vitamin A and vitamin in the liver; iron in the liver and bone marrow; protein in lean body mass; and calcium, phosphorus, and magnesium in bones. The Large Intestine The journey through the GI tract ends with the passage of waste through the large intestine, which is located just after the ileum in the small intestine. Here the surface cellular anatomy changes from villi and microvilli to cells designed to absorb water, so the compaction of waste and absorption of water occur in prepararation for excretion. The large intestine includes the cecum (the appendix, a small finger like extension, sticks out from this structure); colon (ascending, transverse, and descending); rectum; and anal canal (anus). The large intestine can also be called the large bowel or colon. BioBeat 3.4 Anabolic and Catabolic Reactions Metabolism involves a constant process of chemical reactions, some of which are constructive in nature, whereas others are destructive. Homeostasis maintains a balanced state between building (anabolism) and destroying (catabolism) reactions. Anabolic, or building, processes are involved in growth, repair, synthesis, and repletion of tissues. A typical chemical reaction involved in anabolism is a condensation reaction, in which molecules are joined together as a chemical substance is built up (see Figure 3.9). In a condensation reaction, hydrogen and oxygen atoms are no longer needed, so they are condensed into water when the molecules are joined. Two examples of anabolic reactions are the combining of individual amino acids to make a protein and the combining of glucose units to make glycogen. On the other hand, catabolism is the breakdown of complex organic molecules into simpler components, accompanied by the release of energy (ATP). Catabolic processes occur in energy metabolism, stress, and tissue degradation. A typical chemical reaction involved in catabolism is a hydrolysis reaction: When the molecules of a chemical substance are broken apart, water is needed (see Figure 3.9). The water is split apart into atoms of hydrogen and oxygen, which are used to balance the chemical fragments resulting from the catabolic reaction. Two examples of catabolic reactions are breaking peptide bonds in a protein to release the individual amino acids and releasing glucose units from glycogen. How does your body remain relatively the same in the midst of ongoing catabolism and anabolism in the dynamics of life? Figure 3.9 Condensation and hydrolysis reactions. A set of two diagrams shows condensation and hydrolysis reactions. Diagram (A) shows two phenol groups. One with O H group attached at C 1 and other with O H group attached at C 4 and they are placed adjacent to each other. The O H group of the phenol on the left reacts with H of the phenol on the right. A text below reads: Enzyme action at functional groups. Both the phenols are connected with O in the middle and releasing a water molecule or H 2 O. A text at the bottom reads: Condensation. An –O H group from one molecule combines with an H atom from another. Water forms as the two molecules bond covalently. Diagram (B) shows two six membered rings connected via an O. An H 2 O is being added to the above atom and a text below reads: Enzyme action at functional groups. The addition of an H 2 O group results in two separate phenols. A text below the diagram reads: Hydrolysis. A molecule splits, and then an O H group and an H atom from a water molecule become attached to sites exposed by the reaction. © 2016 Cengage Learning Excretion The start of the journey through the GI tract was the result of a conscious decision for food ingestion, and then the unconscious process of peristalsis took over after swallowing to propel food through the tract. Now elimination occurs by conscious action through the circular anal sphincter. When the journey through the GI tract ends, a new one will surely begin as the food processor known as the gastrointestinal tract continues to function making the substances in food available for cellular metabolism (see Table 3.1 for a summary). Waste products and by-products from cellular metabolism and the digestive process are eliminated from the body by excretion through one of four methods: feces, urinary output, respiration, and skin secretions. Table 3.1 Highlights of Digestion Site Mechanical ProcessChemical Process Accessory Organs Absorption Along the GI tract Uses nerves and muscles Uses hormones, enzymes, digestive juices, and acids Aids digestion outside the GI tractSubstance is taken up by the body Mouth Mastication Amylase (Footnote) N/A N/A Esophagus Peristalsis N/A N/A N/A Stomach Mixing, churning HCl acid Pepsin (Footnote) Gastrin (Footnote) N/A Alcohol Small intestine (duodenum, jejunum, ileum) Peristalsis Amylase (Footnote) Protease (Footnote) Lipase (Footnote) Cholecystokinin (Footnote) Secretin (Footnote) Bile Liver: Bile synthesis Gallbladder: Bile storage Pancreas: Enzymes and sodium bicarbonate Carbohydrates, proteins, fats, vitamins, minerals Large intestine Peristalsis N/A N/A Water Rectum Excretion N/A N/A N/AEnlarge Table © 2016 Cengage Learning Feces Feces are excreted as the solid waste products of the GI system. Solid waste is largely composed of; bacteria, fiber, sloughed-off intestinal epithelial cells, undigested food, and small amounts of GI tract juices. The speed at which food moves through the GI tract is based on meal size, dietary composition, and physiological factors and is referred to as transit time. Transit time from ingestion to excretion is typically 9 to 48 hours. Urinary Output Urinary output is the volume of urine excreted in a 24-hour period. Urine is an aqueous waste solution excreted from the urinary system, and produced by the kidneys. Urine contains water, nitrogen waste (mostly urea), detoxified chemicals (including medications), mineral salts, and excess or degraded vitamins and minerals. The most common mechanism for disposing cellular, chemical, metabolic waste is via the urine. Respiration Respiration is excretion of gaseous metabolic waste products by the pulmonary system, or lungs. Respired air has high concentrations of carbon dioxide and water vapor produced by cellular metabolism of carbohydrates, fats, and proteins. Skin Secretions Aqueous and sebaceous waste are excreted as either sensible or insensible secretions from the integumentary system, or skin. Insensible excretion produces small amounts of water, mineral salts, lipids, and amino acids. Sensible secretion is noticeable sweating resulting from heat, exercise, fever, or hormonal imbalance. Pop-Up Tutor: GI Tract: Overview Watch this tutorial video to increase your understanding of the GI tract. Copyright © Cengage Learning. All Rights Reserved. 3.2 Proteins: From Foods to Cellular Utilization in the Body Introduction Dietary protein provides the amino acids for many critical functions in the body. It is important to understand how the human body uses the amino acid building blocks provided from dietary protein to cells in order to synthesize their own proteins. Self-synthesized proteins (endogenous protein synthesis) are needed for body tissue repair and maintenance, and the basic structures of all cells in organs, muscles, the brain, nerves, skin, blood, hair, and nails, as well as regulatory components such as enzymes and hormones. The process of dietary protein denaturation and digestion is described next. Greater detail on protein is also provided with respect to protein character, types, deficiency, and excess., Protein Denaturation versus Digestion Proteins are three dimensional chemical structures made from strands of proteins. These strands of proteins are made from many amino acids bound together. Protein structure can be altered. Protein denaturation is one way to alter a protein and it is an important step in protein digestion. Protein denaturation causes the protein to change its three-dimensional structure as the coiled shape of the protein is loosened or modified (see Figure 3.10). Protein denaturation can be caused by treatment with heat, acid, and alkali, or exposure to heavy metals. If you have ever cooked an egg, you have seen protein denaturation in action in both the white and the yolk. Denaturation also occurs with exposure of protein to acid or alkali (basic) treatments, as when lemon juice is added to milk: The milk proteins curdle. Acid denaturation occurs unseen when a food protein encounters stomach acid. Exposure of proteins to metals, such as by cooking foods in cast-iron pots, causes denaturation too. After denaturation, the enzymes can more easily access the various parts of the protein strand to digest (break apart) the amino acids in the protein; therefore, the process of denaturation is important for digestion, because it better prepares dietary protein for enzymatic digestion. Figure 3.10 Protein denaturation and digestion. © 2016 Cengage Learning When a protein is digested, the peptide bonds between the amino acids in the protein strand are broken, and the amino acids are released. Protein digestion takes place in the stomach and small intestine through the action of protease enzymes. The amino acids that are released from digested proteins can be absorbed into intestinal villi cells and transported to the body cells via the blood stream. Inside body cells, the amino acids are ready to be incorporated into newly built or synthesized proteins based on the particular metabolic need of the cell at the time. Protein Synthesis It is important for each cell in the body to make specific proteins in order to repair cell structures and carry out many cellular chemical processes. Protein is synthesized in the body from amino acids according to the genetic code, which is housed within the cell nucleus as part of a chromosome, in a process that begins with gene expression. Gene expression is regulated by cell signals, such as by hormonal messages, based on the cellular environment. Basically, when a cell needs a particular protein product to perform a particular task, the gene for that protein is expressed. After gene expression occurs, the deoxyribonucleic acid (DNA) gene code is copied to a messenger ribonucleic acid (mRNA), which is then translated to make an amino acid sequence that creates the unique protein strand. The formed protein strand folds and interacts with itself and sometimes with other protein molecules to make a unique threedimensional shape and then performs its specific function. This process of making a functional protein, from copying DNA to RNA and then to creating a protein, is called protein synthesis (see BioBeat 3.5 and Figures 3.11 and 3.12). Figure 3.11 The cell houses the nucleus, where the chromosomes, with their DNA sequences, are located. Adapted from “A Primer: From DNA to Life,” Human Genome Project, U.S. Department of Energy Office of Science; http://www.ornl.gov/sci/techresources/Human_Genome/. Figure 3.12 Protein synthesis involves DNA transcription in the cell nucleus and then mRNA translation with ribosomes and tRNA in the cell cytosol. © 2016 Cengage Learning BioBeat 3.5 Protein Synthesis (DNA to mRNA to Protein) DNA and mRNA are nucleic acids. DNA is made of four nucleotide bases: adenine (A), cytosine (C), guanine (G), and thymine (T). These bases are organic compounds containing carbon, hydrogen, oxygen, nitrogen, and phosphorus. They are made of a nitrogenous base, five-carbon sugar, and phosphate constituents. They form into a double-stranded DNA helix that has sugar and phosphate units attached. From the double strand of the DNA, one strand forms the “sense” sequence, and the other strand forms the “antisense” sequence. In the DNA double helix, A always pairs with T, and C always pairs with G. For example: Sense Antisense A T T A G C C G DNA is converted to mRNA through a process called transcription. In mRNA, the nucleic acid uracil replaces thymine. Uracil pairs with adenine. Through a process called translation mRNA is used to order the amino acids in the protein. Typically, three base pairs of RNA are read at a time to encode an amino acid. For example, AAG calls for lysine. As the mRNA is translated, the amino acids are linked together, forming a polypeptide and eventually a protein. Once the protein is formed, it can perform its function within the cell. DNA, mRNA, and protein are considered biomolecules. What is the fate of the amino acids in the proteins you eat? The expressed gene in the DNA template is transcribed, or copied, to mRNA. This process is called transcription. Afterward, the mRNA leaves the cell nucleus and enters the cell cytosol. Here, the mRNA attaches to ribosomes (the site of protein synthesis), where the mRNA code is translated to an amino acid sequence. This process is called translation and is somewhat like code breaking. The RNA in the ribosome (ribosomal RNA, or rRNA) reads the code and interprets the meaning to a specific amino acid that is needed in the protein sequence. Transfer RNA (tRNA) collects the needed amino acids and delivers them to the ribosome. Amino acids are then linked together in a protein strand. The bonds between the amino acids are called peptide bonds. The order of the amino acids in a protein is determined by transcription and translation of genetic material (see Figure 3.12 and BioBeat 3.5). When the mRNA message is fully translated and the protein is synthesized, the mRNA is degraded and the tRNA and ribosomes are freed to make other proteins. Further, the protein may exist in the cell for only minutes and then be degraded after it has done its job. The amino acids from the degraded protein then reenter the cellular amino acid pool and are available to make other proteins. An example of hormonal control over gene expression and protein synthesis is the hormone testosterone’s response to exercise. Testosterone is present in both men and women, although it exists in higher concentrations in men. In response to exercise stress, increased amounts of testosterone bind to cell receptors and signal an increase in protein synthesis, resulting in increased muscle mass and enhanced muscular strength and size. Thus, the genes that encode cellular proteins are turned on, or expressed, after the testosterone hormone signal is received. Pop-Up Tutor: Protein Synthesis Listen as Professor Coppola reviews protein synthesis. Volume 90% Copyright © Cengage Learning. All Rights Reserved. Protein Character The human body uses 20 different amino acids to synthesize specific proteins for structure and function. Nine are essential amino acids. The remaining 11 are nonessential amino acids, because the body can make them from carbohydrate intermediates and nitrogen molecules. The body can acquire essential amino acids only from dietary protein sources. The 20 different amino acids making up proteins have different chemical properties due to their side chains that cause them to fold and interact with each other in unique ways to ultimately create a three-dimensional functional protein. These unique protein structures define the protein character, which is partly determined by the amino acid sequence in the protein. Proteins have many chemical functions as buffers, receptors, enzymes, and hormones. Their abilities to participate in their unique and numerous roles in the body depends on their three-dimensional structures. Proteins begin with a primary amino acid sequence that dictates each protein’s three-dimensional secondary, tertiary, and even quaternary structure (see Figure 3.13). Figure 3.13 The primary, secondary, tertiary, and quaternary structure of the protein hemoglobin, found in red blood cells. Hemoglobin contains the mineral iron in the heme group to bind oxygen and deliver it to all body cells. Hemoglobin is a globular protein; it is part random and part alpha-helix. © 2016 Cengage Learning Types of Protein To better understand the types of proteins that make up lean body mass and function in the body, it is important to know about protein types. There are two basic types of protein: fibrous and globular. A fibrous protein is uniform in its structure created from the way the amino acid side chains interact (fold) in the protein strand. Its formation is either exclusively helical or in a pleated sheet. This means that the amino acids in the polypeptide strand fold and interact chemically together to form a uniform threedimensional structure. Fibrous protein three-dimensional structures can look like the toy called a Slinky (alpha-helix) or an accordion (beta-sheet). Some examples of fibrous proteins are the proteins found in hair and fingernails. A globular protein has variation in its structure. It can be part helical, part sheet, part random, or completely random, meaning there are no consistent segments in the protein structures. Some examples of globular proteins in the body include proteins found in blood and mucus. Protein Functions Proteins and their amino acid building blocks are needed to build cellular proteins for many important body functions. The functions of protein include growth and maintenance of lean body mass (tissue turnover, maintenance, replacement, and deposition); creation of enzymes (chemical catalysts); creation of antibodies (immune agents); fluid and electrolyte balance (osmolarity); acid-base (pH) balance; creation of protein hormones like insulin and glucagon; nutrient transport proteins in the blood and lymphatic vessels (such as lipoproteins); and release of energy (4 Calories per gram) when the body is under stress. Recall, using amino acids for fuel requires nitrogen removal or deamination (removal of an amine group from an amino acid) and increases the synthesis of urea, a nitrogenous waste product. Each day, new protein needs to be consumed to replace the protein that was lost from the body in vital functions. Protein replacement is accomplished by the consumption of animal and plant proteins. Because most animal proteins have all the essential amino acids present in optimal amounts and ratios, they provide complete, high-quality, or high biological-value proteins. Plant proteins, on the other hand, provide incomplete, low-quality, or low biological-value proteins, because they are lacking one or more of the essential amino acids in their composition. The quality of the protein is only as good as the most limiting essential amino acid present in the protein. Egg white is the highest-quality natural food protein for humans, because the ratios of the essential amino acids in combination with all the amino acids present in the protein match human needs. When a protein is incomplete, it can be complemented with another incomplete protein to reach the equivalent of a complete protein. Complementary proteins occur by combining plant-based groups such as grains with legumes in such a way that the essential amino acids missing or limited in one protein are abundantly supplied by the other protein (see Module 1). Protein Recommendations Both complete and incomplete proteins provide needed amino acids and other nutrients for the body. The Dietary Reference Intake (DRI) for protein for adults is 0.8 gram per kilogram of body weight. However, there are times when more protein may be needed. For example, the body uses more protein while in anabolic states (such as during childhood growth, pregnancy, or body building and rigorous athletic training). The DRI for protein for infants, children, and adolescents is higher than that for grown adults (see Appendix A for values). For athletes needing muscle growth and/or tissue repair from bouts of exercise, approximately 1.2 to 1.6 grams per kilogram of body weight (high-quality or equivalent dietary protein) is needed to support repair of lean body mass. Endurance athletes can safely consume up to 1.8 to 2 grams per kilogram of protein due to extended exercise stress on the body. The values given for adult athletes are not DRIs. For all adults, the Acceptable Macronutrient Distribution Range (AMDR) for protein is 10 to 35 percent of total Calories. However, when the dietary intake of protein exceeds twice the DRI amount of an individual, it is considered a high-protein diet and is not healthy due to excessive nitrogen waste. Protein Deficiency and Excess When protein intake is below the DRI and energy intake is adequate, protein deficiency, or protein malnutrition, occurs. This state of protein deficiency is called kwashiorkor. Kwashiorkor, a term derived from Africa, meaning “the one who is displaced” and refers to the development of the condition after a sibling is born and the older child is weaned from the breast. If the older child then is placed on a diet that is inadequate in protein, with plenty of Calories, the signs and symptoms of kwashiorkor (peripheral edema, protruding belly, decreased muscle mass, hair and skin changes, irritability, and lethargy) emerge (see Figure 3.14). Edema is a common sign of kwashiorkor. One of the functions of protein is to regulate fluid balance. With low protein status, fluid shifts from the bloodstream to the extremities, causing peripheral edema. Figure 3.14 Marasmus (top) and kwashiorkor (bottom). AFP/Stringer/Getty Images Mauro Fermariello/Science Source When both protein and energy (Calorie) intake are inadequate, protein-energy malnutrition, also called marasmus, occurs. Marasmus is characterized by total body wasting (see Figure 3.14). Both kwashiorkor and marasmus occur primarily in undeveloped countries, but in developed countries, individuals who are on starvation diets, are poor or abused, or who are in hypermetabolic states can also experience marasmus. In severe cases, refeeding the individual is a delicate matter. The person cannot be immediately given a nutritionally balanced diet for protein. The unaccustomed ingestion of protein would cause fluid to shift too rapidly into the bloodstream and lead to sudden death. Refeeding is gradual and progressive over several weeks to months until a healthy intake is established. Depending on the duration and severity of the deficiency, permanent damage may have occurred. The intake of high-protein diets causing protein excess is most common in some athletes and fad dieters. Consuming excess dietary protein has negative health consequences. High-protein foods tend to be from animal sources that are also high in fat, phosphorus, and cholesterol. Though high-protein diets have been touted to promote weight loss, the success rate of losing weight and keeping the lost weight off for more than two years from this type of dietary pattern is low., , , , Further, this style of eating doesn’t promote the adoption of a healthy diet, because it is limited in fruits, vegetables, whole grains, and low-fat dairy products or alternatives. Thus, there is a tendency in the long run for high-protein diets to promote obesity, heart disease, cancer, and osteoporosis., , , , , , Other health risks from consuming high amounts of protein in excess include dehydration, calcium and zinc loss, and spleen enlargement. Chronic protein overload is detrimental to kidney function in individuals with kidney disease and when coupled with low carbohydrate intake, can cause metabolic acidosis. Interestingly, vitamin is needed to process protein, so highprotein diets may increase the chance for developing a vitamin deficiency., 3.3 Photosynthesis and Fiber Introduction In this section, we will discuss how carbohydrates are made (synthesized) in plants and photosynthetic life forms through a process of carbon fixing. We will look at the variety of carbohydrate molecules, some of which are digestible (starch and sugars) and some that are indigestible (cellulose and other fibers types). We will see that consuming adequate amounts of the indigestible carbohydrate (fiber) has many health benefits. Photosynthesis Carbohydrates, including fiber, are found mostly in plant foods and are made by a process of carbon fixing referred to as photosynthesis (see Figure 3.15 and BioBeat 3.6). Photosynthesis occurs via this simple formula: Carbon dioxide plus water plus sunlight energy makes carbohydrate and water. This process takes place inside one of the plant cell organelles called the chloroplast and requires chlorophyll, the plant pigment that captures electromagnetic sunlight energy to make carbohydrate molecules, including sugars, starches, and fibers, from carbon dioxide and water. Figure 3.15 Photosynthesis is the process by which plants make carbohydrates. © 2016 Cengage Learning FFiber When plant foods are consumed, not only are a variety of nutrients consumed, but fiber as well. A common fiber type called cellulose consists of many glucose units linked together by beta chemical bonds, thus it is a complex carbohydrate. Because humans do not produce digestive enzymes to break the beta bonds in fiber, fiber is an indigestible, noncaloric (0 Calories per gram) polysaccharide. It is important to know how much fiber a food item contains so that the dietary consumption can be appropriately planned for adequate intake. Presently, dietary and total fiber values are determined in foods. When either isolated or synthetic fiber is directly added to a processed food, the amount added is predetermined, and thus the quantity is known. Some fiber types have been termed functional fiber because of their beneficial physiological effects in addition to their indigestible benefits. Let’s discuss dietary, functional, and total fiber in more detail. Dietary Fiber Dietary fiber consists of the edible, indigestible components of carbohydrates and lignin naturally found in plant foods. The most common dietary fiber consumed is cellulose. Dietary fiber is measured as the residue remaining after digestion, absorption, and excretion has occurred in vivo. In vivo means “tested in a living system.” In this case, an animal is fed a known quantity of a plant food, and the indigestible carbohydrate residue is measured after excretion. So the amount of dietary fiber present in food is the in vivo measurement of indigestible material. This measurement for fiber is used to label the grams of fiber per serving in human foods., Functional Fiber Indigestible carbohydrates, either synthetic or isolated from natural sources, are sometimes added as ingredients to processed foods (see Table 3.2) and have additional beneficial physiological effects in humans. Examples are cellulose gel, fructooligosaccharides, psyllium, inulin, xanthan gum, guar gum, or flaxseed meal, which contains lignin. Adding functional fiber to the diet in processed foods provides a source of fiber to the individual as well as health benefits; for example, psyllium may improve heart health, and fructooligosaccharides may improve gastrointestinal health., Table 3.2 Comparison of Whole-Wheat Bread and High-Fiber White Bread The nutrition facts are shown, and the functional fibers added to boost the fiber content are highlighted in the ingredient list. © 2019 Cengage Learning Total Fiber The sum of dietary plus functional fiber in food is the total fiber value that is reflected as the fiber content in grams on food package labels in the Nutrition Facts panel. It is the total grams of fiber present per serving. BioBeat 3.6 Photosynthesis Some organisms and green plants have the ability to convert light energy absorbed by chlorophyll into chemical energy through the process of photosynthesis. This process requires chlorophyll, carbon dioxide, water, and sunlight. Carbohydrate chemical structures are produced, and oxygen is released. Carbon fixing is an anabolic chemical reaction. Specifically, . The stored chemical energy in carbohydrate, when digested, is then available to the organism itself, as well as to other organisms that can degrade the organic material produced in the photosynthetic organism (e.g., a human eating spinach). Photosynthesis produces simple carbohydrates and complex carbohydrates, including fibers. An added bonus is that all animals and aerobic organisms can use the oxygen that plants generate while making carbohydrates. How does photosynthesis impact your well-being? Categories of Fiber There are many types of fiber, with varying chemical structures that affect the body in different ways (see the section called “Benefits and Actions of Fiber,”). Though there are different types of fibers found in whole plant foods, they have an overarching property that causes them to be categorized as soluble or insoluble. Soluble fibers soften and gel in water, whereas insoluble fibers do not soften in water but do attract water molecules (see Table 3.3)., Table 3.3 Some Dietary Fiber Facts Category Solubility Fibers Common Food Sources Soluble Soften and gel in water Pectins, gums, mucilages Fruits (like apple pectin), vegetables, legumes, and oats (e.g., oatmeal, cereals) Insoluble Do not soften or gel in water; do attract water Cellulose, hemicellulose, lignins Whole-grain foods, celery strings, apple peels © 2016 Cengage Learning Fiber Recommendations How much fiber is needed in the daily diet for optimal health and functioning? There are standard adult DRIs for fiber. The general DRI for total fiber intake is 38 grams for an adult male and 25 grams for an adult female. However, these values are not personalized. A more accurate calculation of an individual’s fiber recommendation is derived based on that person’s daily Calorie intake. This personal recommendation for fiber is 1.4 grams per 100 Calories consumed. Let’s put this concept into action by looking at an example. How much fiber does a person eating 4,200 Calories per day need? You can calculate the answer using this formula: The answer would be This gives approximately 59 grams as the personalized recommendation for fiber for this Calorie intake level. If another person eats 1,430 Calories, then the fiber need would be Data Practice Solah consumed 2,321 Calories and 17 grams of dietary fiber today. What is her personalized recommendation for fiber based on this Calorie intake? What percentage of her personalized recommendation did she consume for dietary fiber? What health implications can she expect to experience based on her dietary fiber intake? To obtain the health benefits from consuming adequate fiber, high-fiber foods should be incorporated into the daily diet. A high-fiber food contains more than 2 grams of fiber per serving. In general, whole plant foods (legumes, grains, fruits, and vegetables) or products made with whole plant foods provide a good source of fiber. Examples of fiber-containing foods are provided in Table 3.4 and are shown by fiber category type in Figure 3.16. Adequate-fiber diets meet the fiber personalized recommendation of 1.4 grams per 100 Calories consumed, and high-fiber diets provide at least 2 grams of fiber per 100 Calories. Table 3.4 Where’s the Fiber? Food Group Very High (> 4 g) High (2–4 g) Good (1–2 g) Low (≤ 1 g) Grain ½ cup bran flakes 1 cup shredded whole-wheat or whole multi-grain cereals 1 cup oatmeal or brown rice cereal 1 slice whole-wheat bread 1 slice rye bread ½ cup brown or wild rice 1 corn tortilla 1 cup corn flakes ½ cup white rice ½ cup pasta Vegetable ½ cup legumes (dried beans) ½ cup broccoli, cauliflower, corn, beans, cabbage 1 ounce nuts/seeds ½ cup carrots, green pepper, celery, onion, lettuce 1 cup some vegetable juices Fruit N/A 1 apple, banana, orange, peach 1 cup berries 2 prunes ½ cup melon flesh 1 cup fruit juice © 2016 Cengage Learning Figure 3.16 Food sources of dietary fiber. © 2016 Cengage Learning There are many benefits from having an adequate fiber intake; however, as with many things, too much of a good thing can be bad. Though a Tolerable Upper Intake Level for fiber has not been established, there are negative effects associated with too much fiber in the diet. We will next discuss the benefits of adequate fiber and the negative effects of too much fiber. Benefits and Actions of Fiber There are many benefits derived from consuming dietary fiber; several are highlighted here. Both soluble and insoluble fiber add bulk to the diet and increase stool volume. All types of fiber increase the volume of chyme through the GI tract. Also, high-fiber foods have decreased caloric density. In other words, you can eat larger volumes of food with fewer Calories absorbed. For example, having a slice of high-fiber bread with a glass of water or eating a salad with nonfat dressing to begin a meal will help reduce overall Calorie intake. Dietary fiber is also a stool softener. The carbohydrate-related molecules that make up fiber associate strongly with water molecules, creating a softer stool that is easier to move through the GI tract, and is effective for relieving constipation (promoting laxation). The abundant insoluble fiber cellulose is the main fiber that softens stool by attracting water and all fiber types soften stool. Fiber also decreases transit time. This means fiber decreases the amount of time food spends in the GI tract. Soft stool moves more quickly through both the small and large intestines. The exposure time of the large intestine cells to potential carcinogens in the feces is decreased, so the risk for colorectal cancer may be decreased. All types of fiber have this benefit. A high-fiber diet also improves the tone of GI tract muscles. Peristalsis is the circular wave action that propels food through the GI tract. Because fiber types retain water, fiber softens waste and increases the volume of chyme passing through the GI tract. This allows the muscles of the GI tract to exercise better during peristalsis resulting in improved muscular strength. Having strong muscles decreases the risk of having GI tract diseases like diverticular disease. All types of fiber have this benefit. Unique to soluble fiber types is its molecular affinity to bind lipids, specifically cholesterol and thus promote heart health. The fiber in oat bran and legumes (soluble fiber) is particularly effective in binding the cholesterol-rich bile salts and also undigested breakdown products of fats that may have the potential for carcinogenesis. Besides the possibility of reducing the risk for colon cancer, soluble fiber reduces blood or serum cholesterol levels and can be beneficial in the prevention of heart disease. Finally, soluble fiber increasesgastric emptying time. This means fiber increases the time chyme spends in the stomach. Associated with increased gastric emptying time with the presence of soluble fiber, the rate of glucose absorption is slowed, thus the blood sugar level after eating is lower and may be more favorable for individuals with diabetes and metabolic syndrome., , , , , , , , , , Negative Effects of Too Much Fiber Although fiber is beneficial in many ways, too much fiber has unpleasant effects; for example, a sudden transition to a high-fiber diet can cause gas and bloating. Bloating occurs because of gas produced when bacteria in the colon decompose or catabolize fiber molecules. To avoid this unpleasant side effect, a person should transition slowly to a high-fiber diet by gradually replacing low-fiber foods with higher-fiber foods. A diet too high in fiber can cause frequent bowel movements. Having large, soft stools and frequent bowel movements is usually a benefit, but when fiber intake is too high, waste volume and frequency may become excessive and unhealthy. There is no real definition for normal bowel movements, as this is a personal thing—usually once or twice a day for most people—but anything from more than two per day and less than one per two days is probably approaching abnormal, causing the person to feel discomfort. Another potential drawback of consuming too much fiber is the binding of positively charged minerals. Certain fiber types can bind to and prevent the absorption of positively charged minerals like calcium, magnesium, zinc, copper, and iron. This effect has been shown to affect nutritional status in animals, but not humans. Also, certain fibers can bind beta-carotene and other carotenoids, blocking the absorption of beta-carotene (provitamin A). The decreased caloric value associated with high-fiber diets may cause Calorie deficiency. Fiber decreases energy intake because of the reduced caloric density. For an overweight adult, this characteristic of fiber is a benefit, but for an undernourished child, it is a drawback. For the very young and the very old, it may be difficult to achieve energy balance if dietary fiber intake is too high. Lastly, a lack of sufficient water consumption with a high-fiber intake can cause intestinal blockage. This is especially true with encapsulated fiber supplements. Encapsulated fiber dietary supplements intended to promote weight loss have been known to cause blockages of the gastrointestinal tract. Such blockages usually require medical intervention. Effect of Whole-Grain Processing on Fiber Grains are the seeds of grass-type plants and are also consumed as foods or used to make food products like bread, cereal, and pasta. Some examples of whole grains that can be processed or eaten in their whole state include wheat, barley, rye, oats, corn, and rice. Some other grains eaten as staple foods in various parts of the world include amaranth, buckwheat, millet, quinoa, spelt, and teff. In each case the kernel of grain is protected by a husk that is always removed before marketing. So a whole-grain or whole-grain–containing food product has the bran, germ, and endosperm of the grain (see Figure 3.17). When a whole grain is refined, the bran layer containing fiber and the germ layer containing vitamins, minerals, and fatty acids are removed. This leaves the endosperm for use in refined-grain food products. The endosperm contains starch and protein, which are both needed for baked-food product quality, but it has far less fiber, vitamins, and minerals. Figure 3.17 Basic components of a wheat plant and wheat kernel. © 2016 Cengage Learning When whole grains are refined, the nutrient content of the food product is diminished. Because of this loss of essential nutrients, the Enrichment Act of 1942 was passed with the goal of improving the nutritional quality of refined grains. Nutrients that have been added back by law in the enrichment process include iron, thiamin, riboflavin, and niacin. As of January 1, 1998, folic acid has also been added back into refined bread and cereal products. These nutrients are added back to enriched products at levels close to those present in the whole grain., , It is important to realize that whole-grain products are still more nutritious than enriched products, because not all of the nutrients lost in the grain-refinement process are added back into enriched processed foods; vitamin , magnesium, and zinc are not included in the enrichment process. Further, fiber content is greatly diminished by refining whole grains. Thus, fewer nutrients are obtained from consuming the refined food product than from the whole, unrefined food. A refined product with functional fiber added to the processed food, such as soy fiber added as an ingredient to refined wheat bread or white bread that has added fiber and calcium, are examples of refined-grain products with fiber and nutrients added back (recall Table 3.2). To compare the nutritional differences between whole-grain bread and enriched white bread, see Figure 3.18. Figure 3.18 Nutrients in whole-grain, enriched white, and unenriched white breads. Note: Folate is the nutrient form in whole grain bread, whereas the active nutrient form, folic acid, is added by law to enriched bread. © 2016 Cengage Learning iber When plant foods are consumed, not only are a variety of nutrients consumed, but fiber as well. A common fiber type called cellulose consists of many glucose units linked together by beta chemical bonds, thus it is a complex carbohydrate. Because humans do not produce digestive enzymes to break the beta bonds in fiber, fiber is an indigestible, noncaloric (0 Calories per gram) polysaccharide. It is important to know how much fiber a food item contains so that the dietary consumption can be appropriately planned for adequate intake. Presently, dietary and total fiber values are determined in foods. When either isolated or synthetic fiber is directly added to a processed food, the amount added is predetermined, and thus the quantity is known. Some fiber types have been termed functional fiber because of their beneficial physiological effects in addition to their indigestible benefits. Let’s discuss dietary, functional, and total fiber in more detail. Dietary Fiber Dietary fiber consists of the edible, indigestible components of carbohydrates and lignin naturally found in plant foods. The most common dietary fiber consumed is cellulose. Dietary fiber is measured as the residue remaining after digestion, absorption, and excretion has occurred in vivo. In vivo means “tested in a living system.” In this case, an animal is fed a known quantity of a plant food, and the indigestible carbohydrate residue is measured after excretion. So the amount of dietary fiber present in food is the in vivo measurement of indigestible material. This measurement for fiber is used to label the grams of fiber per serving in human foods., Functional Fiber Indigestible carbohydrates, either synthetic or isolated from natural sources, are sometimes added as ingredients to processed foods (see Table 3.2) and have additional beneficial physiological effects in humans. Examples are cellulose gel, fructooligosaccharides, psyllium, inulin, xanthan gum, guar gum, or flaxseed meal, which contains lignin. Adding functional fiber to the diet in processed foods provides a source of fiber to the individual as well as health benefits; for example, psyllium may improve heart health, and fructooligosaccharides may improve gastrointestinal health., Table 3.2 Comparison of Whole-Wheat Bread and High-Fiber White Bread The nutrition facts are shown, and the functional fibers added to boost the fiber content are highlighted in the ingredient list. © 2019 Cengage Learning Total Fiber The sum of dietary plus functional fiber in food is the total fiber value that is reflected as the fiber content in grams on food package labels in the Nutrition Facts panel. It is the total grams of fiber present per serving. BioBeat 3.6 Photosynthesis Some organisms and green plants have the ability to convert light energy absorbed by chlorophyll into chemical energy through the process of photosynthesis. This process requires chlorophyll, carbon dioxide, water, and sunlight. Carbohydrate chemical structures are produced, and oxygen is released. Carbon fixing is an anabolic chemical reaction. Specifically, . The stored chemical energy in carbohydrate, when digested, is then available to the organism itself, as well as to other organisms that can degrade the organic material produced in the photosynthetic organism (e.g., a human eating spinach). Photosynthesis produces simple carbohydrates and complex carbohydrates, including fibers. An added bonus is that all animals and aerobic organisms can use the oxygen that plants generate while making carbohydrates. How does photosynthesis impact your well-being? Categories of Fiber There are many types of fiber, with varying chemical structures that affect the body in different ways (see the section called “Benefits and Actions of Fiber,”). Though there are different types of fibers found in whole plant foods, they have an overarching property that causes them to be categorized as soluble or insoluble. Soluble fibers soften and gel in water, whereas insoluble fibers do not soften in water but do attract water molecules (see Table 3.3)., Table 3.3 Some Dietary Fiber Facts Category Solubility Fibers Common Food Sources Soluble Soften and gel in water Pectins, gums, mucilages Fruits (like apple pectin), vegetables, legumes, and oats (e.g., oatmeal, cereals) Insoluble Do not soften or gel in water; do attract water Cellulose, hemicellulose, lignins Whole-grain foods, celery strings, apple peels © 2016 Cengage Learning Fiber Recommendations How much fiber is needed in the daily diet for optimal health and functioning? There are standard adult DRIs for fiber. The general DRI for total fiber intake is 38 grams for an adult male and 25 grams for an adult female. However, these values are not personalized. A more accurate calculation of an individual’s fiber recommendation is derived based on that person’s daily Calorie intake. This personal recommendation for fiber is 1.4 grams per 100 Calories consumed. Let’s put this concept into action by looking at an example. How much fiber does a person eating 4,200 Calories per day need? You can calculate the answer using this formula: The answer would be This gives approximately 59 grams as the personalized recommendation for fiber for this Calorie intake level. If another person eats 1,430 Calories, then the fiber need would be Data Practice Solah consumed 2,321 Calories and 17 grams of dietary fiber today. What is her personalized recommendation for fiber based on this Calorie intake? What percentage of her personalized recommendation did she consume for dietary fiber? What health implications can she expect to experience based on her dietary fiber intake? To obtain the health benefits from consuming adequate fiber, high-fiber foods should be incorporated into the daily diet. A high-fiber food contains more than 2 grams of fiber per serving. In general, whole plant foods (legumes, grains, fruits, and vegetables) or products made with whole plant foods provide a good source of fiber. Examples of fiber-containing foods are provided in Table 3.4 and are shown by fiber category type in Figure 3.16. Adequate-fiber diets meet the fiber personalized recommendation of 1.4 grams per 100 Calories consumed, and high-fiber diets provide at least 2 grams of fiber per 100 Calories. Table 3.4 Where’s the Fiber? Food Group Very High (> 4 g) High (2–4 g) Good (1–2 g) Low (≤ 1 g) Grain ½ cup bran flakes 1 cup shredded whole-wheat or whole multi-grain cereals 1 cup oatmeal or brown rice cereal 1 slice whole-wheat bread 1 slice rye bread ½ cup brown or wild rice 1 corn tortilla 1 cup corn flakes ½ cup white rice ½ cup pasta Vegetable ½ cup legumes (dried beans) ½ cup broccoli, cauliflower, corn, beans, cabbage 1 ounce nuts/seeds ½ cup carrots, green pepper, celery, onion, lettuce 1 cup some vegetable juices Fruit N/A 1 apple, banana, orange, peach 1 cup berries 2 prunes ½ cup melon flesh 1 cup fruit juice © 2016 Cengage Learning Figure 3.16 Food sources of dietary fiber. © 2016 Cengage Learning There are many benefits from having an adequate fiber intake; however, as with many things, too much of a good thing can be bad. Though a Tolerable Upper Intake Level for fiber has not been established, there are negative effects associated with too much fiber in the diet. We will next discuss the benefits of adequate fiber and the negative effects of too much fiber. Benefits and Actions of Fiber There are many benefits derived from consuming dietary fiber; several are highlighted here. Both soluble and insoluble fiber add bulk to the diet and increase stool volume. All types of fiber increase the volume of chyme through the GI tract. Also, high-fiber foods have decreased caloric density. In other words, you can eat larger volumes of food with fewer Calories absorbed. For example, having a slice of high-fiber bread with a glass of water or eating a salad with nonfat dressing to begin a meal will help reduce overall Calorie intake. Dietary fiber is also a stool softener. The carbohydrate-related molecules that make up fiber associate strongly with water molecules, creating a softer stool that is easier to move through the GI tract, and is effective for relieving constipation (promoting laxation). The abundant insoluble fiber cellulose is the main fiber that softens stool by attracting water and all fiber types soften stool. Fiber also decreases transit time. This means fiber decreases the amount of time food spends in the GI tract. Soft stool moves more quickly through both the small and large intestines. The exposure time of the large intestine cells to potential carcinogens in the feces is decreased, so the risk for colorectal cancer may be decreased. All types of fiber have this benefit. A high-fiber diet also improves the tone of GI tract muscles. Peristalsis is the circular wave action that propels food through the GI tract. Because fiber types retain water, fiber softens waste and increases the volume of chyme passing through the GI tract. This allows the muscles of the GI tract to exercise better during peristalsis resulting in improved muscular strength. Having strong muscles decreases the risk of having GI tract diseases like diverticular disease. All types of fiber have this benefit. Unique to soluble fiber types is its molecular affinity to bind lipids, specifically cholesterol and thus promote heart health. The fiber in oat bran and legumes (soluble fiber) is particularly effective in binding the cholesterol-rich bile salts and also undigested breakdown products of fats that may have the potential for carcinogenesis. Besides the possibility of reducing the risk for colon cancer, soluble fiber reduces blood or serum cholesterol levels and can be beneficial in the prevention of heart disease. Finally, soluble fiber increasesgastric emptying time. This means fiber increases the time chyme spends in the stomach. Associated with increased gastric emptying time with the presence of soluble fiber, the rate of glucose absorption is slowed, thus the blood sugar level after eating is lower and may be more favorable for individuals with diabetes and metabolic syndrome., , , , , , , , , , Negative Effects of Too Much Fiber Although fiber is beneficial in many ways, too much fiber has unpleasant effects; for example, a sudden transition to a high-fiber diet can cause gas and bloating. Bloating occurs because of gas produced when bacteria in the colon decompose or catabolize fiber molecules. To avoid this unpleasant side effect, a person should transition slowly to a high-fiber diet by gradually replacing low-fiber foods with higher-fiber foods. A diet too high in fiber can cause frequent bowel movements. Having large, soft stools and frequent bowel movements is usually a benefit, but when fiber intake is too high, waste volume and frequency may become excessive and unhealthy. There is no real definition for normal bowel movements, as this is a personal thing—usually once or twice a day for most people—but anything from more than two per day and less than one per two days is probably approaching abnormal, causing the person to feel discomfort. Another potential drawback of consuming too much fiber is the binding of positively charged minerals. Certain fiber types can bind to and prevent the absorption of positively charged minerals like calcium, magnesium, zinc, copper, and iron. This effect has been shown to affect nutritional status in animals, but not humans. Also, certain fibers can bind beta-carotene and other carotenoids, blocking the absorption of beta-carotene (provitamin A). The decreased caloric value associated with high-fiber diets may cause Calorie deficiency. Fiber decreases energy intake because of the reduced caloric density. For an overweight adult, this characteristic of fiber is a benefit, but for an undernourished child, it is a drawback. For the very young and the very old, it may be difficult to achieve energy balance if dietary fiber intake is too high. Lastly, a lack of sufficient water consumption with a high-fiber intake can cause intestinal blockage. This is especially true with encapsulated fiber supplements. Encapsulated fiber dietary supplements intended to promote weight loss have been known to cause blockages of the gastrointestinal tract. Such blockages usually require medical intervention. Effect of Whole-Grain Processing on Fiber Grains are the seeds of grass-type plants and are also consumed as foods or used to make food products like bread, cereal, and pasta. Some examples of whole grains that can be processed or eaten in their whole state include wheat, barley, rye, oats, corn, and rice. Some other grains eaten as staple foods in various parts of the world include amaranth, buckwheat, millet, quinoa, spelt, and teff. In each case the kernel of grain is protected by a husk that is always removed before marketing. So a whole-grain or whole-grain–containing food product has the bran, germ, and endosperm of the grain (see Figure 3.17). When a whole grain is refined, the bran layer containing fiber and the germ layer containing vitamins, minerals, and fatty acids are removed. This leaves the endosperm for use in refined-grain food products. The endosperm contains starch and protein, which are both needed for baked-food product quality, but it has far less fiber, vitamins, and minerals. Figure 3.17 Basic components of a wheat plant and wheat kernel. © 2016 Cengage Learning When whole grains are refined, the nutrient content of the food product is diminished. Because of this loss of essential nutrients, the Enrichment Act of 1942 was passed with the goal of improving the nutritional quality of refined grains. Nutrients that have been added back by law in the enrichment process include iron, thiamin, riboflavin, and niacin. As of January 1, 1998, folic acid has also been added back into refined bread and cereal products. These nutrients are added back to enriched products at levels close to those present in the whole grain., , It is important to realize that whole-grain products are still more nutritious than enriched products, because not all of the nutrients lost in the grain-refinement process are added back into enriched processed foods; vitamin , magnesium, and zinc are not included in the enrichment process. Further, fiber content is greatly diminished by refining whole grains. Thus, fewer nutrients are obtained from consuming the refined food product than from the whole, unrefined food. A refined product with functional fiber added to the processed food, such as soy fiber added as an ingredient to refined wheat bread or white bread that has added fiber and calcium, are examples of refined-grain products with fiber and nutrients added back (recall Table 3.2). To compare the nutritional differences between whole-grain bread and enriched white bread, see Figure 3.18. Figure 3.18 Nutrients in whole-grain, enriched white, and unenriched white breads. Note: Folate is the nutrient form in whole grain bread, whereas the active nutrient form, folic acid, is added by law to enriched bread. © 2016 Cengage Learning 3.4 Carbohydrate Storage and Disorders Introduction Glucose is a very important carbohydrate molecule. The majority of six-carbon sugars consumed in the diet are glucose, and the concentrations of glucose in the blood are tightly regulated. In this section we will focus on blood sugar (also called blood glucose), blood sugar regulation, starch, and glycogen, and the hormones insulin and glucagon. Additionally, lactose intolerance and lactose maldigestion, hypoglycemia (low blood sugar levels) and hyperglycemia (high blood sugar levels), and diabetes will be discussed as health conditions related to altered carbohydrate metabolism. Carbohydrate Disorders There are many disorders related to carbohydrates. Some that will be explored include lactose intolerance, milk allergy, hypoglycemia, and diabetes., , Lactose Intolerance and Lactose Maldigestion Drinking cow’s milk throughout life is common among Americans, but what other species drink the mother’s milk of other species and lifelong? Are there any genetic signatures leading to natural selection from this behavior? Why do some people, especially those from certain cultural heritages, tolerate cow’s milk while others do not? The answers are tied to human biocultural evolution and how several alterations to the human genome can contribute to genetic variance at the population level (see BioBeat 3.8). The ability to digest and absorb mother’s milk is a necessity for the survival of any infant mammal, including humans. Historically, when milk consumption ends after weaning, the lactase enzyme gene is no longer required, so the gene is turned off. With this gene inactive, the consumption of milk sugar (lactose) or milk products leads to a severe gastrointestinal condition known as lactose intolerance. In general, many individuals of American and Northern European descent tend to be more lactase persistent and lactose tolerant, whereas Native Americans and people from Asia and West Africa, whose ancestors are from non-cattleranching cultures, tend to be more lactase deficient and lactose intolerant. As human age, the concentrations of lactase diminishes causing an inability to digest lactose. We can label this condition of not being able to digest lactose adequately from milk products as lactose maldigestion., BioBeat 3.8 The Evolution of Lactase Persistence As late as 5000 bc, fossil evidence indicates that ancient adult Europeans were unable to digest milk. It has been estimated that 7,000 to 9,000 years ago, one of the strongest human genetic natural selection signatures took place by the origination of cattle ranching in the Middle East, making cow’s milk readily available for humans to drink. Natural selection is the evolution of a species through genetic alterations in heritable traits that enable the species to adapt and survive in its environment. The new genetic trait makes the species stronger or gives it a greater ability to endure. This trait is passed on to new offspring in successive generations. Being able to drink milk into and through adulthood (by keeping the lactase enzyme gene active) is advantageous because of the nutritional value and source of fluid for the body. The Fulani and Tutsi are two cattle-ranching tribes of East Africa. They coevolved with European cattle ranchers. Yet, the two cultures have specific DNA mutations, or molecular signatures, enabling their lactase gene persistence and thus lactose tolerance. Several distinct single-gene mutations occurring at the population level (single nucleotide polymorphism [SNP]) have been tracked genetically through these and other cultures. The studies of inheritance patterns and evolution reveal varying degrees of lactase gene persistence and thus lactose tolerance. Two such common gene mutations causing lactase persistence and thus lactose tolerance are shown in Figure 3.21., , , How do genetics and evolution affect your tolerance of lactose? Figure 3.21 The genetics and co-evolution of lactase persistence in East Africans and Northern Europeans, with mutations highlighted on the minichromosome maintenance 6 (MCM6) gene, which alters the sense (S) and antisense (AS) base pair (bp) sequence of adenine (A), thymine (T), cytosine (C), and guanine (G). When a gene sequence alteration occurs commonly in a population, it is called a single nucleotide polymorphism (SNP). © 2020 Cengage Learning Lactose intolerance occurs when an enzyme called lactase, which is needed to digest lactose (that is, to break the glucose-galactose bond), is missing. With lactose maldigestion, the lactase enzyme is reduced. Normally, cells in the small intestine produce lactase, and the enzyme is present in pancreatic digestive secretions. When the lactase enzyme is missing or insufficient, and a person consumes lactose from dairy products, lactose digestion does not occur in the small intestine. Intact, undigested lactose is not absorbable, and the result is that high concentrations of lactose enter the colon and are metabolized by bacteria in the GI tract. The metabolic by-products produced by the bacteria from the lactose catabolism cause fluid to shift into the GI tract, leading to osmotic diarrhea. The gases produced from the bacterial catabolism of lactose also contribute to the discomforts of gas, bloating, cramps, and diarrhea (see Figure 3.22)., Figure 3.22 Osmotic diarrhea results from lactose intolerance. © 2016 Cengage Learning Individuals with lactose maldigestion may be able to tolerate a small amount of lactose from dairy products, especially those produced with bacteria (such as yogurt, aged cheese, and milk products with bacteria added, like acidophilus milk). In contrast, individuals with lactose intolerance may need to avoid the intake of dairy products altogether or use an over-the-counter product to supply the missing lactase enzyme upon ingestion of lactose from dairy products. Dairy products include milk, yogurt, cheese, and many puddings and frozen desserts like ice cream. These products are commonly made from cow’s or goat’s milk. The conditions of lactose intolerance and lactose maldigestion are influenced ecologically by intestinal bacteria. These bacteria can metabolize lactose and thus contribute to the negative effects of milk consumption in the lactose-intolerant person. Additionally, excess physiological, psychological, physical, and emotional stress can cause epithelial cells at the tip of the villi, where lactase production is the most abundant, to waste away (atrophy). However, these epithelial cells of the GI tract, like those of the skin, regenerate within several days to weeks. So if the stressor is removed and the lactase-persistent gene is present, then lactose tolerance may be regained in this situation. BioBeat 3.9 Food Allergies Food allergies occur when the protein components within foods are recognized by the immune system as foreign, causing the body to produce antibodies directed against them. Antibodies (also called immunoglobulins [Ig]) are large protein structures produced by B lymphocytes (immune cells) that circulate in the body to fight disease and infection. There are five classes of antibodies: IgA, IgD, IgE, IgG, and IgM. IgEs are involved most commonly in allergic reactions. The IgE is made by the immune system to specifically bind to a protein component of the offending food. When a protein in food is known to cause an allergic response in a person, the protein is termed an allergen. Normally, antibodies are directed against proteins in viruses or bacteria. In this case, the offending protein is called an antigen. Allergens and antigens are foreign substances that elicit an immune response. A classic allergic response that is IgE mediated is the type I immediate hypersensitivity reaction. This reaction occurs within seconds to minutes of allergen exposure. Allergic reactions may also be delayed. These reactions occur within hours to days of allergen exposure. Signs and symptoms associated with the allergic reaction include nausea, vomiting, diarrhea, skin reactions like itchy hives and dermatitis, or in some cases, increased secretions of the mucus membranes that are most noticeable in the upper respiratory tract or the GI tract. Asthma and anaphylactic shock are severe and even life-threatening responses to allergens., , , , , , , ,, Is your immune system reactive to food protein antigens? Milk Allergy Lactose intolerance is different from milk allergy. Many people experience a significant histamine-mediated allergic response when dairy products are consumed. The histamine response causes mucus membranes to secrete fluid. This is why allergic responses usually involve skin, gut, and respiratory distress. With milk allergy, the culprit is not the lactose sugar, but rather the casein protein. The immune system of the person with a milk allergy reacts to the ingested casein protein as if it were a foreign substance invading the body (see BioBeat 3.9 and Figure 3.23). The GI tract is lined with an enormous number of immune cells, collectively called the gut-associated lymphoid tissue. These cells are meant to fight off foreign substances such as bacteria and viruses. However, some people have inherited allergies, and their immune cells react to foods, environmental agents (e.g., pollen, mold, pollution), and/or animal dander. Figure 3.23 Mast cells (A) in an allergic immune-mediated response (B). © 2016 Cengage Learning Allergies involve the immune system, and when food is the cause of the allergy, the responses are usually immediate and can include gastrointestinal distress (nausea, vomiting, cramps, and diarrhea), skin reactions (urticaria or hives, rashes, and eczema), and respiratory problems (from mucus formation to narrowing of the airway or anaphylaxis). The common food allergens are specific proteins in milk, eggs, fish, crustacean shellfish, tree nuts, peanuts, wheat, and soybeans. Many other foods cause allergies, including oral allergy syndrome, which is characterized by tingling, itching, and/or swelling of the lips and mouth. An adverse allergy-like reaction to a food or food component that does not trigger an immune response is termed food intolerance. There is limited treatment for food allergy or intolerance besides eliminating the offending food from the person’s diet., , Hypoglycemia Hypoglycemia is a condition characterized by low blood sugar (glucose) levels. Generally, a blood sugar value of less than 60 mg/dL causes symptoms of hypoglycemia. Low blood sugar levels can occur in some people as reactive, spontaneous, or drug-induced hypoglycemia. In this condition, the signs and symptoms are feeling nervous, light-headed, shaky, weak, sweaty, and/or faint, as well as having a headache, blurred vision, and/or hunger. Reactive Hypoglycemia This is a condition in which blood sugar levels drop after eating a load of digestible carbohydrate. Reactive hypoglycemia occurs because too much insulin is secreted in response to a quick, sharp increase in blood sugar level. Too much glucose (blood sugar) is removed too rapidly from the blood because excess insulin caused too much blood glucose to enter the muscles and liver, resulting in low blood sugar levels. The period of low blood sugar levels last usually about 10 minutes until a normal blood sugar level can be re-established. Spontaneous Hypoglycemia Spontaneous hypoglycemia occurs when liver stores of glycogen are depleted, diminishing the body’s ability to maintain a normal blood sugar level. This condition happens to everyone between meals or when adequate amounts of carbohydrate have not been consumed. Drug-Induced Hypoglycemia Drug-induced hypoglycemia lowers blood sugar levels as a side effect or dose issue. Antiinflammatory and thyroid medications are known to cause hypoglycemia. In persons with diabetes, overadministration of insulin and/or excess exercise without proper dietary carbohydrate intake results in hypoglycemia. Diabetes Diabetes is a chronic disease of the endocrine system resulting in the inability to maintain normal blood sugar (glucose) levels. Thus, hyperglycemia (elevated levels of glucose in the bloodstream), or high blood sugar, occurs. Diabetes is most commonly categorized as type 1 or type 2. Both types may be aggravated by increased stress, oxidative stress, and inflammation. Type 2 diabetes, the most common type, is strongly linked to obesity (although not all type 2 diabetics are obese) and a physically inactive lifestyle. The incidence of type 2 diabetes has been rising dramatically, in concert with the worldwide obesity epidemic. Currently, 26 million Americans (more than 8 percent of the population) are estimated to have diabetes, compared with 5.76 million in 1980. Several million American people are undiagnosed or unaware of their disease state. The high level of sugar in the blood puts a diabetic person at increased risk for heart disease, stroke, kidney disease, retinopathy (loss of vision), neuropathy (loss of nerve function), limb atrophy sometimes leading to amputation (due to a combination of problems associated with damage to the circulatory system and nervous system), and decreased life expectancy. Knowing how to manage blood sugar levels in the type 1 or 2 diabetes disease state is critical in order to prevent the complications of hyperglycemia resulting from extensive cellular, tissue, and organ damage (see Table 3.5)., , , , , , , , , , , Table 3.5 Common Complications of Unmanaged Diabetes by Body System Organ/Site Damage Circulatory system Damage to blood vessels causing increased risk for atherosclerosis and high blood pressure as well as heart attack, stroke, and blindness (from damage to the retina of the eye, causing changes to lens shape and vision) Digestive system Gum disease, gastroparesis (nerve damage) causing delayed stomach emptying, heartburn, nausea, and vomiting Endocrine system Abnormal hormonal control of blood glucose causing hyperglycemia Integumentary system Increased risk of skin infection, patchy skin discoloration, and skin thickening on the back of the hands Peripheral nervous system Nerve damage impairing sensations of pain and/or poor circulation, foot ulcers, and possible foot/leg amputation from formation of necrotic (dead) tissue Reproductive system Possibility of gestational diabetes in pregnant women, leading to high birthweight, prenatal complications, and type 2 diabetes later in life Urinary system Damage to blood vessels of the kidney, leading to kidney damage and failure © 2016 Cengage Learning Type 1 Diabetes This type of diabetes occurs in approximately 5 percent of diabetes cases. The onset of the disease is typically acute and occurs most often during the childhood, adolescence, or early adult years. Thus, it has been termed juvenile-onset diabetes. Type 1 diabetes is the severe form of diabetes, difficult to control, caused by an autoimmune reaction against the pancreatic beta cells that has destroyed or reduced the ability of the pancreas to synthesize adequate amounts of insulin to lower blood glucose levels. Because the pancreatic beta cells have been destroyed by the autoimmune response, the body loses its ability to produce insulin. Blood sugar in this case can be lowered only by insulin administration. Type 1 diabetics must eat amounts of carbohydrates compatible with the type and amount of insulin injected, measure their blood sugar levels frequently throughout the day, and adjust their medication or carbohydrate intake to achieve normal blood sugar levels. Insulin is usually injected into soft tissue just under the skin, although an inhaled form holds promise for those with diabetes. It cannot be taken in pill form because insulin is a protein hormone. It would be denatured and digested like any other ingested protein if taken orally, thus rendering it nonfunctional in the bloodstream. Type 2 Diabetes This type of diabetes occurs in approximately 95 percent of diabetes cases. Typically, the onset of the disease occurs during adulthood. However, with the rise in childhood obesity, children are being diagnosed with type 2 diabetes more frequently. Developing type 2 diabetes is predisposed by obesity and genetics (see Figure 3.24). In type 2 diabetes, insulin resistance occurs partly because of a decreased insulin receptor responses in the liver and muscle cells. The “lock” has changed, so the “key” doesn’t work. In other words, the cells of the muscle and liver that normally take up glucose in response to insulin binding to insulin receptors have become resistant to the insulin signal. Thus, even though insulin is present in the blood, blood sugar remains high because muscle cells do not allow glucose to enter. Hyperglycemia and hyperinsulinemia occur commonly in type 2 diabetes. Insulin sensitivity can be re-established with lifestyle changes. The high blood sugar levels occurring in type 2 diabetes can be reduced by making changes in diet, exercise, body weight, and stress management and, if necessary, oral hypoglycemic agents can be taken in pill form to help augment the action of self-produced insulin., , , , , Type 2 diabetes was initially called non-insulin-dependent diabetes mellitus. Now it is considered a lifestyle disease because blood sugar control is quite achievable with regular exercise and consuming a healthy diet that promotes weight loss when needed. Type 2 Diabetes Watch the video about Type 2 Diabetes and the alarming rate of increase. Volume 90% Video supplied by BBC Worldwide Learning Figure 3.24 The type 2 diabetes implicating factors and cycle when unmanaged. © 2016 Cengage Learning Diagnosing Diabetes Diabetes, whether type 1 or type 2, is diagnosed by hyperglycemia. Thus, a fasting blood sugar level will indicate whether a person has a normal glucose level or hyperglycemia. A 12-hour fasting blood glucose level of 126 mg/dL or higher o