PDF Energy 269 (2023) 126775
Document Details
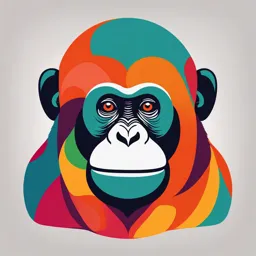
Uploaded by GuiltlessCopernicium3228
Universidade do Porto
2023
Ricardo Pinto, Sofia T. Henriques, Paul E. Brockway, Matthew Kuperus Heun, Tânia Sousa
Tags
Related
- SET Chapter 11: Electrical Supply Systems PDF
- High Voltage Transmission Techniques Lecture Notes PDF
- Energy Audit Report Banswara Syntex Ltd - September 2024 PDF
- Energy Efficiency In Fans And Pumps PDF
- Indian Railways AC Traction Manual Volume 1 - Energy Conservation PDF
- Renewable Energy Executive Summary 2024 PDF
Summary
This article investigates the rise and stall of global electricity efficiency from 1900 to 2017. It analyzes the societal exergy of electricity with considerations of energy carriers, end-uses, and efficiencies. The study finds that while conversion efficiency has improved significantly, final-to-useful efficiency has remained surprisingly stable, highlighting the importance of electrification.
Full Transcript
Energy 269 (2023) 126775 Contents lists available at ScienceDirect Energy journal homepage: w...
Energy 269 (2023) 126775 Contents lists available at ScienceDirect Energy journal homepage: www.elsevier.com/locate/energy The rise and stall of world electricity efficiency:1900–2017, results and insights for the renewables transition Ricardo Pinto a, *, Sofia T. Henriques b, c, Paul E. Brockway d, Matthew Kuperus Heun e, Tânia Sousa a a MARETEC—Marine, Environment and Technology Center, LARSyS, Instituto Superior Técnico, Universidade de Lisboa, Avenida Rovisco Pais, 1, Lisboa, 1049-001, Portugal b CEFUP, Faculdade de Economia da Universidade Do Porto, Rua Dr. Roberto Frias, 4200-464, Porto, Portugal c Department of Economic History, Lund University, Box 7080, S-220 07, LUND, Sweden d Sustainability Research Institute, School of Earth and Environment, University of Leeds, Leeds, LS2 9JT, United Kingdom e Engineering Department, Calvin University, 3201 Burton St. SE, Grand Rapids, MI 49546, USA A R T I C L E I N F O A B S T R A C T Keywords: In the coming renewables-based energy transition, global electricity consumption is expected to double by 2050, Energy efficiency entailing widespread end-use electrification, with significant impacts on energy efficiency. We develop a long- Electricity run, worldwide societal exergy analysis focused on electricity. Our 1900–2017 electricity world database con Carbon intensity tains the energy carriers used in electricity production, final end-uses, and efficiencies. We find world primary-to- Decarbonization Energy history final exergy (i.e. conversion) efficiency increased rapidly from 1900 (6%) to 1980 (39%), slowing to 43% in 2017 Energy end-uses as power station generation technology matured. Next, despite technological evolution, final-to-useful end-use efficiency was surprisingly constant (~48%), due to “efficiency dilution”, wherein individual end-use efficiency gains are offset by increasing uptake of less efficient end uses. Future electricity efficiency therefore depends on the shares of high efficiency (e.g. electrified transport) and low efficiency (e.g. cooling and low temperature heating) end uses. Our results reveal past conversion efficiency increases (carbon intensity of electricity pro duction reduced from 5.23 kgCO2/kWh in 1900 to 0.49 kgCO2/kWh in 2017) did little to decrease global electricity-based CO2 emissions, which rose 380-fold. The historical slow-pace of transition in generation mix and the need to electrify end-uses suggest that strong incentives are needed to meet climate goals. 1. Introduction renewables will mean rapid growth in electricity generation and con sumption into the future, but energy efficiency is a complex and nuanced 1.1. Global electricity demand is projected to have rapid growth issue, with impacts on economic growth, energy rebound, and aggregate efficiency [8–10]. The share of electricity in world total final consumption (TFC) has Electrification of end uses will enable widespread deployment of increased significantly, from 0.1% (1900) to 4% by mid-century (1950), low-carbon, electricity-producing sources of energy, especially wind and and 19% in 2022 [1,2]. Importantly, global electricity demand keeps solar. IRENA forecasts that, by 2050, 33% of final energy for transport rising, and is projected by the International Renewable Energy Agency will be provided by electricity, up from 1% in 2015. Buildings are (IRENA) to double between 2015 and 2050. While electricity gen also expected to increase their electricity demand by 70% until 2050, eration doubled between 1990 and 2014 , carbon dioxide (CO2) from their 2015 value, due to increased cooling demand, electrification emissions associated with electricity increased only slightly less, 87%, of heating, and growing electricity consumption in developing countries from 6.28 GtCO2 to 11.76 GtCO2. To limit end-of-century warming [3,11]. Other forecasts propose scenarios which rely on near 100% to 1.5 ◦ C whilst meeting UN Sustainable Development Goal #7 electrification with renewables to reach climate targets, where demand (affordable and clean energy) , electrification, renewables, and en for electricity is expected to more than double [12–14]. ergy efficiency are thought to be essential. Electrification and Beyond electrification, emerging end uses will add to future * Corresponding author. E-mail address: [email protected] (R. Pinto). https://doi.org/10.1016/j.energy.2023.126775 Received 23 May 2022; Received in revised form 5 December 2022; Accepted 18 January 2023 Available online 20 January 2023 0360-5442/© 2023 The Authors. Published by Elsevier Ltd. This is an open access article under the CC BY license (http://creativecommons.org/licenses/by/4.0/). R. Pinto et al. Energy 269 (2023) 126775 electricity demand, especially information and communication tech three relationships imply increases in final-to-useful efficiency nology (ICT). For three specific categories of ICT (communication net contribute to economic growth and, paradoxically, an increase in the works, personal computers, and data centres), Heddeghem et al. demand of energy, a phenomenon known as energy rebound. Indeed, found that between 2007 and 2012 electricity consumption from ICT Ayres et al. state efficiency gains at the final-to-useful stage lead to uses grew at 7% per year, while overall electricity use increased only 3% higher final energy consumption. However, overall effects of per year, thereby raising the share of total worldwide electricity con final-to-useful efficiency increases on the demand for final energy can be sumption for ICT to 4.6%. The increase in electricity demand due to positive or negative, depending on the presence of rebound effects. electrification and emerging energy uses will be partially controlled by Furthermore, the relationship between electricity efficiency and energy efficiency. aggregate final-to-useful efficiency is complex. In Portugal between 1900 and 2009, the aggregated final-to-useful efficiency was always 1.2. The uncertainty of the impact of energy efficiency on electricity lower than 25% , while final-to-useful efficiency for electricity was demand always above 30%. Also, in Mexico between 1971 and 2009, electricity is the energy carrier with the highest final-to-useful efficiency Energy efficiency can be calculated between different stages of the. The same occurred in the US where Ayres et al. estimated a energy conversion chain. Societies use primary energy such as coal in a high stable, average value of 55%, for the final-to-useful US electricity thermoelectric power plant or wind energy in a wind farm to produce efficiency between 1900 and 2000. Thus, the growing use of electricity final energy in the form of electricity. Afterwards, electricity is trans increases aggregate final-to-useful exergy efficiency. formed to useful energy such as light in lamps or heat in electric heaters Regarding, primary-to-useful efficiency, Brockway et al. anal or heat pumps. yse the UK and USA, for the period 1960–2010. While rising UK elec The estimation of useful energy is crucial because it is closer to the tricity exergy efficiency drove increases to UK aggregate exergy energy services that people and firms want and therefore more closely efficiency, USA aggregate exergy efficiency remained very stable due to tied to economic activity than final energy. As such, it is the appropriate efficiency dilution caused by increasing consumption of low-efficiency stage at which to measure energy when the goal is understanding trends air conditioning. In Portugal, the primary-to-useful efficiency of in the relationship between energy and economic activity, which is an electricity increased between 1900 and 1990 but stagnated afterwards essential step to make scenarios of the future energy transition. One can due to increasing share of electricity consumed in less-efficient estimate the energy efficiency of each conversion, that is the ratio of sectors, mainly residential and commercial. “useful” energy output to “final” energy input. The higher the energy This emerging picture of the role of useful exergy and efficiency on efficiency, the better. However, energy efficiency gives us incomplete economic growth and energy consumption (and CO2 emissions) is illu information about potential energy savings. For example, the energy minating. However, current understanding is based on analyses of single efficiency of an electric heater is 100% which suggests that there are no countries [17,22–25,27,28] or a small number of countries (between 2 potential energy savings. However, this is not the case because a heat and 15 countries) [18,26] over short timescales (40–50 years) [18,24, pump can provide the same output using ¼ of the electricity. Here, we 26,28] with little-to-no electricity end-use detail [25,27]. A few studies address this issue using exergy efficiency which is also the ratio of useful [17,22,23] have longer timescales with more detail on electricity con output to input but using exergy to quantify energy. Exergy is a mea sumption but focus on single countries (Portugal and US). Additionally, surement of energy that quantifies the potential of an energy flow to do these studies use varying methodologies to estimate efficiencies, leading physical work. For electricity and mechanical work, exergy is equal to results inhibiting comparison. At the world level, there are two to the energy content because these types of energy can be completely studies for a single year [30,31] and only one long-run (1900–2010) converted into work. For heat, exergy content is lower than its energy study , which calculated final-to-useful efficiencies using GDP as because even an ideal Carnot machine cannot completely convert heat at proxy, thereby linking energy and economic growth. Additionally, the a temperature TH into work: it needs to reject some heat at a lower long-run study lacks detail in allocations of electricity to end-uses, temperature TC. The fundamental constraint in the conversion of heat assuming constant end-use shares within each sector throughout the into work is associated with the fact that heat carries both energy and period 1900–2010. These assumptions are problematic, because the entropy. Since entropy cannot be destroyed, the Carnot machine needs estimation of overall electricity efficiency is highly dependent on both to reject heat to get rid of the entropy. (a) the detail in allocating electricity to end uses (see Refs. [23,32]) and The use of exergy is important not only to estimate an efficiency that (b) the methods used to estimate efficiencies. provides a meaningful measure of the potential energy savings because the maximum exergy efficiency is 100% but also because exergy effi 1.3. Motivation, aim, contribution, and structure ciency and useful exergy have been empirically linked to economic growth. Economies run on work that (as opposed to heat) shapes The motivation for this paper is based on the increasing importance of materials, assembles machines and products, and transports people, electricity in the future, due to both (a) the need to decarbonise energy goods, and services throughout the economy. To a lesser extent, econ systems and (b) increasing share of end-uses such as ICT. Individual omies also consume heat. But heat can (and probably should) be sup country studies have shown that the efficiency of electricity production plied via a heat pump, which consumes work (usually in the form of and consumption has significant impacts on final-to-useful and primary- electricity). Useful exergy is the amount of work (or electricity) needed to-final efficiencies, economic growth, and greenhouse gas (GHG) as input to the economy when all potential energy savings have been emissions. However, our historical knowledge is incomplete, because accomplished. there is no detailed, world-level exergy-based study covering a long time Three recent studies illustrate the complexities of energy efficiency. span that focuses on electricity end-uses. A long-run analysis of past First, Serrenho et al. [17,18] showed that the ratio of useful exergy to electricity production, efficiency trends, and carbon emissions will GDP for Portugal is approximately constant, a finding that holds for the provide insights to guide scenarios and policies for electrification, re other EU-15 countries if the relative size of heavy industry end uses newables, and energy efficiency. (High temperature heat (HTH)) and domestic end uses (Low Tempera The aim of this article is to evaluate world long-term trends of past ture Heat (LTH)) remain constant. Second, Santos et al. show that an electricity consumption and production, end-uses, efficiency, and car increase in final-to-useful exergy efficiency makes a key contribution to bon intensity. The key contributions of this paper are the development of higher GDP for Portugal. Third, Ferguson et al. shows electricity (a) a detailed world long-run database for electricity production and consumption and economic development are strongly correlated for consumption and (b) historical time series datasets for the evolution of more than 100 countries between 1971 and 1995. Taken together, these primary-to-final, final-to-useful, and primary-to-useful exergy 2 R. Pinto et al. Energy 269 (2023) 126775 efficiencies. hydroelectricity, indicating that production started before Etemad et al. The structure of this paper is as follows: In section 2, we explain the series , so we looked for other data to complete the series until 1900. method for constructing a world database for electricity consumption For Norway 1900–1936, we estimated hydroelectricity production using and production. In section 3, we show results for world electricity pro a report for all hydropower plants in use in 1943. (See supple duction and consumption, efficiencies, and carbon emissions. In section mentary information (SI) A.) For Norway 1930–1960, we estimated 4, we discuss the results in historical perspective and in the context of thermal electricity sources using statistical yearbooks. For Sweden the ongoing decarbonization transition. Section 5 summarizes. 1900–1928, we estimated hydroelectricity production using shares of hydro generation available in Kander et al.. South Africa electricity 2. Data and methods production data was taken from the Bureau of census and statistics between 1917 and 1959 afterwards data was taken from Etemad et al. Electricity production data provides the starting point, because it is. the energy stage available in yearbooks and books, for construction of Data for total, hydro, geothermal, and nuclear electricity production the long run database of primary, final, and useful electricity. Electricity for other countries was obtained from Etemad et al.. Switzerland production is the electricity that leaves the alternator in a power plant, started with a high value of hydroelectricity, so we assumed that the so it is considered final energy. Fig. 1 and table A9 of the supplementary share of hydroelectricity for the early years was equal to the average information A summarize the steps used to calculate primary and useful share of hydroelectricity for the first five years of available data. For exergy as well the main sources used to obtain the data necessary to medium producer countries for 1900–1960, we assumed electricity not these calculations. The following subsections will go into further details generated from hydropower, nuclear or geothermal sources was pro about each stage of the energy conversion chain: primary, final, and duced from coal (except France, Italy, and Norway), as oil was the only useful. other credible source, and no large oil producers are classified as me dium producers. IEA data from 1960 onwards for OECD countries pro vides carrier-level electricity production data for almost all the medium 2.1. Final energy stage: electricity production and sources producers, the non-OECD exceptions being China, Czechoslovakia, India, and South Africa (their IEA data starts in 1971). Our starting point was Etemad et al. “World Energy Production” Small producers comprise all remaining countries that, individually,. Two years were assessed first: 1920 and 1970 Fig. 2. The year 1920 each produced less than 1% of world electricity in 1920 and 1970. For is the first year for which most countries have data available on elec tricity production in Etemad et al.. The year 1970 is the year before small producers, total electricity and hydroelectricity values were taken from Etemad et al. , with the exception of hydroelectricity values for world data are available from the International Energy Agency (IEA). Countries were divided in three groups: large, medium, and small pro Latin America which were obtained from Rubio and Tafunell. The share of thermoelectricity produced by each energy carrier was assumed ducers, as shown in Fig. 3. Large producers (Canada, Germany, Japan, UK, USA, and USSR) supplied more than 5% of the world electricity in equal to the weighted average of medium and large producers, including only non-hydro energy carriers. 1920 or 1970. Fig. 4 shows the share of the world total electricity of these countries for the period 1900–1970 (Canada , Germany 2.2. Primary energy stage: From final-to-primary exergy [37–39], Japan , UK [41,42], USA and USSR [44–46]).1 When no other source of data was available, Etemad et al. was used. For Primary energy gives information about the resources necessary to years in which no data were available, we interpolated linearly. produce the energy we purchase (final energy, such as electricity). Medium producers are all countries that produced more than 1% of Moving from final-to-primary energy requires electricity generation ef the world electricity in 1920 or 1970: Australia, Austria, China, Cze ficiencies for fossil fuels (coal, oil, natural gas) and renewables (e.g., choslovakia, France, India, Italy, Norway, Poland, South Africa, Spain, hydro). Sweden, and Switzerland. Fig. 4 shows the share of world electricity For Japan, UK, USA, and USSR (the largest producers) for production by these countries for the period 1900–1970. The classifi 1900–1970, fossil fuel electricity generation efficiencies were calculated cation of a country as a big, medium or small producer controlled the directly from available primary energy consumption and electricity amount of effort in gathering data for that country between 1900 and production data. For these countries, we had both primary and final 1970. More data were collected for large and medium countries. Fig. 4 energy so there was no need to use efficiencies to estimate primary shows that the maximum amount of electricity produced by all small energy. However, for Canada, Germany, and all medium producers, producers between 1900 and 1970 is 10%. primary-to-final efficiencies were obtained from Etemad et al. as Data for thermal and hydroelectricity production for France, Italy, primary energy data were not available. The same method was used for and Spain were found in the statistical yearbooks of each country and for small producers for 1900–1970, with electricity generation efficiencies Italy also in Malanima [47–50]. The primary reference for Spain, the for each energy source taken from Etemad et al. assuming that statistical yearbook includes only total production values, so hy these countries had the lowest efficiency recorded for each year. From droelectricity production until 1928 was estimated based on Rodríguez 1971 onwards, the IEA has data on primary energy for electricity. Missing values for hydroelectricity production in France prior to production for all countries. 1925 were estimated based on Bordes. For France, we deter For renewables, three options exist for estimating the equivalent mined the share of oil in thermal electricity for 1952 and natural primary energy source value: resource content method (RCM), physical gas share for 1957 and 1958. Together with data for 1960 from the content method (PCM) and partial substitution method (PSM). We IEA , we interpolated other years. In the case of Italy, for choose the most commonly used option: PCM, the method used by the 1925–1960, we identified the share of each fuel in thermal electricity IEA. In the PCM definition, primary energy is the first form of energy generation based on Castelli. that is commercially available, meaning wind and solar gross electricity Norway and Sweden data series started with high values of produced is considered primary energy , with no losses from primary-to-final energy stage. 1 Last, we convert from primary energy to primary exergy, via multi Germany data started with a high value of hydroelectricity production, suggesting hydroelectricity production began before 1920, but as no sources plication of exergy coefficients, shown in Table 1 [23,60]. were found for the period prior to 1920 we assume the share of Germany’s hydroelectricity prior to 1920 was equal to the average of the period 1920–1925. 3 R. Pinto et al. Energy 269 (2023) 126775 Fig. 1. Flow chart summarizing how primary, final and useful exergy were calculated. Red arrows: used only for pre-1971 calculations. Blue arrows: used for both pre- and post-1971 calculations. Fig. 2. Large and medium producers share of the world electricity production in 1920 and 1970, large producers’ countries that generate more than 5% of world electricity production, in 1920 or 1970, while medium producers are the countries that generate more than 1%. Fig. 3. Flow chart summarizing the countries which were allocated to three different groups, based on size of production share. 4 R. Pinto et al. Energy 269 (2023) 126775 Fig. 4. Share of world electricity production for 1900–1970 for large, medium, and small producers. equations in Table 2 or obtained from the literature [22,35]. Exergy Table 1 efficiencies for heating/cooling end-uses depend on the temperature of Exergy conversion factors per energy carrier. the surrounding environment and that required for the task. Different Energy carrier Exergy coefficient end-use temperatures were used depending on the end-use application. Coal and Coal products 1.06 Refrigeration and space cooling efficiencies were calculated by dividing Oil and Oil products 1.06 an average real Coefficient of Performance (COP) for machines in each Natural gas 1.04 year by the ideal COP shown in Table 2. For refrigerators, the ideal COP Combustible Renewables 1.11 Electricity 1.00 was calculated assuming that a third of the electricity was consumed by the freezer, at − 18 ◦ C, while the remaining two thirds were consumed by the cooler at 5 ◦ C, following Palma. The environmental tempera 2.3. Useful energy stage: Final-to-useful exergy ture was assumed to be 20 ◦ C. For space cooling, the ideal COP was calculated assuming a 25 ◦ C environmental temperature and 20 ◦ C To move from final exergy to useful exergy, we multiply by end-use end-use temperature. End-use temperatures for heating were taken as efficiencies. Useful exergy (also called useful work) is defined as “the 100 ◦ C for cooking, 60 ◦ C for water heating and 20 ◦ C for space heating. minimum amount of work (or exergy) required to produce a given en LTH exergy efficiencies were calculated assuming an energy efficiency, ergy transfer” [17, p.2]. Unfortunately, data in yearbooks and other ƞ, of 100% and a Carnot efficiency based on the end-use temperature statistical sources rarely allocates final energy to end-use tasks. Avail (T1) and environment temperature (T0). For cooking and water heating, able references sometimes allocate electricity to the sector or subsector T0 was taken as the average annual world temperature. For space in which it is consumed. Thus, the first step was collecting data for the heating, T0 was taken as the average world temperature for the coldest consumption of electricity in sectors and subsectors from multiple month of each year. HTH exergy efficiencies were calculated by multi sources for 1900–1971 [22,23,37–40,42,44,47,48,50,56,58,61–65]. plying the energy efficiency from Ayres et al. by the Carnot effi Detailed descriptions of country-level references are given in Table A1 of ciency from Table 2. T1 was assumed to be 500 ◦ C and T0 the average SI A. After 1971, sectoral electricity consumption is available from the world annual temperature. A table with the references for exergy effi IEA. Refer to Sections A2.1 and A2.2 of SI A for a more detailed ciency per end-use is available in section A2.4 of the SI A as well as more description of the methodology used for allocating electricity con details about real COP values. sumption to sectors and subsectors. The second step was the allocation to end-uses within each sector and subsector. We considered the following 10 end-uses: lighting, 2.4. Carbon intensity communication and electronics, electrochemical, high temperature heat (HTH), low temperature heat (LTH), cooling, transport, residential ap To estimate CO2 emissions, we used Intergovernmental Panel on pliances, commercial appliances, and machine tools and pumps. Allo Climate Change (IPCC) emission factors , which do not include the cation to end-uses was previously completed for Portugal and the USA life cycle emissions for each electricity production technology. Thus, [22,23]. Electricity consumption in residential and commercial sectors renewable technologies have emission factors equal to zero. Due to lack for the remaining countries was allocated to end-uses using Ayres et al. without modification. Electricity consumption in industrial sub Table 2 sectors was allocated to end-uses assuming one main end-use for each Cooling and heating exergy efficiencies (all formulas use temperature in subsector: HTH for iron and steel, electrochemical for the electro Kelvin (K)). T0 - environment temperature; T1 - end-use temperature; Tc – chemistry and electrometallurgy industries, and machine tools and Desired temperature of the freezer/cooler; ƞ - energy efficiency. Ideal pumps for other industries. All industrial subsectors have end-uses of COPs for the freezer, cooler and their combined average are 6.7, 18.5 and varying proportion among lighting, communication/electronics, and 14.6, respectively. cooling, with shares taken from the industrial sector of Ayres et al.. Ideal COP Cooling TC A more detailed description is available in section A2.3 of the SI A. T0 − TC Cooling exergy efficiency COPcooling, real The last step was the calculation of useful exergy via multiplying Ɛ = COPcooling, ideal end-use electricity consumption by associated final-to-useful exergy ef Heat exergy efficiency ( T0 ) Ɛ =ƞ 1− ficiencies. Final-to-useful exergy efficiencies were calculated with the T1 5 R. Pinto et al. Energy 269 (2023) 126775 of data, we do not take into account upstream emissions from fossil fuels. chain. In 2000, these emissions represented between 10% and 25% of the direct The sharp rise in the electrochemistry and electrometallurgy and emissions of a power plant, depending on the fuel and country consid iron and steel industries at the expense of other industries after 1971 is ered [67,68]. Thus, carbon intensity is the ratio of direct emission due to classifications of the IEA data. The IEA data contain a level of s/exergy, calculated at both the final stage (CIF) and the useful stage detail that enables allocation of a larger share of other industries to the (CIU). two subsectors, especially for the USSR. 3. Results 3.2.2. Allocation to end-uses Fig. 7 shows electricity consumption shares by individual end-uses. This section contains results obtained for electricity production and The share of lighting end-uses decreased throughout the period by consumption as well as efficiencies and carbon intensities associated more than half. Transport has decreased markedly (1/20th of the 1900 with electricity use. The data used to create each graph is available in SI share), because electric trams were replaced by automobiles. By 2017, B. This section is focused on overall trends while a detailed discussion of HTH end-uses were less than one third of their share in 1900. On the shorter-term trends is left to section 4. other hand, LTH and cooling end-uses have increased significantly, representing over 10% and 15% of total consumption, respectively, in 2017. By comparison in 1900, LTH had no share of electricity con 3.1. World electricity production sumption and cooling had less than 3% share. Communication and electronics and residential appliances end-uses shares experienced a Fig. 5 shows world shares of electricity production by energy source similar increase from less than 1% to close to 10%. Commercial appli (left axis) and total electricity production (right axis). Fossil fuels (in ances end-use share almost doubled to 5% in 2017. The share for ma grey scale colours) are responsible for a relatively stable fraction of chine tools and pumps has varied considerably. Looking only at 1900 electricity production (about 60%) from 1900 to 2017. Renewables, and 2017, it increased from 23% to 29%. Electrochemical end-uses have especially wind, have increased their share in the last decade. The fuel remained largely stable over the period (average ~4%). sources for electricity production have become more varied through time. 3.3. Electricity efficiencies 3.2. World electricity consumption 3.3.1. World primary-to-final exergy efficiencies for fossil fuels 3.2.1. Allocation to sector and subsector Fig. 8 shows the evolution of primary-to-final exergy efficiency for Fig. 6 shows the allocation of electricity consumption by subsector. fossil electricity generation. Average efficiency has grown over the last The share of electricity consumption in the transport sector decreased 117 years, mainly due to improvements in electricity generating tech significantly from 1900 (27.7%) to 2017 (1.7%). In contrast, con nology and with a smaller importance the increasing share of higher- sumption by residential and commercial sectors increased from a com efficiency generation. The sudden increase in oil efficiency in 1913 is bined share of 23.5% in 1900 to 48.4% in 2017. The sum of industry related to a change in source for Russia while the decrease in 1997 is due subsectors (iron and steel, electrochemistry and electrometallurgy, and to IEA data classifications. The jump in efficiency in 1971 is due to the other industries) decreased little from 1900 (47.3%) to 2017 (41.9%). switch in pre/post IEA datasets. Total electricity consumption in Fig. 6 (green line, right axis) shows a similar trend compared to Fig. 5, where total electricity production is 3.3.2. World primary-to-final, final-to-useful and primary-to-useful exergy shown - the differences are Fig. 6 excludes transmission losses and efficiencies electricity self-consumed by the energy industry. The average value of Fig. 9 shows primary-to-final, final-to-useful, and overall exergy ef transmission losses was 9.1% of electricity produced, while electricity ficiencies. As electricity production has been dominated by fossil fuel used in the energy industry was on average 8.6% of electricity produc sources since 1900, primary-to-final exergy efficiency follows a similar tion, see section A1.2 of the SI A. Both electricity production and con pattern to average fossil fuel exergy efficiency, Fig. 8. sumption are part of the final energy stage of the energy conversion Final-to-useful exergy efficiency remains surprisingly stable, within Fig. 5. World shares of electricity production per energy source (left axis) and total electricity production (right axis) (1 PWh = 1012 kWh = 3.6 EJ). 6 R. Pinto et al. Energy 269 (2023) 126775 Fig. 6. World shares of electricity consumption by sector and subsector (left axis) and total electricity consumption (right axis) (1 PWh = 1012 kWh = 3.6 EJ). Fig. 7. World shares of electricity consumption allocated per end-use. the range 40–50% over the whole period. In 1900, efficiency was 44% uses, as space heating reduced its importance while water heating and in 2017 it was approximately 47%. increased. The efficiency of cooling end-uses decreased between the In the period 1900–2017, primary-to-useful exergy efficiency grew mid-1950s and 1970 due to decreases in refrigeration efficiency because significantly reaching 17% in 2017. of increasing refrigerator and freezer size and new additional features. 3.3.3. Final-to-useful exergy efficiencies per end-use Fig. 10 shows final-to-useful exergy efficiencies for each end-use. The 3.3.4. Final-to-useful exergy efficiencies for each sector LTH line shows a decreasing efficiency trend, until the 1980’s, followed Fig. 11 shows the electricity efficiency for the different sectors and by a period of constant efficiency and more recently, after the early the aggregated final-to-useful efficiency. The industrial and transport 2000’s, a slow increasing trend is observed. This pattern is a result of the sectors have higher efficiencies than the global average. The transport variable shares of the different LTH uses. LTH is composed of three sector has mainly one end-use, transport end-use, which is a highly different uses: cooking, water heating, and space heating. A decrease in efficient end-use. Industry is mainly composed of HTH use, electro cooking use translated to diminishing share of LTH, whilst space heating chemical and machine tools and pumps end-uses. High efficiency ma and water heating weights increased. Water heating and (especially) chine tools and pumps have the largest share, leading to high efficiency space heating are less efficient than cooking, because of the lower for the industrial sector. The residential and commercial sectors had temperature of use, causing the decrease in overall LTH exergy effi distinct historical differences in terms of efficiency but have since ciency seen until the 1980s. The small increase in efficiency observed evolved into a similar sectoral final-to-useful efficiency. These two after the early 2000’s is again a result of a change in weights of LTH end- sectors have low efficiencies because of the significant share of low 7 R. Pinto et al. Energy 269 (2023) 126775 Fig. 8. Primary-to-final exergy efficiency for fossil fuel electricity production, between 1900 and 2017. Fig. 9. World electricity primary-to-useful, primary-to-final and final-to-useful exergy efficiencies, using the PCM method. Fig. 10. Final-to-useful exergy efficiencies for each electricity end-use, during 1900–2017. 8 R. Pinto et al. Energy 269 (2023) 126775 Fig. 11. Final-to-useful exergy efficiency of electricity use for different sectors. efficiency end-uses: lighting, LTH, cooling and communication and kg CO2/kWh while the CIU decreased from 13.18 to 1.24 kg CO2/kWh. electronics. Figures with allocation per end-use for each sector/subsector are 3.5. Annual growth rates available at section A4.1 of the SI A. The variability in efficiencies of the residential and commercial sectors are associated with fluctuations of Fig. 13 shows that there has never been a period when primary-to- individual end-use shares, which are retrieved directly from Ayres et al. useful exergy efficiency growth has led to CO2 emissions decline, since. the CO2 growth rate has never been negative. Fig. 13 also shows the lack of correlation between primary-to-useful exergy efficiency and CO2 emissions, especially after 1940. Primary-to-useful exergy efficiency 3.4. Carbon intensity and carbon dioxide emissions growth rate was close to zero, less than 0.5%/year, in the 1940s and between 1970 and 2010. The result holds for primary-to-final and final- Fig. 12 (right axis) shows the exponential growth of world CO2 to-useful exergy efficiencies, as shown in Figures A7 and A8 of SI A. emissions associated with electricity production for 1900–2017. Fig. 12 (left axis) also illustrates how carbon intensity has decreased during this 4. Discussion period. Two different metrics are shown: one considers carbon intensity at the final stage of the energy conversion chain (CIF) while the other In this section we will look in detail at the results shown above and takes one step forward and calculates carbon intensity at the useful level will go through them using a historical perspective first and then (CIU). Carbon intensity, both at the useful stage and final stage, has a zooming in to electricity efficiency and transitions. We will also discuss descending trend for 1900–2017 but both also exhibit stabilization since some limitations associated with this work. the 1980s, with slight decrease of carbon intensity in the last 5 years. Looking at the whole period 1900–2017, CIF dropped from 5.23 to 0.49 Fig. 12. CO2 emissions from electricity generation in grey (right axis), carbon intensity of final exergy in blue and useful exergy in orange (left axis), during the period 1900 to 2017. 9 R. Pinto et al. Energy 269 (2023) 126775 Fig. 13. Annual electricity production based growth rates of CO2 emissions and primary-to-useful exergy efficiency of electricity shown as 10-year moving averages (1910 represents the average annual growth rate between 1901 and 1910). 4.1. Historical perspective primary-to-final efficiency. Comparative results using PSM and RCM methods show similar results and can be seen in section A4.2 of the SI A. The construction of the world long-run electricity production and Throughout 1950–2017, residential and commercial sectors consumption database enables consideration of the evolution of elec increased their shares of electricity consumption, at the expense of the tricity production, consumption, and efficiency since the beginning of industrial sector (Fig. 6). These sectoral changes have impacted end-uses the 20th century. We see two distinct periods: 1900–1950 and as seen in the significant increase of the LTH end-use associated with the 1950–2017. increase of the residential and commercial sectors (Fig. 7). On the other Between 1900 and 1950, on average, over 95% of the world elec hand, the share of the machine tools and pumps end-uses decreased tricity was produced from coal and hydro sources, with approximately sharply due to the decrease in share of the industrial sector (Fig. 7). constant shares (coal representing 55% and hydro 40%) (Fig. 5). From Between 1950 and 2017, cooling end-use share grew only 3% but 1900 to 1950 primary-to-final exergy efficiency increased significantly, experienced a change in relative importance of its two constituents: the from 6% to 31%, caused by efficiency improvements in thermal power relative weight of refrigeration reduced, while space cooling grew. plants (blue line Fig. 9). During this period, the industrial sector Final-to-useful exergy efficiency varied between 46% and 50% during increased its share of electricity consumption at the expense of the this period but remained overall stable (orange line Fig. 9), due to ef transport sector (Fig. 6). Within the industrial sector, iron and steel ficiency dilution effects. Primary-to-useful exergy efficiency increased decreased its share more than 10% (Fig. 6). These sectoral changes in until 1960, caused by the increase in primary-to-final exergy efficiency fluence end-uses, with significant increases in the share of machine tools and stabilized afterwards (grey line Fig. 9). In 2017, carbon intensity and pumps and cooling end-uses, while end-uses for transport and HTH was almost half the 1950 value (orange and blue lines Fig. 12). CIF decreased their share significantly (Fig. 7). Final-to-useful exergy effi declined continuously until 1985. From 1950 to 1970, the decline in CIF ciency increased only slightly from 1900 (44%) to 1950 (47%) (orange is caused by growing primary-to-final efficiency while from 1971 to line Fig. 9), due to efficiency dilution effects. Final-to-useful exergy ef 1985, carbon intensity improvements are explained by an increase in the ficiency reached a peak in 1940 and declined thereafter (orange line share of electricity production with no CO2 emissions, mostly nuclear Fig. 9), this peak is associated with a peak in the share of the end-use power. Although carbon intensity, CIF and CIU, stabilized between 1985 machine tools and pumps. Primary-to-useful exergy efficiency, the and 2014 (orange and blue lines Fig. 12), electricity production was not product of both efficiencies, increased sharply (grey line Fig. 9), mostly flat (green line in Fig. 5). In fact, electricity production more than due to the increase of primary-to-final exergy efficiency. Carbon in doubled and therefore total electricity-based CO2 emissions also more tensity decreased to 0.85 kgCO2/kWh (CIF) and 2.13 kgCO2/kWh (CIU) than doubled, between 1985 and 2014. The most recent decline in respectively by 1950, less than 1/5th of their 1900 values (orange and carbon intensity (2014–2017) is caused by growth in wind and solar blue lines Fig. 12), mainly because of primary-to-final efficiency im electricity. provements. Nonetheless, CO2 emissions increased 24-fold during this period due to the near 150-fold increase in electricity production (grey line Fig. 12 and green line Fig. 5). 4.2. Electricity efficiency In the second period (1950–2017), electricity production from nu clear, oil, and (more recently) natural gas sources rose in prominence End-use efficiency (i.e. more energy efficient cars, lights, heating (Fig. 5). Whilst in the last decade (2007–2017) solar and wind have etc.) is commonly assumed to be a key driver of (primary and final) become more important, their combined share remains less than 10% energy reductions (and associated carbon emissions) in future scenarios (Fig. 5). Primary-to-final exergy efficiency increased significantly in the [2,3,13]. In contrast, our results show that: (1) individual exergy effi 1950–1960 decade, due to efficiency improvements in thermal power ciencies grew throughout 1900–2017 (with the exception of LTH and plants, and stabilized until 1970 (blue line Fig. 9). After an increase in cooling) due to technological evolution, but aggregate primary-to-useful the early 1970s, primary-to-final exergy efficiency stalled during the exergy efficiency gains were significant only until mid 1930s (pri following 40 years, rising slightly after 2005 (blue line Fig. 9) because of mary-to-useful exergy efficiency grew 10% from 1900 until 1935 and increasing (a) fossil fuel thermal powerplant efficiency and (b) share of only 4% in the period 1935–2017) and (2) there is no obvious correla solar/wind based electricity – which via the PCM method assumes 100% tion between aggregate primary-to-useful exergy efficiency, electricity consumption and CO2 emissions (Fig. 13). 10 R. Pinto et al. Energy 269 (2023) 126775 Two different stories have unfolded: primary-to-final exergy effi shows that transition has been slow and too small to reduce electricity- ciency increased by a factor of 7, mainly in the period 1900–1960, and related CO2 emissions, which have increased almost every year. On stayed quite stable thereafter. Final-to-useful exergy efficiency increased average across 1900–2017, 60% of electricity was produced using fossil only slightly during the whole period from 44% (1900) to 47% (2017). fuels (Fig. 5). There has been slowly increasing share of oil-based elec This near stagnation was due to efficiency dilution, where growing de tricity in the 1960s and early 1970s, nuclear electricity in the 1970s and mand for less efficient end-uses (LTH and cooling) in residential and 1980s, and natural gas based electricity in the 1990s until 2010. commercial sectors offset efficiency gains of end-use devices. Recently, there is a small increase in the share of renewables. Whilst To our knowledge, this study is the first to estimate world electricity moving through decreasing carbon intensities of fuels (coal-oil-nuclear- production and consumption efficiency over a long period. Other studies gas and now to renewables) are positive steps, past transitions have not have looked to specific countries for shorter time periods. The results been sufficiently large to reduce electricity-related CO2 emissions, obtained for final-to-useful exergy efficiency for the USA in Ayres et al. which have increased almost every year (grey line Fig. 12). also show that final-to-useful exergy efficiency remained approxi Carbon intensity of electricity is an insufficient metric to assess mately constant during 1900–2000, due to the increase in LTH end-uses. transitions if the goal is reducing CO2 emissions. During 1900–2017, Felício et al. show Portugal’s final-to-useful efficiencies are similar world carbon intensity dropped by around 90% (orange and blue lines in 1900 and 2014. Between those two years there is variation but, in the Fig. 12), a result similar to Felício et al. results for Portugal. Ang beginning, (1900), and in the end (2014) of the period final-to-useful and Su found similar results at the world level 1990–2013 for efficiencies are both close to 30%. However, while world carbon intensity at the final stage. However, carbon emissions increased final-to-useful exergy efficiency has remained constant since 2000, 380-fold since 1900, as electricity production had a much larger Portugal’s show a continuous decrease, due to the growth of the resi (4000-fold) increase since 1900. Historically, rising demand for elec dential sector share. Our world results do not show a decrease in tricity (driven by economic growth and electrification of end-uses) has primary-to-final exergy efficiency as found in the the long-term study for always outstripped the capability of efficiency to reduce CO2 emissions. Japan , because hydro was not as significant at the world level as in In 2017, carbon intensity of electricity production was 0.49 kgCO2/ Japan. Regarding primary-to-useful exergy efficiency, we estimate an kWh. Using the IRENA scenario for 2050 a carbon intensity of 0.10 increase of 1% for 1960–2010, in line with the result for China , kgCO2/kWh is required, implying a further reduction of 0.39 USA, and UK. kgCO2/kWh - a fifth of its current value, in less than 40 years. When we Global electricity consumption is expected to rise from 19% in 2022 compare to the decrease of 0.12 kgCO2/kWh between 1977 and 2017, to more than 40% of total final energy consumption by 2050 [2,3]. The the tremendous challenge ahead is obvious. The necessary speed of increase of electrification may contribute to an increase in the aggregate decrease of carbon intensity to meet Paris objectives is unprecedented. final-to-useful exergy efficiency, because electricity end-uses typically have higher final-to-useful exergy efficiencies compared to other 4.4. Limitations end-uses. Additionally, the mix of end-uses provided by electricity will change significantly which might push the electricity final-to-useful ef Final-to-useful exergy efficiency depends on the allocation to sectors, ficiency upwards if the increase in the electrification of transport dom and it also depends on the allocation to end-uses within the sectors. inates the transition. Regarding allocation to sectors, IEA data were available after 1971 , while before 1971 information for various countries was collected as 4.3. Transitions described in sections 2.3 of this paper and A2.1 of SI A. Considering allocation to end-uses within each sector and subsector, we assumed the The time series of world electricity production and consumption USA as a proxy, except for the industrial sector where we used the provides insight into future energy transitions, in particular electrifica allocation to subsectors that were country-specific. For the residential tion, renewables, and efficiency as potential drivers of decarbonization. and commercial sectors, we used USA data to allocate sectoral con In the future, electrification of end-uses will create structural changes to sumption to end-uses. Although the USA is unlikely to be representative electricity consumption, with an increase in the share of electricity of the sectoral electricity consumption patterns in every country, errors consumption for the transport sector. Indeed, the transport sector share associated with this assumption are minimized at the world level, is expected to reach over 20% of total electricity consumption by 2050, because the USA consumes a large share (36%) of world electricity while commercial and residential sectors are expected to decrease their production on average over the time period of this study. share. Under those assumptions, aggregate world final-to-useful One way to estimate the uncertainty of our results is to undertake a exergy efficiency would increase because the transport sector is ex full sensitivity analysis, as for example done by Paoli et al. but the pected to remain more efficient than the commercial and residential necessary data are not available, as in the past the uncertainty estimates sectors (Fig. 11). were not provided with statistics. Paoli et al. state that “the main The IEA forecasts predict that electricity production will double source of uncertainty is found in the allocation to end-use applications”. between 2020 and 2050. The past increase in electricity production, Therefore, to provide a check on the sensitivity of our results regarding which doubled between 1990 and 2017 suggests that the IEA scenario is the allocation to end-uses within the residential sector, we replaced al feasible. In contrast, Jacobson et al. states that if we transition locations for every country except the US with allocations for Portugal totally to electricity by 2050, electrify all end-uses currently using fossil. The results obtained were not significantly different: an average fuels, we will reduce final energy demand by more than 50%, compared final-to-useful exergy efficiency of 47.1% compared to 48.4% and a to a business-as-usual scenario but tripling electricity consumption be similar trend. The differences between the two final-to-useful exergy tween 2020 and 2050 would require an increase in electricity produc efficiency curves can be seen in section A4.4 of the SI A. tion higher than observed in the past. Other possible sources of uncertainty are the final-to-useful indi But will electrification and efficiency be enough to meet decarbonization vidual exergy efficiencies. To evaluate the sensitivity of our study, we needed? Fig. 13 shows efficiency alone has never been sufficient to changed the efficiency of the end-use with the largest share (see Fig. 7), decrease CO2 emissions, with no observed historical correlation between machine tools and pumps. We changed the efficiency of machine tool rising primary-to-useful exergy efficiency and CO2 emissions decline and pumps by 10% (for example in 1900 the efficiency went from 75% (Fig. 13). Our results suggest electrification and efficiency must be to 82.5%) and obtained an average aggregate final-to-useful exergy ef linked with a deep renewables transition if decarbonization is to occur. ficiency of 48.4% ± 2.8%. Additionally, this change did not affect the We know that a large-scale transition to renewables must happen overall trend of stagnation, see section A4.5 of the SI A for the quickly (10–20 years) to meet Paris climate objectives. However, Fig. 5 comparison. 11 R. Pinto et al. Energy 269 (2023) 126775 This paper is only focused on electricity which means that our results and heating services. If so, will climate policies be sufficient to drive a do not quantify the evolution of overall efficiency (efficiency consid supply-side transition to renewable sources for electricity generation at ering all final energy sources including oil, coal, etc.) and are insufficient sufficient speed to keep pace with demand-side electrification? Our re to evaluate the impact of switching from other fuels to electricity. sults show that the rate of increase in electricity production between 1900 and 2017 was 7.4%/year. However, between 1990 and 2017 the 5. Conclusions rate of increase in electricity production was 2.9%/year which is higher than the rate needed for the electrification of the world energy system This work produced a novel long-run 1900–2017 global electricity under IEA net zero emission scenario , 1.8%/year, but lower than the dataset that relates primary energy sources to final and useful energy rate of increase of electricity production necessary to reach nearly total flows. We presented new figures for electricity production and con electrification as described in Jacobson et al. , which is 3.4%/year, sumption at a sectoral level, primary to final and final to useful exergy and much lower than the rate of increase outlined in Ram et al. , efficiencies and final and useful carbon intensities of electricity. The 5.2%/year. Additionally, the fastest transitions in the electricity gener most striking finding is that world electricity energy efficiency ation mix were the increase in fossil fuel share in the 1950s and 1960s (measured as overall primary-to-useful exergy efficiency) has stalled, and the increase in nuclear share in the 1960s and the 1970s, none of rising dramatically from 2% in 1900 to 15% in 1960, and remaining which exceeded 1%/year. The transition to non-hydro renewables has nearly stable for the last 50 years, only reaching 17% by 2017. The rapid been significant after the mid-2000s, rising from 0.6% to more than 6% efficiency gain in the 1900–1960 period was due to the 6-fold rise (from of total electricity production, however the increases in share have not 6% to 36%) in primary-to-final electricity efficiency (mainly due to exceeded 1%/year, highlighting the need for a massive investment in improvements in power station efficiency), as final-to-useful exergy ef renewables and a significant decrease in new fossil fuel generation. Both ficiency was stable, with an average of 48%, over 1900–2017. In the last the electrification of end-uses and the transition to renewables are 50 years, the stagnation of both electricity generation (primary-to-final) required to meet Paris targets. The results presented above show that exergy efficiency and end use (final-to-useful) exergy efficiency means doing both at sufficient speed will be unprecedented. that the overall (primary-to-useful) electricity efficiency has remained stable (15–17%) since 1960. Carbon intensities decreased significantly Credit author statement until 1960, mainly due to increases in power station (primary-to-final) energy conversion efficiency. In 2017, carbon intensities at the final and Ricardo Pinto: Conceptualization, Methodology, Formal analysis, useful energy stages were less than 10% their 1900 values. However, to Investigation, Data curation, Writing – original draft, Visualization. reach climate goals, a further decrease in carbon intensity to 20% of Sofia T. Henriques: Conceptualization, Methodology, Investigation, current values (2% of 1900 values) is necessary before 2050. Writing – review & editing. Paul E. Brockway: Conceptualization, The cause of the final-to-useful exergy efficiency stagnation in the Methodology, Writing – review & editing, Supervision. Matthew Kupe past is efficiency dilution caused by structural transitions and end-use rus Heun: Conceptualization, Methodology, Writing – review & editing. changes, such as the increasing share of low-temperature heating Tânia Sousa: Conceptualization, Methodology, Writing – review & caused by the increasing share of overall electricity consumption in the editing, Supervision, Project administration. residential and commercial sectors and the decreasing importance of static mechanical work associated with the decrease in the share of the Declaration of competing interest industrial sector. The empirical result shows that final-to-useful effi ciency has stalled because it has been significantly dependent on the The authors declare that they have no known competing financial type of end-uses that were electrified, suggesting that future energy ef interests or personal relationships that could have appeared to influence ficiency may be mostly dictated by the mix of end-uses. The result em the work reported in this paper. phasizes that technological developments alone will not be the only factor in changing aggregated electricity efficiency. Instead, shares of Data availability key electrified technologies (transport, low-temperature heat and ICT) in electricity consumption will have significant impacts on changing SI B contains the data used to build the graphs displayed in this aggregate electricity efficiencies upwards or downwards. Whether paper. Any need for more detailed data will be made available on overall efficiency increases or decreases will depend on technological request. and policy choices and development patterns. For example, a crucial question is whether developing and emerging economies will follow the Acknowledgments path of their forerunners. If developing economies become more service based and expansion of household low-temperature heat and cooling The authors are grateful to Zeke Marshall for the help with cooling applications continue, we may expect end-use efficiencies to drop. On data and efficiency; to Roger Fouquet for the help with UK data; and to the other hand, if developing economies leapfrog to electric cars and Laura Felício and Tiago Domingos for their insightful suggestions. avoid pollution intensive modes of transportation, end-use efficiencies Ricardo Pinto’s work was supported by Fundação para a Ciência e may increase and a brighter future might emerge. Our results show that Tecnologia through the individual research grant SFRH/BD/146923/ there is a need for a deeper investigation of the full impact of electrifi 2019. Paul Brockway’s time was funded by the UK Research and Inno cation on world energy efficiency. A follow-on study that includes the vation (UKRI) Council, supported under EPSRC Fellowship award EP/ remaining energy carriers (coal, oil, natural gas and biomass) will R024254/1. Sofia Henriques acknowledges the generous support of enable comparisons among electricity and other energy carriers’ effi Handelsbanken and Crafoord foundation for the projects “Energy use ciencies by end-uses and forecasts of the full impact of electrification on and economic growth: a long-run European study 1870–2013”. overall efficiency due to the forthcoming energy transition. Overall, our study raises crucial questions for future renewable Appendix A. Supplementary data electricity transitions. It is known that the key drivers of previous transitions have been cheaper and/or better energy services. (E.g., The supplementary information is divided in two documents. The electricity-altered production processes allowed radical reconfigura first is a word document entitled “SI A Methodology and supplementary tions of the factory floor.) It seems fair to ask whether electrifica results” where details about the methodology and some additional re tion of transport and heating end uses could be driven similarly at sults are presented. The second is an excel document entitled “SI B data” sufficient speed by a demand-side clamour for improved transportation and contains the data used to build the graphs displayed in this paper. 12 R. Pinto et al. Energy 269 (2023) 126775 Supplementary data to this article can be found online at https://doi.org Sousa T, Brockway PE, Cullen JM, Miller J, Cabrera A, Domingos T. The need for robust, consistent methods in societal exergy accounting. Ecol Econ 2017;141: /10.1016/j.energy.2023.126775. 11–21. https://doi.org/10.1016/j.ecolecon.2017.05.020. Cullen JM, Allwood JM. The efficient use of energy: tracing the global flow of References energy from fuel to service. Energy Pol 2010;38:75–81. https://doi.org/10.1016/j. enpol.2009.08.054. De Stercke S. Dynamics of energy systems: a useful perspective. IIASA Interim Nakićenović N, Gilli PV, Kurz R. Regional and global exergy and energy Report,IIASA; 2014. efficiencies. Energy 1996;21:223–37. https://doi.org/10.1016/0360-5442(96) IEA (International Energy Agency). Net zero by 2050. 2021. Paris. 00001-1. International Renewable Energy Agency (IRENA). Global energy transformation: a Palma M, Sousa T, Guevara Z. How much detail should we use to compute societal roadmap to 2050. 2018. aggregated exergy efficiencies? Energies 2016;9. https://doi.org/10.3390/ IEA (International Energy Agency). World energy outlook 2018. 2018. en9050364. Goh T, Ang BW, Su B, Wang H. Drivers of stagnating global carbon intensity of Etemad B, Luciani J, Bairoch P, Toutain J-C. World energy production. electricity and the way forward. Energy Pol 2018;113:149–56. https://doi.org/ Switzerland: DROZ; 1991. 10.1016/j.enpol.2017.10.058. International Energy Agency (IEA). Extended world energy balances. IEA World Rogelj J, Luderer G, Pietzcker RC, Kriegler E, Schaeffer M, Krey V, et al. Energy Energy Statistics and Balances (Database); 2018. https://doi.org/10.1787/data- system transformations for limiting end-of-century warming to below 1.5 ◦ C. Nat 00513-en. Clim Change 2015;5:519–27. https://doi.org/10.1038/nclimate2572. Heun MK, Marshall Z, Aramendia E, Brockway PE. The energy and exergy of light IEA, International Renewable Energy Agency (IRENA), UNSD, World Bank. WHO. with application to societal exergy analysis. Energies 2020;13:1–24. https://doi. Tracking SDG 7: the energy progress report 2020. 2020. Washington DC. org/10.3390/en13205489. Brockway PE, Sorrell S, Semieniuk G, Heun MK, Court V. Energy efficiency and Dominion Bureau of Statistics. The Canada year book. 1925-1977. 1925. economy-wide rebound effects: a review of the evidence and its implications. Statistischen reichsamt. Statistisches Jahrbuch für das Deutsche Reich. 1928-1941. Renew Sustain Energy Rev 2021;141:110781. https://doi.org/10.1016/j. 1928. rser.2021.110781. Staatlichen zentralverwaltung für statistik. Statistisches jahrbuch der Deutschen Santos J, Domingos T, Sousa T, st Aubyn M. Useful exergy is key in obtaining demokratischen republik. 1955-1971. 1955. plausible aggregate production functions and recognizing the role of energy in Statistisches bundesamt. Statistisches Jahrbuch für die Bundesrepublik economic growth: Portugal 1960–2009. Ecol Econ 2018;148:103–20. https://doi. Deutschland. 1953-1972. 1953. org/10.1016/j.ecolecon.2018.01.008. Japan Statistics Association Historical statistics of Japan, 1868-1984. https://warp. Herring H. Energy efficiency - a critical view. Energy 2006;31:10–20. https://doi. da.ndl.go.jp/info:ndljp/pid/11423429/www.stat.go.jp/english/data/chouki/10. org/10.1016/j.energy.2004.04.055. html. [Accessed 17 February 2020]. Waite M, Cohen E, Torbey H, Piccirilli M, Tian Y, Modi V. Global trends in urban Mitchell BR. European historical statistics 1750–2010. London: MacMillan; 2013. electricity demands for cooling and heating. Energy 2017;127:786–802. https:// Department of Energy & Climate Change (DECC). Historical electricity data: 1920 doi.org/10.1016/j.energy.2017.03.095. to 2019. https://www.gov.uk/government/statistical-data-sets/historical-electricit Ram MA, Bogdanov D, Aghahosseini A, Gulagi A, Oyewo SA, Child M, et al. Global y-data. [Accessed 22 January 2020]. energy system based on 100% renewable energy - power, heat, transport and United States Bureau of the Census. Historical statistics of the United States, desalination. Berlin: Lappeenranta; 2019. colonial times to 1970. Bicentennial Ed. Washington, DC: United States Jacobson MZ, Delucchi MA, Cameron MA, Coughlin SJ, Hay CA, Manogaran IP, Government Printing Office; 1975. et al. Impacts of green new deal energy plans on grid stability, costs, jobs, health, U.S.S.R Commitee for International Scientific and Technical Conferences. Electrical and climate in 143 countries. One Earth 2019;1:449–63. https://doi.org/10.1016/ power development in the USSR. INBA publishing society; 1936. j.oneear.2019.12.003. National Foreign Assessment Center. USSR: development of the gas industry. A Teske S. Achieving the paris climate agreement goals: global and regional 100% research paper. The center; 1978. renewable energy scenarios with non-energy GHG pathways for +1.5◦ C and +2◦ C. Wilson D. The demand for energy in soviet union. Rowman & Allanheld; 1983. Springer International Publishing; 2019. https://doi.org/10.1007/978-3-030- Institut national de la statistique et des Études Économiques. Annuaire statistique 05843-2. de la France; 1966. Van Heddeghem W, Lambert S, Lannoo B, Colle D, Pickavet M, Demeester P. Instituto Nacional Estadistica. Anuario estadistico de España. 1930-1961. 1930. Trends in worldwide ICT electricity consumption from 2007 to 2012. Comput Malanima P. Energy consumption in Italy in the 19th and 20th centuries: a Commun 2014;50:64–76. https://doi.org/10.1016/j.comcom.2014.02.008. statistical outline. 2006. Reistad GM. Available energy conversion and utilization in the United States. Italian National Institute of Statistics. Gross electricity production and final Journal of Engineering for Power 1975;97:429–34. https://doi.org/10.1115/ electricity consumption in Italy - years 1883-2014. http://seriestoriche.istat.it/ 1.3446026. index.php?id=1&no_cache=1&L=1&no_cache=1&tx_usercento_centofe%5Bcate Serrenho AC, Warr B, Sousa T, Ayres RU, Domingos T. Structure and dynamics of goria%5D=31&tx_usercento_centofe%5Baction%5D=show&tx_usercento_centofe useful work along the agriculture-industry-services transition: Portugal from 1856 %5Bcontroller%5D=Categoria&cHash=579686ba1e4b0850cf494bfb15f55d77. to 2009. Struct Change Econ Dynam 2016;36:1–21. https://doi.org/10.1016/j. [Accessed 14 March 2020]. strueco.2015.10.004. Isabel Bartolomé Rodríguez. La Industria Eléctrica en España (1890- 1936). Serrenho AC, Sousa T, Warr B, Ayres RU, Domingos T. Decomposition of useful Estudios de Historia Económica - Banco de España 2007;50:165. work intensity: the EU (European Union)-15 countries from 1960 to 2009. Energy Bordes J. Les barrages en France du XVIII è à la fin du XX è siècle Histoire , 2014;76:704–15. https://doi.org/10.1016/j.energy.2014.08.068. évolution technique et transmission du savoir. 1994. p. 70–120. Ferguson R, Wilkinson W, Hill R. Electricity use and economic development. Organisation for European Economic Co-operation (OEEC). The electricity supply Energy Pol 2000;28:923–34. https://doi.org/10.1016/S0301-4215(00)00081-1. industry in europe. 1955. Ayres RU, Ayres LW, Warr B. Exergy, power and work in the US economy, 1900 – Castelli F. Developments in the use of fuels for thermal power generation in Italy. 1998. Energy 2003;28:219–73. https://doi.org/10.1016/S0360-5442(02)00089-0. Sixth world Power Conference; 1962. Heun MK, Brockway PE. Meeting 2030 primary energy and economic growth Norwegian Water Resources and Energy Directorate. Utbygd vannkraft i Norge. goals: mission impossible? Appl Energy 2019;251:112697. https://doi.org/ Oslo: Norges Vassdrags- Og Elektrisitetsforening; 1946. 10.1016/j.apenergy.2019.01.255. Statistisk Sentralbyra. Statistisk årbok for norge. 1940-1970. 1940. Ayres RU, Ayres LW, Pokrovsky V. On the efficiency of US electricity usage since Kander A, Malanima P, Warde P. Power to the people: energy in europe over the 1900. Energy 2005;30:1092–145. https://doi.org/10.1016/j.energy.2004.07.012. last five centuries. 2013. https://doi.org/10.1017/S0022050715001011. Felício L, Henriques ST, Domingos T, Serrenho AC, Sousa T. Insights from past Bureau of census and statistics. Union Statistics for fifty years. Pretoria; 1960. trends in exergy efficiency and carbon intensity of electricity: Portugal, Rubio MDM, Tafunell X. Latin American hydropower: a century of uneven 1900–2014. Energies (Basel); 2019. https://doi.org/10.3390/en12030534. evolution. Renew Sustain Energy Rev 2014;38:323–34. https://doi.org/10.1016/j. Guevara Z, Sousa T, Domingos T. Insights on energy transitions in Mexico from the rser.2014.05.068. analysis of useful exergy 1971–2009, vol. 9. Energies (Basel); 2016. https://doi. Ertesvåg IS, Mielnik M. Exergy analysis of the Norwegian society. Energy 2000;25: org/10.3390/en9070488. 957–73. https://doi.org/10.1016/S0360-5442(00)00025-6. Williams E, Warr B, Ayres RU. Efficiency dilution: long-term exergy conversion World Power Conference. Statistical year book of the world power conference trends in Japan. Environ Sci Technol 2008;42:4964–70. https://doi.org/10.1021/ No.8. 1956. es0716756. World Power Conference. Statistical year book of the world power conference No. Brockway PE, Barrett JR, Foxon TJ, Steinberger JK. Divergence of trends in US and 9. 1960. UK aggregate exergy efficiencies 1960-2010. Environ Sci Technol 2014;48: Urquhart MC, Buckley KAH. Historical statistics Canada.pdf. 1965. 9874–81. https://doi.org/10.1021/es501217t. Statistiska centralbyrån. Stockholm: Statistisk årsbok för Sverige; 1925. Eisenmenger N, Warr B, Magerl A. Trends in Austrian resource efficiency: an Union Internationale des Producteurs et Distributeurs D’Énergie Électrique exergy and useful work analysis in comparison to material use, CO 2 emissions, and (UNIPEDE). Statistiques. (Several issues) years between 1937 and 1952. 1937. land use. J Ind Ecol 2017;21:1250–61. https://doi.org/10.1111/jiec.12474. Eggleston HS, Buendia L, Miwa K, Ngara T, Tanabe K. IPCC guidelines for national Brockway PE, Steinberger JK, Barrett JR, Foxon TJ. Understanding China’s past greenhouse gas inventories, ume 2. Energy. Japan: Institute for Global and future energy demand: an exergy efficiency and decomposition analysis. Appl Environmental Strategies; 2006. Energy 2015;155:892–903. https://doi.org/10.1016/j.apenergy.2015.05.082. Dones R, Heck T, Hirschberg S. Greenhouse gas emissions from energy systems, comparison and overview. Encyclopedia of Energy 2004;77–95. https://doi.org/ 10.1016/B0-12-176480-X/00397-1. 13 R. Pinto et al. Energy 269 (2023) 126775 Weisser D. A guide to life-cycle greenhouse gas (GHG) emissions from electric Paoli L, Lupton RC, Cullen JM. Probabilistic model allocating primary energy to supply technologies. Energy 2007;32:1543–59. https://doi.org/10.1016/j. end-use devices. Energy Proc 2017;142. https://doi.org/10.1016/j. energy.2007.01.008. egypro.2017.12.180. Dahmus JB. Can efficiency improvements reduce resource consumption? A Fouquet R. The slow search for solutions: lessons from historical energy transitions historical analysis of ten activities. J Ind Ecol 2014;18:883–97. https://doi.org/ by sector and service. Energy Pol 2010;38:6586–96. https://doi.org/10.1016/j. 10.1111/jiec.12110. enpol.2010.06.029. Ang BW, Su B. Carbon emission intensity in electricity production: a global Marshall ZHM, Brockway PE, Aramendia E, Steenwyk P, Relph T, Widjanarko M, analysis. Energy Pol 2016;94:56–63. https://doi.org/10.1016/j. et al. A Multi-Regional Primary-Final-Useful (MR-PFU) energy and exergy database enpol.2016.03.038. v1.0, 1960-2020. 2023. https://doi.org/10.5518/1199 [Dataset]. 14