Unit 7 - Electrochemistry PDF
Document Details
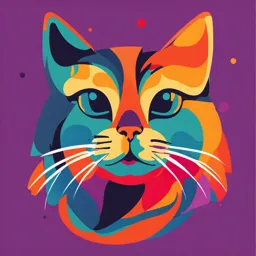
Uploaded by ShinyDahlia
Tags
Summary
This document provides an overview of electrochemistry and redox reactions. It begins by defining oxidation and reduction and moves on to concepts like the electronic concept of oxidation and reduction. The document also covers topics such as oxidation number, half-reaction and complete reactions.
Full Transcript
# UNIT 7 - ELECTROCHEMISTRY ## Topics Covered: 1. Simple oxidation - reduction reactions in a beaker * Direct redox reaction * Indirect redox reaction 2. Electrochemical cell- working and description, representation of an electrochemical cell calculation of Eº cell for galvanic cells. Eg...
# UNIT 7 - ELECTROCHEMISTRY ## Topics Covered: 1. Simple oxidation - reduction reactions in a beaker * Direct redox reaction * Indirect redox reaction 2. Electrochemical cell- working and description, representation of an electrochemical cell calculation of Eº cell for galvanic cells. Eg: Daniel cell 3. Predicting the feasibility of redox reaction 4. Non-Spontaneous reaction- electrolytic cell. 5. Corrosion of iron - Conditions and prevention of corrosion. # REDUCTION AND OXIDATION According to the classical concept, oxidation is a process of addition of oxygen or removal of hydrogen, and reduction is a process of removal of oxygen or addition of hydrogen. ## Examples of oxidation-reduction reactions * **Reaction of PbO and carbon:** PbO + C → Pb + CO Here oxygen is being removed from PbO and is being added to carbon. Thus PbO is reduced while C is oxidized. * **Reaction of H2S and Cl2:** Cl2 + H2S → 2HCl + S Here hydrogen is being removed from hydrogen sulphide and is being added to chlorine. Thus H2S is oxidized and Cl2 is reduced. # ELECTRONIC CONCEPT OF OXIDATION AND REDUCTION Oxidation is a process in which an atom or a group of atoms taking part in a chemical reaction loses one or more electrons. For example: | Reactant | Product | |-----------------------|-----------------------| | MnO4^2- (Manganate) | MnO4^1- (Permanganate) | | Fe^2+ (Ferrous) | Fe^3+ (Ferric) | | Cu (Copper) | Cu^2+ (Cupric) | The species that undergo the loss of electrons during the reactions are called **reducing agents**. MnO4^2-, Fe^2+ and Cu are reducing agents in the above examples. ## Reduction Reduction is a process in which an atom or a group of atoms taking part in a chemical reaction gains one or more electrons. For example: | Reactant | Product | |----------------|---------| | Ag^+ | Ag | | Fe^3+ | Fe^2+ | The species which undergo gain of electrons during the reactions are called **oxidizing agents**. In the above examples Ag+, Fe^3+ are oxidizing agents. # Simultaneous Occurrence of Oxidation and Reduction Since oxidation involves loss of electrons and reduction involves gain of electrons, it is evident that if one substance loses electrons, another substance at the same time must gain electrons because electrons cannot be the products in any chemical change. This means that in any process oxidation can occur only if reduction is also taking place side by side. Both the processes are complementary. Hence chemical reactions involving both reduction and oxidation are called **redox reactions**. ## Summary: * **Oxidation:** Loss of electrons (Species that undergoes oxidation is the reducing agent) * **Reduction:** Gain of electrons (Species that undergoes reduction is the oxidizing agent) # OXIDATION HALF AND REDUCTION HALF REACTIONS Every redox reaction can be split up into two half reactions: * **Oxidation half-reaction:** Representing loss of electrons. * **Reduction half-reaction:** Representing gain of electrons. ## Examples: * **Example 1:** Zn + Cu^2+ → Zn^2+ + Cu * Zn - 2e → Zn^2+ (Oxidation half-reaction) * Cu^2+ + 2e → Cu (Reduction half-reaction) * **Example 2:** Sn^2+ + 2Hg^2+ → Sn^4+ + Hg2^2+ * Sn^2+ - 2e → Sn^4+ (Oxidation half-reaction) * 2Hg^2+ + 2e- → Hg2^2+ (Reduction half-reaction) # Oxidation Number or Oxidation State We have learnt that redox reactions involve the transference of electrons from one species to another. The transference of electrons can be easily understood in case of ionic species because the charge on them also changes during redox reactions. However, the redox changes in case of covalent compounds cannot be easily explained in terms of transference of electrons. For example, in a chemical reaction between zinc and copper ions, it is quite relevant that zinc atoms lose electrons and Cu^2+ ions gain electrons. Zn(s) + Cu^2+ (aq) → Zn^2+ (aq) + Cu (s) But in a chemical reaction between Hydrogen and Chlorine, the loss and gain of electrons cannot be easily explained. Thus, in order to explain transference of electrons in either of the species in a more convenient way, the concept of **oxidation number** has been introduced. H2(g) + Cl2(g) → 2HCl(g) ## Determining Oxidation Number **Oxidation Number (O.N.)** of the elements is defined as the residual charge, which its atom has or appears to have when all other atoms from the molecule are removed as ions. During the removal of atoms, the electrons are counted according to the following fundamental rules: 1. **Electrons shared between 2 similar atoms are divided equally between the sharing atoms.** For example, in chlorine molecule the electron pair is equally shared between the two chlorine atoms [Cl-Cl]. Therefore, one electron is counted with each chlorine. Now there is no net charge on each atom of chlorine. In other words the oxidation number of chlorine in chlorine molecule is 0. 2. **Electrons shared between 2 dissimilar atoms are counted with the more electronegative atom.** For example, in hydrogen chloride molecule, chlorine molecule is more electronegative than hydrogen. Therefore, the shared pair of electrons is counted towards the chlorine atom. As a result, chlorine gets an extra electron and acquires a unit negative charge. Hence, the oxidation number of chlorine is -1. On the other hand, without electron, hydrogen has a unit positive charge and the oxidation number hydrogen is +1. 3. **Thus, atoms can have positive, zero or negative values of oxidation number depending upon their state of combination. In fact, oxidation number is the charge assigned to the atom in a species according to some arbitrary rules as given below:-** 4. **The oxidation number of an element in the free or chemical state is always zero.** For example, oxidation numbers of He in He, Cl in Cl2, S in S8, P in P4 are zero. 5. **The oxidation number of the element, which forms monoatomic ion, is equal to the charge on the ion.** For example, the oxidation number of K is +1 while that of Cl is -1. In the same way, the oxidation number of alkali metals is +1 and that of alkaline earth metals is +2. 6. **The oxidation number of fluorine is always -1 in all compounds. Oxygen is assigned oxidation number -2 in most of the compounds. However in peroxides like H2O2, Ba2O2, Na2O2 etc. its oxidation number is -1. Similarly the exception also occurs in compounds of fluorine and oxygen like OF2 and O2F2 in which the oxidation number of oxygen is +2 and +1 respectively.** 7. **The algebraic sum of the oxidation number of all the atoms in a neutral molecule is zero. But in case of a compound ion the sum of the oxidation number of all its atoms is equal to the charge on the compound ion.** # Example: Calculate the oxidation number of sulphur in a) H2S2O7 and b) HSO3¯ **Solution:** **a) Let the oxidation number of S be x**. Since it is a neutral molecule: (+1 × 2) + 2x + (-2 × 7) = 0 or x = +6 **b) Let the oxidation number of S be x**. Since it is a polyatomic ion: (+1) + x + (-2 × 3) = -1 or x = +4 # Calculate the oxidation number of the element in bold, in the following: 1. H2S 2. HSO4 3. NaClO3 4. ClO4 5. K2MnO4 6. K2Cr2O7 7. SO4^2- 8. HPO4 9. C6H12O6 10. C2H6 # REDOX REACTIONS AND ELECTROCHEMICAL CELLS In aqueous solutions, the spontaneous redox reactions can be carried out directly as well as indirectly. The energy liberated during and indirect redox reactions appear in different forms. ## Direct redox reaction: A redox reaction in which reduction and oxidation takes place in the same vessel is called direct redox reaction. For example, if a zinc rod is immersed in copper sulphate solution taken in a beaker, a spontaneous reaction takes place and following observations are made: * Zinc rod starts dissolving and loses its mass gradually. * The blue colour of CuSO4 solution slowly fades. * A reddish-brown precipitate of copper settles down at the bottom of the beaker. * The reaction is exothermic. * The solution remains electrically neutral. * The reaction does not continue indefinitely but stops after sometime. The overall reaction taking place in the beaker may be represented as: Zn(s) + Cu^2+(aq) + SO4^2-(aq) → Zn^2+(aq) + SO4^2-(aq) + Cu (s) Discarding the common sulphate ions Zn(s) + Cu^2+(aq) → Zn^2+(aq) + Cu (s) **Observations Explanation:** * Zinc loses electrons and changes into Zn^2+ ions. As a result the mass of zinc rod decreases. * The electrons lost by zinc rod are gained by Cu^2+(aq) ions and they change into Cu(s) atoms which settle down at the bottom of the beaker in the form of precipitate. In direct redox reactions, the chemical energy appears as heat. ## Indirect redox reactions and electrochemical cells The redox reactions in which oxidation and reduction take place in different vessels are called **indirect redox reactions**. In such reactions, the transfer of electrons takes place indirectly through conducting wires. The decrease of energy during the indirect redox reactions appears as electrical energy. These reactions involve the conversion of the chemical energy into electrical energy. The arrangement for carrying out the indirect redox reaction is referred to as **electrochemical cell**. An electrochemical cell is a device in which chemical energy of a spontaneous redox reaction is converted into electrical energy. These are also called galvanic cells or voltaic cells. # Construction and Working of a Simple Electrochemical Cell **Key:** * **Zinc anode** * **Copper cathode** * **Salt bridge** * **ZnSO4 solution** * **CuSO4 solution** [Image Description: A diagram of a galvanic cell. * A zinc rod (anode) is placed in a beaker with a solution of ZnSO4. * A copper rod (cathode) is placed in a beaker with a solution of CuSO4. * The two beakers are connected by a salt bridge, which is an inverted U-tube filled with an electrolyte solution. * The connecting wire between the zinc and copper electrodes includes a voltmeter to measure the potential difference between the two electrodes.] **Observations:** * **Electron flow:** There is a deflection in the galvanometer, which indicates the flow of conventional current from copper to zinc indicating that copper is +ve terminal and zinc is -ve terminal. This means that electrons flow from zinc to copper. * **Mass change:** The zinc rod loses mass whereas the copper rod gains mass. * **Concentration change:** The concentration of zinc sulphate solution increases and that of copper sulphate solution decreases. * **Heat change:** No evolution of heat is observed during the reaction. * **Electrical neutrality:** The solutions in the 2 beakers remain electrically neutral throughout. * **Reaction stopping:** Reactions as well as the flow of electrons stop after sometime. **Explanation:** * Zinc atoms undergo **oxidation** and they are oxidized to Zn^2+ ions by losing two electrons. * The electrons liberated during the reaction are pushed through the connecting wire to the copper rod. * These are picked up by the Cu^2+ ions which get reduced to copper atoms. * The copper atoms so formed get deposited on the copper rod. This is why copper gains weight. **Oxidation:** Zn(s) → Zn^2+(aq) + 2e **Reduction:** Cu^2+(aq) + 2e- → Cu(s) **Overall reaction:** Zn(s) + Cu^2+(aq) → Zn^2+(aq) + Cu(s) **Electrode Terminology:** * The electrode at which **oxidation** takes place is called **anode** and that at which **reduction** takes place is called the **cathode**. * In the above electrochemical cell, oxidation occurs at the zinc rod, therefore zinc is the anode and reduction occurs at the copper rod, hence the copper is the cathode. * It may be noted that the anode electrode constitutes -ve terminal and the cathode electrode constitutes +ve terminal of the cell. By convention the **flow of current** is from the positive terminal to the negative terminal which is **opposite to the flow of electrons**. ## Function of the salt bridge: * It allows the flow of current by completing the circuit. * It maintains electrical neutrality. **Observations Explanation:** * The transfer of electrons from the zinc anode to the copper cathode leads to the development of a net positive charge around the anode due to the formation of Zn^2+ ions and net negative charge around the cathode due to the deposition of Cu^2+ ions as Cu (s) on the cathode. The positive charge accumulated around the anode will prevent electrons to flow and the negative charge due to the excess of sulphate collected around the cathode will prevent the acceptance of electrons from the anode. As the transfer of electrons stops no current flows. The salt bridge comes to the aid and restores electrical neutrality to the solutions in the two compartments. **Salt bridge** contains a concentrated solution of an inert electrolyte, the ions of which are not involved in electrochemical reactions. The anions of the electrolyte (e.g. Cl in case the salt bridge contains KCl) migrate to the anode compartment and cations, (that is K+ ions) migrate to the cathode compartment so that the solution in these compartments become neutral and the flow of electrons continues. Thus, the salt bridge helps to prevent the accumulation of charges and maintains the flow of current. # DANIEL CELL **[Image Description: A diagram of Daniell cell set up. * A copper vessel contains a solution of CuSO4. * A porous pot containing a zinc rod and a solution of ZnSO4 is placed inside the copper vessel. * The copper vessel is connected to a voltmeter.]** The electrochemical cell based on Zn-CuSO4 redox reaction is called the **Daniell Cell**. The commercial form of the Daniell cell is shown in the figure. * It consists of a copper vessel, which contains copper sulphate solution. The copper vessel also acts as the cathode. * A porous pot containing zinc sulphate solution and a zinc rod is placed in the vessel. The zinc rod acts as the anode. * The porous pot serves the purpose of the salt bridge by allowing inter-migration of ions between the two vessels or compartments. # OTHER GALVANIC OR ELECTROCHEMICAL CELLS: Theoretically any redox reaction is separable into two half-cell reactions and can be used for generating electric current in a galvanic cell. Some simple redox reactions are given below. * Cu(s) + 2Ag^+(aq) → Cu^2+(aq) + 2Ag(s) * Ni(s) + 2Ag^+(aq) → Ni^2+(aq) + 2Ag(s) * Zn(s) + 2H^+(aq) → Zn^2+(aq) + H2(g) # Representation of galvanic cells: The electrochemical cell can be represented briefly according to certain universally accepted conventions as described below: 1. **The half-cell is usually represented as M/Mn+ where M represents the symbol of the element and Mn+ represents its cation in the electrolyte.** The line (/) represents the interphase between the two phases (maybe solid/liquid or liquid/gas). The concentration of the electrolyte also may be mentioned in brackets. For example, let us consider copper electrode in contact with cupric ions with concentration equal to 1.0M. It can be represented as Cu^2+ (1.0M)/Cu if it constitutes the reduction half-cell. It can be represented as Cu/Cu^2+(1.0M) if it constitutes the oxidation half cell. 2. **Anodic half cell (oxidation half) is written on the left hand side while the cathodic half cell(reduction half) is written on the right hand side.** 3. **The two half-cells are separated by two vertical lines which indicate the salt bridge.** For example, Zn-CuSO4 cell may be represented as :Zn/Zn^2+ (1.0M) || Cu^2+ (1.0M)/Cu # EMF of cell: It has been pointed out earlier that the electrochemical cell consists of two half cells. The electrodes in these half cells have different electrode potentials. When the circuit is completed the loss of electrons occurs in the electrode having lower reduction potential whereas the gain of electrons occurs at the electrode having higher reduction potential. The difference in the electrode potentials of the two electrodes of the cell when no or very little current is drawn from it is termed as **electromotive force( EMF or cell voltage).** Mathematically it can be expressed as Ecell = E^o Reduction - E^o Oxidation Since in the representation of the cell, cathode is written on the right hand side and the anode on the left hand side, EMF of a cell is also sometimes written as, EMF = Eright - Eleft It is measured with the help of a potentiometer or a vacuum tube voltmeter. # ELECTRODE POTENTIAL When a strip of a metal M is brought in contact with a solution of its own ions Mn+, it has either of the two tendencies: Mn+ + ne↔ M In either case the equilibrium leads to separation of charges and results in the potential being developed between the metal strip and its solution. The potential developed at the interface when a metal is in equilibrium with the solution of its own ions is called **electrode potential.** **Note:** 1. Electrode potential is called **oxidation potential** if oxidation takes place and **reduction potential** if reduction takes place. 2. Reduction potential and oxidation potential of an electrode are **numerically equal but have opposite signs**. 3. According to IUPAC, electrode potential should be expressed in terms of reduction. # FACTORS AFFECTING ELECTRODE POTENTIAL: 1. Nature of electrode material 2. Concentration of metal ions in solution 3. Temperature 4. Valency # Standard electrode potential (Eº): It is defined as the potential developed at the interface when a metal is in equilibrium with one molar solution of its own ions at 298K. # Determination of standard electrode potential Absolute value of electrode potential of an electrode cannot be determined because the half cell by itself cannot cause movement of charges (flow of electrons) is due to the fact that once equilibrium is reached between the electrode and the solution of the half cell, no further displacement of charges can occur unless and until it is connected to another half cell with different electrode potential. This difficulty is overcome by finding the electrode potential of various electrode relative to a reference electrode whose electrode potential is arbitrarily fixed. The common reference electrode used for this purpose is **standard hydrogen electrode** or **normal hydrogen electrode(SHE or NHE)** whose electrode potential is arbitrarily taken as zero. # Normal Hydrogen electrode (NHE) **[Image Description: Diagram of normal hydrogen electrode setup. * A platinum wire sealed in a glass tube, with platinum foil coated with finely divided platinum, is placed in a beaker containing 1M HCl solution. * Hydrogen gas (H2) at 1 atm is continuously bubbled through the solution. ]** Normal Hydrogen Electrode consists of a platinum wire sealed into a glass tube carrying a platinum foil at one end. The platinum foil is coated with finely divided platinum. This electrode is placed in a beaker containing an aqueous solution of some acid having one molar concentration of H+ ions. Hydrogen gas at 1 atmospheric pressure is continuously bubbled through a solution at a temperature of 298K. The oxidation or reduction in the NHE takes place at the platinum foil. * **If NHE acts as anode, oxidation takes place at it:** H2(g) → 2H+(aq)+2e- In such a case, it is represented as, Pt //½ H2/H+ (1M). * **If NHE acts as cathode, reduction takes place at it:** 2H+(aq)+ 2e- → H2(g). In this case it is represented as, H⁺(1M)/12 H2, Pt. Pt here is an inert electrode. # The electrode potential of an electrode determined relative to the standard hydrogen electrode under standard conditions is called **standard electrode potential**. It is represented as Eº. The standard conditions are 1M concentration of ions in the solution, 298K temperature and 1 atmospheric pressure. The electrode potential of a standard hydrogen electrode is taken as 0V at 298 K. # Measurement of standard electrode potential of an electrode: 1. The electrode in standard conditions is connected to SHE using a salt bridge to constitute a cell. 2. Potential difference (Eºcell) is determined with the help of potentiometer. 3. From the direction of flow of current cathode and anode of the cell are determined. 4. Eºcell = Eºcathode - Eºanode. Knowing Eºcell and electrode potential of one of the electrodes(SHE), that of the other can be calculated # Example, to determine Eº Zn2+/zn, a cell is constructed as follows: Zn/Zn^2+ (1M)//H+ (1M) //½ H2 (g) (1 atm), Pt Anode Cathode Potential difference (Eºcell) is measured to be 0.76V. Eºcell = ESHE - E°Zn2+/Zn 0.76 V = 0 - E°Zn2+/Zn E°Zn2+/Zn= -0.76 V # Similarly, to determine EºCu2+/ Cu, the cell is constructed as mentioned below and the potential difference (Ecell) is measured to be 0.34V. Pt, 1/2 H2(1 atm)/ H+ (1м)// Cu 2+ (1M)/ Cu (s) Anode cathode Ecell = E° Cu2+/Cu - ESHE 0.34 V = E° Cu2+/Cu - 0 E°Cu2+/Cu= 0.34 V # ELECTROCHEMICAL SERIES The arrangement of various elements in the order of increasing value of standard reduction potentials is called the **electrochemical series** or **activity series**. ## Electrochemical Series Table Standard Electrode | Electrode reaction | Standard electrode potential (V) -------------------|---------------------|----------------------------- Li^+/ Li | Li^++ e^ - → Li | -3.04 K^+/K | K^++ e^ - → K | -2.92 Ba^2+/ Ba | Ba^2+ + 2e^- → Ba | -2.90 Ca^2+/ Ca | Ca^2+ + 2e^- → Ca | -2.87 Na^+/ Na | Na^++ e^ - → Na | -2.71 Mg^2+/ Mg | Mg^2+ +2e^- → Mg | -2.37 Zn^2+/ Zn | Zn^2+ +2e^- → Zn | -0.76 Fe^2+/ Fe | Fe^2+ + 2e^- → Fe | -0.44 H^+/ 1/2 H2, Pt | H^++ e^- → 1/2 H2 | 0.00 Cu^2+/ Cu | Cu^2+ +2e^- → Cu | +0.34 Ag^+/ Ag | Ag^++ e^ - → Ag | +0.80 Au^3+/ Au | Au^3+ + 3e- → Au | +1.50 Pt, 1/2 F2/ F^ - | 2F2+e^- → F- | +2.85 # Applications of the electrochemical series Some of the important applications of the electrochemical series have been discussed as follows: 1. **Calculation of the standard EMF of a cell:** From the electrochemical series, the standard reduction potentials of the electrodes are found out. The electrode with higher standard reduction potential is taken as the cathode & the other is taken as the anode. From this, standard EMF of the cell is calculated as: Ecell = Ecathode - Eanode 2. **Comparison of Reactivity of metals:** The metals occupying higher positions in the electromagnetic series have lower reduction potentials. This indicates that ions of such metals will not be reduced to metals easily. On the contrary, such metals would be easily oxidized to their ions by losing electrons. Therefore from the position of a metal in the electrochemical series, it is possible to predict the relative reactivity of metals. A metal having smaller reduction potential (i.e. greater negative reduction potential) can displace metals having greater reduction potential (i.e. smaller negative reduction potential) from the solutions of their salts. In other words, a metal occupying higher in the series can displace the metals lying below it from the solutions of their salts. For example, zinc lies above copper in the series& hence, it can displace copper from copper sulphate solution & copper can not displace zinc from zinc sulphate solution because it lies below zinc in the series & hence it is less reactive. Thus, we can conclude that the metals occupying higher positioning the electrochemical series are more reactive in displacing the other metals from the solutions of their salts. For example, zinc lies above copper in the series & hence it can displace copper from copper sulphate solution & copper cannot displace zinc from zinc sulphate solution because it lies below zinc in the series & hence its less reactive. 3. **Predicting the feasibility of a redox reaction:** With the help of the electrochemical series we can predict whether a given redox reaction will take place or not. From the given equation the substances undergoing oxidation & reduction are identified. The substance undergoing oxidation will act as anode & the substance undergoing reduction will act as a cathode. The EMF of this hypothetical cell is calculated as under: E cell = Eº cathode - Eº anode If EMF comes out to be positive the given redox reaction will take place & if the EMF comes out to be negative redox reaction will not take place. 4. **To predict the reaction of a metal with dilute acids to liberate hydrogen gas:** Some metals like iron, zinc react with dilute acids like HCl, H2SO4 to liberate hydrogen gas while some other metals like copper, silver do not liberate hydrogen gas with dilute acids like HCl, H2SO4. A prediction about the capacity of a given metal to produce hydrogen gas by its reaction with dilute acids can be easily made from the knowledge of the electrochemical series. Metals which lie above hydrogen in the electrochemical series can reduce H+ ions to hydrogen and hence liberate hydrogen gas on reaction with dilute acids. In other words metals having -ve reduction potentials can displace hydrogen from acids. For example, zinc( E° Zn /Zn = -0.76 volt) lies above hydrogen and hence it can displace hydrogen from dilute acids whereas copper (Ecu /Cu = +0.34 volt) which is lying below hydrogen in the series cannot displace hydrogen from acids # ELECTROLYSIS The process of chemical decomposition of the electrolyte by the passage of electricity through its molten or dissolved state is called electrolysis. ## Electrolytic cell: The device or arrangement in which the process of electrolysis is carried out. It consists of the following parts: * **Electrolytic Tank:** Which is made up of some non-conducting material like glass, wood or bakelite. * **Electrolyte:** In its dissolved state or molten state is contained in the electrolytic tank. * **Source of electricity:** An electrochemical cell or battery. * **Two metallic strips or rods:** Suspended in the electrolyte and connected to the battery through conducting wires. The rods are called **electrodes**. The electrode which is connected to the negative terminal is called the **cathode** while the other one which is connected to the positive terminal of the cell is called **anode**. **[Image Description: A diagram of an electrolytic cell setup. * An electrolytic tank containing a solution of sodium chloride is connected to an external battery. * Two electrodes (one cathode and one anode) are immersed in the solution, connected to the positive and negative terminals of the battery respectively.]** # Electrolysis of an aqueous solution of sodium chloride In aqueous solution, sodium chloride dissociates as: NaCl(aq)→Na+(aq) + Cl-(aq) Water also ionizes although to a very small extent as: H2O (l)→H+(aq) + OH(aq) The aqueous solution of sodium chloride thus contains Na+ and H+ (cations) and Cl- and OH- (anions). When electricity is passed through the solution, the following reactions take place at the respective electrodes ## AT CATHODE: H+ ions are preferentially discharged at the cathode by accepting electrons and thereby producing hydrogen gas. This pushes the dissociation equilibrium of water in the forward direction producing more and more number of OH- ions. The net reaction at the cathode involves the reduction of H2O molecules which can be represented as: 2H2O +2e- → 2OH-(aq) + H2 ## AT ANODE: Cl- ions are preferentially liberated at the anode. Cl- → Cl + e- (primary change) Cl + Cl → Cl2 (secondary change) The overall reaction representing electrolysis of aqueous solution of sodium chloride: Electrolysis: 2Na+ +2Cl- +2H2O → 2Na+ + 2OH+H2(g) +Cl2(g) # Differences between electrochemical cells and electrolytic cells: | Feature | Galvanic cell | Electrolytic cell | |----------------------|----------------|-------------------| | Electrical energy | Produced | Consumed | | Reaction direction | Spontaneous | Non spontaneous | | Electrode placement | Two half cells connected through salt bridge or porous partition | Both the electrodes are placed in the same container | | Anode | Negative | Positive | | Cathode | Positive | Negative | # CORROSION OF METALS: **[Image Description: Diagram of an iron nail corroding in a water droplet, showing the formation of rust (Fe2O3, H2O). * The water droplet acts as an electrolyte, and the nail is divided into anodic and cathodic areas. * At the anodic area, iron (Fe) is oxidized to Fe^2+ ions which are released into the water. * At the cathodic area, oxygen (O2) reacts with water, and electrons are consumed, forming hydroxide ions (OH-) and oxygen (O^2-).]** Corrosion of metals is the slow destruction of metals as a result of the chemical attack by air and the moisture present in the atmosphere. During the process of corrosion the useful metals are oxidized by the oxygen present in the atmosphere in the presence of moisture to form a mixture of metal oxide and metal hydroxide. This is an unending process which gradually eats up the whole metal e.g. iron metal corrodes in the presence of air and moisture to form a brown coating of Fe2O3 and Fe (OH)3 on its surface: 4 Fe + 3O2 + 3H2O → Fe2O3 + 2Fe(OH)3 ## Conditions necessary for corrosion: Two conditions are necessary for corrosion of metals to take place: 1. Presence of oxygen in air 2. Presence of moisture in air ## Factors affecting the rate of corrosion: There are several factors that can increase the rate of corrosion. These are discussed below: * **Presence of another metal or impurity:** The process of corrosion is speeded up when two metals are in contact with each other. This is due to the fact that the two metals form an electrochemical cell in the presence of moisture and the electrons start flowing from the most reactive metal to the least reactive metal, By losing electrons the more active metal forms metal ions and hence metal compounds. For example if iron and copper are placed in contact then they will form an electro chemical cell. Iron is more reactive than copper so it will undergo oxidation easily and the electrons will flow towards copper speeding up the oxidation of iron. # Pollutants in air (acidic oxides like SO2 CO2 NO2): Air polluted from smoke from factory chimneys contains acidic oxides which dissolve in moisture to give hydrogen ions which increases the rate of corrosion. # Prevention of corrosion: 1. **Barrier protection:** A barrier film is introduced between iron and atmosphere by (a) painting the surface (b) coating the surface with a thin film of grease or oil and (c) electroplating iron with metals like Cu, Cr or Ni etc., 2. **Sacrificial protection:** Here the surface of iron is covered with a layer of more reactive metal like zinc. Since the reduction potential of zinc is less than that of iron, zinc loses electrons in preference to iron and is consumed. As long as zinc is present, even the nearly uncovered surfaces of iron do not get rusted. The process of covering iron with zinc is known as galvanization. 3. **Using antirust solution:** When iron articles are dipped in boiling and strongly alkaline sodium phosphate( or chromate) solution, a protective insoluble film of iron phosphate is formed on them. The alkaline nature of solution decreases the availability of H+ ions which facilitates the oxidation of iron to Fe^2+. 4. **Electrical protection:** The underground iron pipes etc can be protected from rust by connecting them with a more reactive metal like Mg, Zn or Al. More active metal acts as anode and loses electrons in preference to iron. Thus, iron is prevented from rusting. This is called cathodic protection of iron.