Physical Geology PDF
Document Details
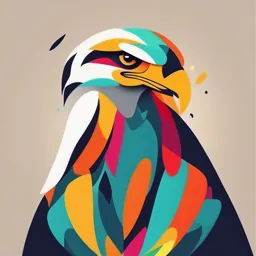
Uploaded by IssueFreeGyrolite4268
Rafa M.Zaki
Tags
Summary
This document is a chapter-by-chapter introduction to Physical Geology. It covers the composition of Earth materials, Earth structures, and Earth processes. It also touches upon different branches of geology, and explores the solar system and the Earth's internal structure, including the history of drilling.
Full Transcript
Physical Geology Prof. Dr. Rafa M.Zaki CHAPTER ONE INTRODUCTION What is the Geology? The word means "study of the Earth". Geology deals with the composition of Earth materials, Earth structures, an...
Physical Geology Prof. Dr. Rafa M.Zaki CHAPTER ONE INTRODUCTION What is the Geology? The word means "study of the Earth". Geology deals with the composition of Earth materials, Earth structures, and Earth processes. It is also concerned with the organisms of the planet and how the planet has changed over time. Geologists search for fuels and minerals, study natural hazards, and work to protect Earth's environment. What do geologists do? Geology is a multi-faceted field with many different areas of specialization. Listed below are some of the more common ones. 1- Earth Science: A mixture of geology, oceanography and climatology) in junior and senior high schools. A teaching certificate from a professional education program is also normally required. 2-Economic Geology: explore for and help produce metallic (iron, copper, gold, etc.) and non-metallic (coal, granite dimension stone, limestone aggregate, sand and gravel, etc.) rock and mineral resources of economic value. 3-Engineering Geology: investigate the engineering properties of rock, sediment and soil below man-made structures such as roads, bridges, high-rise buildings, dams, airports, etc. 1 Physical Geology Prof. Dr. Rafa M.Zaki 4-Environmental Geology: study the environmental effects of pollution on ground and surface waters and surficial materials (rock, sediment and soil), and also recommend solutions to environmental problems. They are also interested in understanding, predicting and mitigating the effects of natural hazards, such as flooding, erosion, landslides, volcanic eruptions, earthquakes, etc. 5-Geochemistry: investigate the chemical composition and properties of earth materials, especially polluted ground and surface waters, fossil fuels (such as petroleum and coal) and other resources of economic value. 6-Geomorphology: study the origin and evolution of landscapes on the continental surfaces. 7-Geophysics: use the principles of physics to investigate the structure of the Earth's deep interior, explore for economic resources in the subsurface, and monitor pollution in ground water. 8-Glacial or Quaternary Geology: study the history of geologically recent (Quaternary period) glaciers as well as the sediment deposits and landforms they produced. 9-Hydrogeology: are concerned with water in the Earth's subsurface, including its sources, quality, abundance and movement. 10-Hydrology: are concerned with water on the Earth's surface, including its precipitation, evaporation and runoff, and its abundance and quality in streams and lakes. 2 Physical Geology Prof. Dr. Rafa M.Zaki 11-Marine Geology: study the physical, chemical and biological characteristics of the sediments deposited on the ocean floors and the rocks that underlie them. 12-Mineralogy: investigate the origins, properties and uses of the minerals occurring within the Earth's rocks. 13-Paleontology: study the remains of ancient animals and plants (fossils) in order to understand their behaviors, environmental circumstances, and evolutionary history. 14-Petroleum Geology: explore for and help produce petroleum and natural gas from sedimentary rocks. 15-Petrology: study the origins and characteristics of igneous, metamorphic and sedimentary rocks. 16-Sedimentology: investigate the origins and characteristics of sediment deposits and the sedimentary rocks that form from them. 17-Stratigraphy: investigate the time and space relationships among sedimentary and other rocks on local to global scales, and are also interested in the geochronology (absolute dating by radiometric methods) and fossil content of rock layers. 18-Structural Geology: study the folding, fracturing, faulting and other forms of deformation experienced by rocks below the Earth's surface, and are also interested in how these processes relate to global Plate Tectonics. 3 Physical Geology Prof. Dr. Rafa M.Zaki 19-Physical Geology: discuss the processes that affect the earth crust as weathering, surface water and groundwater ….etc as well as volcanoes and earthquakes. 20 -Historical Geology: Examines origin of the Earth, origin of life, and changes in Earth andlife through time. It deals with the layered rock record and fossils. 4 Physical Geology Prof. Dr. Rafa M.Zaki CHAPTER TWO Solar System and the Earth The Solar System that we live in consists of a medium-size star (the Sun) with eight planets orbiting it. The planets are of two different types; 1. Terrestrial Planets: These include the inner planets, which arc, small, high densities, rocky and poor in ices and H/He with no rings, rotate slowly and few or no moons (Mercury, Venus, Earth, and Mars). 2. Jovian Planets: These include the outer planets which are large, low density, and gaseous (H, He, CH4), rotate rapidly. All have rings and have many moons (Jupiter - largest planet. Saturn. Uranus. Neptune). Other planet that does not fit into the two previous categories e.g., Pluto, which is the outermost planet - small, icy, low density and has one moon. Fig. The Terrestrial and Jovian Planets. 5 Physical Geology Prof. Dr. Rafa M.Zaki ORIGIN OF THE EARTH AND THE SOLAR SYSTEM The Nebular Theory In this theory, the whole solar system starts as a large cloudcomposed mostly of hydrogen and helium with only small percentage of the heavier elements. About 5 billion years ago, this cloud began to contract under its own gravitational influence; this contraction caused rotation of the cloud, which became faster and faster as it contracted. The rotation caused the nebular cloud to flatten into a desk. Smaller accumulation formed nuclei from which the planets formed. The sun was formed at the center of the desk. Fig. 1: Sketch of Nebular theory While this theory incorporates more basic physics, there are several unsolved problems. For example, a majority of the angular momentum in 6 Physical Geology Prof. Dr. Rafa M.Zaki the Solar System is held by the outer planets. For comparison, 99% of the Solar System's mass is in the Sun, but 99% of its angular momentum is in the planets. Another flaw is the mechanism from which the disk turns into individual planets. How old of the Earth? 4.5 to 4.6 billion years (4,500,000,000 to 4,600,000,000 years) as determined through radiometric dating (Uranium, Thorium), using an instrument called a mass spectrometer. The ideas of the age of the Earth are discussed by Usher (1654), Hutton (1785), and Romans (1820). Where do we find the oldest rocks on Earth? 1. Canadian Shield. (NW Territories near Great Slave Lake, 3.96 by). Gneiss. Narrows the gap between origin of Earth and first rocks to 640 million years. Before this, oldest rocks known were from Isukasia region of Greenland (3.8 by). 2. Glaciers: 2 miles thick scraped off young recycled rocks. Land rose 250 ft since ice was removed indicating more erosion. Spheres of the Earth There are Four spheres of the earth: atmosphere, hydrosphere, biosphere and lithosphere. 1. Lithosphere: The lithosphere is the solid, rocky crust covering entire planet. This crust is composed of igneous, sedimentary and metamorphic rocks. 7 Physical Geology Prof. Dr. Rafa M.Zaki 2. Hydrosphere: It includes all water bodies on the earth such as, the oceans, which constitute about 71% of the earth's surface, the fresh water streams, lakes as well as the underground water. Fresh water is very important for life and also responsible for sculpting and creating many landforms on the earth. 3. Biosphere: It includes all life on the earth either plant life or animal life, in the sea or onland. 4. Atmosphere: It includes the air envelope surrounding the earth. It is important forbreathing and for protection against sun's heat and ultraviolet radiation. It is divided intoseveral layers arranged upwards from the earth's surface: Troposphere (where weather takes place), Stratosphere (where the ozone layer that protects the surface of the Earth from UV rays is found), Mesosphere, Thermosphere and Exosphere (which grades off into outer space). Fig. : Spheres of the earth Earth Structure How do we know what the Earth's Interior is like? 8 Physical Geology Prof. Dr. Rafa M.Zaki Early pioneer studies aiming to know the internal structure of the earth including the following: 1. Drilling: The history of drilling around the world can be summarized as follows: a. Wells drilled into Earth are mostly in the upper 7 km of the crust. Deepest well- Soviet (Russian) well in northern Kola Peninsula 20 year effort to drill a 12 km hole and stopped in 1989. History: 5 years to drill 7 km; 9 years to drill the next 5 km: got stuck at 12 km. Target depth is 15 km. Costs are more than $100 million, bottom hole temperature is 190°C Current status? b. Deepest US well is next to San Andreas Fault (Cajon Pass) Had reached 3.5 km in 1988. Cost was $5 million ($1400 per meter) Cost overruns and budget cuts suspended drilling in 1988. Other deep holes are planned. c. Costs of a German 10 km hole are estimated at $110 million (or $11,000 per meter) d. Germans drilled 3.5 km pilot hole and found bottom temperature was II8°C (instead of the expected 80°C). 2. Volcanic activity: Materials are brought up from below. Xenoliths = foreign rock (pieces of the mantle in lava) example: coarse-grained olivine (peridotite) xenoliths in basaltic lava. Only useful to depth of about 200 km. 3. Samples of the solar system (meteorites). 4. Study of seismic waves generated by earthquakes and nuclear explosions. 9 Physical Geology Prof. Dr. Rafa M.Zaki The interior of the Earth 4.5 billion years ago, the Earth was formed in a massive conglomeration and bombardment of meteorites and comets. The immense amount of heat energy released by the high-velocity bombardment melted the entire planet, and it is still cooling off today. The Earth is divided into three chemical layers: the core, the mantle and the crust (Fig.). The core is composed of iron and nickel and remains very hot, even after 4.5 billion years of cooling. The core is divided into two layers: a solid inner core and a liquid outer core. The inner Core is under such extreme pressure that it remains solid. The middle layer of the Earth is the mantle, is made of minerals rich in the elements iron, magnesium, silicon, and oxygen. Al over I000°C, the mantle is solid but can deform slowly in a plastic manner. The crust is much thinner than any of the other layers, and is composed of the least dense calcium (Ca) and sodium (Na- aluminum-silicate minerals. Being relatively cold, the crust is rocky and brittle, so it can fracture in earthquakes. Fig.: The layers of the earth. 11 Physical Geology Prof. Dr. Rafa M.Zaki There are two types of crust: Oceanic crust is made of relatively dense rock called basalt, the most common rock on Earth. Continental crust is made of lower density rocks, such as andesite and granite. The outermost layers of the Earth can be divided by their physical properties into lithosphere and asthenosphere (Fig. ). Fig. Lithosphere and asthenosphere The lithosphere is the rigid outermost layer made of crust and uppermost mantle. The lithosphere is the "plate" of the plate tectonic theory. The asthenosphere is part of the mantle that flows. It might seem strange that a solid material can flow. A good example of a solid that Mohs, or of plastic behavior, is the movement of toothpaste in a tube. The How of the asthenosphere is part of mantle convection, which plays an important role in moving lithospheric plates. Exploring the Earth's Core How was the Earth's core discovered? Recordings of seismic waves from earthquakes gave the first clue. Seismic waves will bend and reflect 11 Physical Geology Prof. Dr. Rafa M.Zaki at the interfaces between different materials, just like the prism refracts and scatters light waves at its faces (Fig. ). P and S wave travel times depend on properties of rock materials that they pass through. Wave velocity depends on density and elasticity of rock. Seismic waves travel faster in denser rock. Speed of seismic-waves increases with depth as pressure and density increase downward (Table I). Table (1): Densities and the P-wave velocities through the different layers of the Earth. Layer Density (g/cm3) P-wavevelocity (km/sec) Continental crust 2.6 - 2.8 6 Oceanic crust 3.5 7 Mohrovic discontinuity (Moho) Mantle 4.5 - 10 8 - 12 Gutenberg discontinuity - Core (average) 12 - Outer core (liquid) 8 - 10 Inner core (solid) 13. 5 11-12 12 Physical Geology Prof. Dr. Rafa M.Zaki CHAPTER THREE PLATE TECTONICS THEORY Prior to the emergence of "The New Global Tectonics", other explanations accounted for some of Earth's geologic features, such as mountain ranges. These observations were common explained by a "geosynclinal theory". It was thought that elongate belts of deep subsidence a thick sedimentation existed, which were called "geosynclines". The thick geosynclinal strata were folded and metamorphosed and then uplifted and eroded to form the mountain bells that we see today. The Plate tectonic theory is a relatively new one that has revolutionized the way geologists think about the Earth. According to the theory, the surface of the Earth is broken into large plates. The size and position of these plates change over time: The edges of these plates where they move against each other are sites of intense geologic activity, such as earthquakes, volcanoes, and mountain building. Plate tectonics is a combination of two earlier ideas continental drift and sea-floor spreading. Continental drift is the movement of continents over the Earth's surface and in their change in position relative to each other. Sea- floor spreading is thecreation of new oceanic crust at mid-ocean ridges and movement of the crust away from the mid-ocean ridges. I. CONTINENTAL DRIFT (Wegener's Theory) Wegener (1912) originally proposed continental drift. He used the fit of the continents, tin distribution of fossils, and a similar sequence of 13 Physical Geology Prof. Dr. Rafa M.Zaki rocks at numerous locations, ancient climates, and the apparent wandering of the Earth's Polar Regions to support his idea. Wegener used his observations to hypothesize that all of the present-day continents were once part of a single supercontinent called Pangaea (Fig.). Continental Drift – Fossils Fossils of the same species were found on several different continents (Fig.). Wegener proposed that the species dispersed when the continents were connected and later, carried to their present positions as the continents drifted. For example, Glossopteris, a fern, was found on the continents of South America, Africa, India, and Australia. If the continents are reassembled into Pangaea, the distribution of Glossopteris can be accounted for over a much smaller contiguous geographic area. The distribution or other species can also be accounted for by initially spreading across Pangaea, followed by the breakup of the supercontinent, and movement of the continents to their present positions (Fig. ). 14 Physical Geology Prof. Dr. Rafa M.Zaki Continental Drift - Rock Sequences Rock sequences in South America, Africa, India, Antarctica, and Australia show remarkable similarities. Wegener showed that the same three lavers occur at each of these localities. The bottom layer is called tillite and is thought to be a glacial deposit. The middle layer is composed of sandstone, shale, and coal beds. Glossopteris fossils arc in the bottom and middle layers. The top (youngest) layer is lava flows. The same three layers are in the same order in areas now separated by great distances. Wegener proposed that the rock layers were made when all the continents were part of Pangaea. Thus, they formed in a smaller contiguous area that was Inter broken and drifted apart (Fig. ). Continental Drift - Glaciations Glaciations in South America, Africa, India, and Australia are best explained if these continents were once connected. Glaciers covered all or part of each of these continents during the same time period in the geologic past (Fig. ). If the continents were in their present position, a major glaciations event that covered nearly all of the continents and extended north of the equator would be required. Geologists have found no evidence of glacial action in the northern hemisphere during this time period. In fact, during this time period, the climate in North America was warm. Wegener proposed that the continents were adjacent to each other during the glacial event. Therefore, glaciers spread over a much smaller area in the southern hemisphere and probably did not influence the climate of the northern hemisphere (Fig. ). Wegener used the distribution of specific rock types to determine the distribution of climate zones in the geologic past. For example, glacial till 15 Physical Geology Prof. Dr. Rafa M.Zaki and striations (scratches on the rock), sand dunes, and coral reefs, indicate polar, desert, and tropical climates, respectively. The present climate zones are shown in (Fig.). Note how the distribution of reefs, deserts, and glacial ice constrain the position of the rotational pole of the earth. Fig. : Grooves carved by glaciers (shown by arrows) provided evidence for continental drift. This diagram assumes the continents were in their present-day locations. I): The distribution of glacial features can be best explained if the continents were part of Pangaea. Using the distribution of rock types, Wegener reconstructed the distribution of climates zones at specific times in the geologic past. He found that, unlike the present distribution, in which zones parallel the 16 Physical Geology Prof. Dr. Rafa M.Zaki equator, the past zones occupied very different positions. This implies that the rotational pole was invery different locations relative to today. Wegener proposed an alternative interpretation. He believed that the climate zones remained stationary and the continents drifted to different locations. The drift of the continents caused the apparent movement of the climate zones (Fig.). Wegener used the distribution of climate zones to determine the location of the poles at different times in the geologic past. He found that the rotational pole appears to gradually change location, arriving at its present position only in the very recent geologic past. The apparent movement in the pole position over time is called polar wandering. Wegener suggested that the poles remained stationary and that the continents changed their positions relative to the poles (Fig. ). Problems with Wegener's Model of Continental Drift Not all geologists accept Wegener's model. Some thought that dispersion by winds or ocean currents could explain the distribution of fossil species. Other geologists thought the poles might wander and continents remain stationary. The greatest shortcoming was the lack of an adequate mechanism for moving the continents. Wegener proposed that the Earth's spin caused the continents to move, plowing through the oceanic plate and producing mountains on their leading edges. Geologists at that lime understood enough about the strength of rocks to know that this was highly unlikely. Wegener's work was unaccepted in the northern hemisphere. In the southern hemisphere, where geologists were familiar with the rocks that 17 Physical Geology Prof. Dr. Rafa M.Zaki Wegener used to support his hypothesis, continental drift was accepted (Fig. ). Holmes (1928) proposed a mechanism to move continents (Fig.). He believed heal trapped in the Earth caused convection currents, areas where fluids beneath the Earth's crust rise, flow laterally, and then fall. The currents would rise beneath continents, spread laterally, and then plunge beneath the oceans. Fig. ( ): Holme's model of convection currents. Revival of the Continental Drift Hypothesis Advances knowledge of the sea floor and in the magnetic properties of rocks provided new evidence to support continental drift. Geologists have known that a ridge exists in the middle of the Atlantic Ocean. The Mid-Atlantic Ridge is 6,500 feet (2,000 m) above the adjacent sea floor, which is at a depth of about 20,000 feet (6,000 m) below sea level. The global system of mid-ocean ridges is also an active seismic belt, or zone of earthquakes, correspond to a trough, of rift, system similar to the trough known at the crest of the Mid-Atlantic Ridge. The rifts are about 20 miles (30 km) wide and 6,500 feet (2,000 m) deep. In all, the oceanic ridges and their rifts extend for more than 37,500 miles (60,000 km) in all the world's oceans. 18 Physical Geology Prof. Dr. Rafa M.Zaki II. SEA-FLOOR SPREADING Hess (1962) presented an explanation for the global rift system. He proposed that newocean floor is formed at the rift of mid-ocean ridges. The ocean floor and the rock beneath itareproduced by magma that rises from deeper levels. Hess suggested that the ocean floor movedlaterally away from the ridge and plunged into an oceanic trench along the continental margin. Atrench is a steep-walled valley on the sea floor adjacent to a continental margin (Fig. ). Forexample, ocean crust formed at the East Pacific Rise, an oceanic ridge in the east Pacific, plungesinto the trench adjacent to the Andes Mountains on the west side of the.South Americancontinent. In Hess' model, convection currents push the ocean floor from the mid-ocean ridge tothe trench and also help move the continents, much like a conveyor belt. As Hess formulated his hypothesis, Dietz independently proposed a similar model andcalled it sea floor spreading. Dietz's model assumed the sliding surface was at the base of thelithosphere, not at the base of the crust.Hess and Dietz succeeded where Wegener had failed. Continents are no longer thought to plow through oceanic crust but are considered to be part of plates that move on the soft, plastic asthenosphere. A driving force, convection currents, moved the plates. Technological advances and detailed studies of the ocean floor, both unavailable during Wegener's time, allowed Hess and Dietz to generate the new hypotheses. Sea-Floor Spreading Hypothesis Before being widely accepted, a new hypothesis must be tested. One test for the sea-floor-spreading hypothesis involved magnetic patterns on the sea floor (Fig. ). 19 Physical Geology Prof. Dr. Rafa M.Zaki In the late 1950's, scientists mapped the present-day magnetic field generated by rocks on the floor of the Pacific Ocean. The volcanic rocks, which make up the sea floor, have magnetization because, as they cool, magnetic minerals within the rock align to the Earth's magnetic field. The intensity of the magnetic field they measured was very different from the intensity they had calculated (Fig.). Thus, the scientists detected magnetic anomalies or differences in the magnetic field from place to place. They found positive and negative magnetic anomalies. Positive magnetic anomalies are places where the magnetic field is stronger than expected. Positive magnetic anomalies are induced when the rock cools and solidifies with the Earth's north magnetic pole in the northern geographic hemisphere. The Earth's magnetic field is enhanced by the magnetic field of the rock. Negative magnetic anomalies arc magnetic anomalies that are weaker than expected (Fig. ). Negative magnetic anomalies are induced when the rock cools and solidifies with the Earth's north magnetic pole in the southern geographic hemisphere. The resultant magnetic field is less than expected because the Earth's magnetic field is reduced by the magnetic field of the rock. 21 Physical Geology Prof. Dr. Rafa M.Zaki When mapped, the anomalies produce a zebra-striped pattern of parallel positive and negative bands. The pattern was centered along, and symmetrical to, the mid-ocean ridge. Vine and Matthews (1963) proposed that lava erupted at different times along the rift at the crest of the mid-ocean ridges preserved different magnetic anomalies. For example, lava erupted in the geologic past, when the north magnetic pole was in the northern hemisphere, preserved a positive magnetic anomaly. In contrast, lava erupted in the geologic past, when the north magnetic pole was in the southern hemisphere, preserved a negative magnetic anomaly. Lava erupting at the present time would preserve a positive magnetic anomaly because the Earth's north magnetic pole is in the northern hemisphere. Vine and Matthews proposed that lava erupted on the sea floor on both sides of the rift, solidified, and moved away before more lava was erupted. If the Earth's magnetic field had reversed (changed from one geographic pole to the other) between the two eruptions, the lava flows would preserve a set of parallel bands with different magnetic properties. The 21 Physical Geology Prof. Dr. Rafa M.Zaki ability of Vine and Matthews' hypothesis to explain the observed pattern ' of ocean floor magnetic anomalies provided strong support for sea floor spreading. Subduction If new oceanic lithosphere is created at mid-ocean ridges, where does it go? Geologists had the answer to this question before Vine and Matthews presented their hypothesis. Wadati (1935) showed that earthquakes occurred at greater depths towards the interior of the Asian continent. Earthquakes beneath the Pacific Ocean occurred at shallow depths. Earthquakes beneath Siberia and China occurred at greater depths (Fig. ). After World War II, Benioff observed the same distribution of earthquakes but could not offer a plausible explanation. The movement of oceanic lithosphere away from mid-ocean ridges provides an explanation. Convection cells in the mantle help carry the lithosphere away from the ridge. The lithosphere arrives at the edge of a continent, where it is subducted or sinks into the asthenosphere. Thus, oceanic lithosphere is created at mid-ocean ridges and consumed a 22 Physical Geology Prof. Dr. Rafa M.Zaki subduction zones, areas where the lithosphere sinks into the asthenosphere (Fig. ). Earthquake is generated in the rigid plate as it is subducted into the mantle. The dip of the plate under the continent accounts for the distribution of the earthquakes. Magma generated along the top of the sinking slab rises to the surface to form stratovolcanoes. III. THE BIRTH OF PLATE TECTONICS The synthesis began when Wilson (1965) introduced the term plate for the broken pieces of the Earth's lithosphere. In 1967, Morgan proposed that the Earth's surface consists of twelve rigid plates that move relative to each other (Fig. ). Le Pichon published a synthesis showing the location and type of plate boundaries and their direction of movement. Since the mid-1960s, the plate tectonic model has been rigorously tested. Because the model has been successfully tested by numerous methods, it is now called the plate tectonic theory and is accepted by almost all geologists. Location of Plate Boundaries Earthquakes and volcanoes, evidence of unrest in the Earth, help locate the edges of plates. Earthquakes are distributed in narrow, linear belts that circle the Earth (Fig. ). Some of these belts have only shallow (0- 20 miles; 0-35 km) earthquakes, like the mid-Atlantic and cast Pacific ridges. In contrast, earthquakes in other belts, like western South America and south-central Asia, are at shallow, intermediate (20-45 miles; 30-70 km), and deep (45-450 miles: 70-700 km) levels. Volcanoes are also distributed in long belts that circle the Earth (Fig.18). A dramatic example is the line of volcanoes that circles most of the Pacific Ocean. This belt is 23 Physical Geology Prof. Dr. Rafa M.Zaki known as the "Ring of Fire" because it is the site of frequent volcanic eruptions. The distribution of earthquakes and volcanoes coincides at most locations. The Ring of Fire is an excellent example. Geologists believe that areas of intense geologic activity, indicated by earthquakes, volcanoes, and/or mountain building, mark the boundaries between lithospheric plates. The distribution of earthquakes, volcanoes, and mountain ranges define 7 large plates and 20 smaller plates. The Nazca and Juan de Fuca Plates consist of only oceanic lilhosphere. The Pacific Plate is mostly oceanic lithosphere only a small slice of continental lithospherc in southern California and Baja Mexico. Most of the other plates consist of both oceanic and continental lithosphere. 24 Physical Geology Prof. Dr. Rafa M.Zaki Types of plate motion The ways that plates interact depend on their relative motion and whether oceanicorcontinental crust is at the edge of the lithospheric plate (Fig. ). Plates move away from, toward,or slide past each other. Geologists call these divergent, convergent, and transform plateboundaries. Types of Plate Boundaries There are three types of plate boundaries: 1. Divergent Plate boundaries, where plates move away from each other. 2. Convergent Plate Boundaries, where plates move toward each other. 3. Transform Plate Boundaries, where plates slide past one another. 1. At a divergent plate boundary (mid-ocean ridges and continental rifts) lithospheric plates move away from each other (Fig.). The mid- Atlantic Ridge, a topographically high area near the middle of the Atlantic Ocean, is an example of a divergent plate boundary. 2. At a convergent plate boundary (subduction zones and sites of continental collision forming mountain belts), lithospheric plates 25 Physical Geology Prof. Dr. Rafa M.Zaki move toward each other (Fig.). The west margin of the South American continent, where the oceanic Nazca Plate is pushed toward and beneath the continental portion of the South American Plate, is an example of a convergent plate boundary. 3. At a transform plate boundary, transform faults (easily seen where they cut at right angles to the mid-ocean ridges), plates slide past each other (Fig.). The San Andreas fault in California is an example of a transform plate boundary, where the Pacific Plate slides past the North American Plate. 26 Physical Geology Prof. Dr. Rafa M.Zaki Some basic terminology Island Arcs: chains of volcanoes adjacent to subduction zones. Hot spots: Thermal plumes (heat rising in mantle). Plates move over hot spots creating a chain of volcanoes. Hawaiian Islands, Emperor Sea Mounts 27 Physical Geology Prof. Dr. Rafa M.Zaki CHAPTER FOUR PRIMARY AND SECONDARY STRUCTURES PRIMARY SEDIMENTARY STRUCTURES Sedimentary structures are features created when sediments are accumulating as a result of the action of natural processes such as waves, currents, drying events, etc. Because these structures reflect the character of the transporting medium, they can be used in detecting ancient environments. Many of the structures are on the scale of tenths to tens of meters and so are studied, recorded and measured in the field. Sedimentary structures, particularly those formed during sedimentation, have a variety of uses: 1. For interpreting the depositional environment in terms of processes, water depth, wind strength etc. 2. For determining the way up of a rock sequence in an area of complex folding. 3. For deducing the palaeocurrent pattern and palaeogeography. Sedimentary structures include: Bedding means the occurrence of sedimentary rocks in the form of layers called strata or beds (Fig. ). Beds differ from each other in grain size, mineral composition, texture, etc. The planes separating these beds are called bedding planes. These are flat surfaces along which rocks tend to separate or break. Each bedding plane marks the end of one episode of sedimentation and the beginning of another. 28 Physical Geology Prof. Dr. Rafa M.Zaki Graded Bedding: This is also another kind of bedding where the particles within a single layer gradually change from coarse at the bottom to fine at the top (Fig. ). It is characteristic of rapid deposition from water containing sediments of varying sizes. As the energy of water decreases, coarse grains deposit first, and in time smaller particles settle to produce graded bedding. Cross bedding: Normally, most beds are originally deposited horizontal: In some case, the layers within a bed are inclined to the horizontal. This is called cross bedding (Fig.) and is characteristic of sand dunes; rivers deltas are stream channel deposits. Ripple Marks: Ripple marks are undulations of sand that develop on the surface of a sediment layer by the water or wind (Fig. ). Ripple marks formed by water or wind moving in one direction are called current ripple marks. They are asymmetric and have steep slops in the down-current side and gentle slope in the up-current side. Current Ripples include those formed on the surface of sand dunes or those formed by a stream flowing in a sandy channel. Ripple marks formed by the back- and forth movement of waves in a shallow near shore environments are called oscillation ripple marks and are usually symmetric. Mud cracks: These are polygonal shapes result from drying and shrinkage of mud (Fig.). They are found in shallow lakes and desert basins where mud is alternately wet and dry. 29 Physical Geology Prof. Dr. Rafa M.Zaki Fig. Show cross bedding and ripple marks Fig. Show mud cracks and graded bedding DEFORMATION (Secondary structures) Deformation is a general term that refers to all changes in volume and or shape of a rock body. Most crustal deformation occurs along plate’s margins, as they interact along their boundaries, tectonic forces deform the involved rock units. Stress is the force (compressional, tensional, and shear) acting on a body of rock. However, Strain is the response of a rock to stress. It generally involves a change in shape or volume of the rock. Rocks respond to deformation in various ways; at first they deform elastically (return to their original shape and size when 31 Physical Geology Prof. Dr. Rafa M.Zaki the stress is removed). Once the elastic limit is surpassed, rocks either deform plastically (don’t return to their original size and shape when the stress is removed) or fracture (Fig.). Plastic deformation is characteristic to ductile rocks and causes folding and flowing. Fracturing occurs when the rock is brittle and in this case faults are formed. Fig. Show the kinds of differential stress. If stress is not equal from all directions then we say that the stress is a differential stress. Three kinds of differential stress occur; 1. Tensional stress (or extensional stress), which stretches rock; 2. Compressional stress, which squeezes rock; and 3- Shear stress, which result in slippage and translation. 31 Physical Geology Prof. Dr. Rafa M.Zaki Stages of Deformation When a rock is subjected to increasing stress it passes through 3 successive stages of deformation. 1-Elastic Deformation -- wherein the strain is reversible. 2- Ductile Deformation -- wherein the strain is irreversible. 3-Fracture - irreversible strain wherein the material breaks. Geologic structures associated with the deformation are called folds, faults and joints. The study of such structures has an economic important especially most occurrence of oil and natural gas are associated with geologic structures that act to trap these fluids in valuable "reservoirs ". Rock fractures can also be the site of hydrothermal mineralization, which is a major source of metallicores. Moreover, the orientation and characteristics of rock structures must be considered when selecting sites for major construction projects such as bridges, hydroelectric dams and nuclear power plants. FOLDS Folds are structures formed during mountain building, where flat- lying sedimentary and volcanic rocks are bent into a series of wave-like undulations. Folds result from compressional stress and are either broad flexure (bending), in which rock units hundreds of meters thick have been slightly warped. Other folds are very tight microscopic structures such as those found in metamorphic rocks. Folds are found singly, but most often they occur as a series of undulations. 32 Physical Geology Prof. Dr. Rafa M.Zaki Geometry of Folds The parts of the fold are described by their form and orientation. These are; 1-Limbs are the two sides of the fold. 2- Fold axis is a line drawn along the points of maximum curvature of each layer. The fold axis is usually horizontal (parallel to the surface). However in more complex folding, it is often inclined at an angle known as the plunge angle. 3- Axial plane is an imaginary surface that divides a fold as symmetrically as possible. Fig. show the parts of fold TYPES OF FOLDS The folds known as anticlines and synclines; the anticline is a structure formed by the up folding or arching of rock layers (Fig. ). In the 33 Physical Geology Prof. Dr. Rafa M.Zaki anticline the oldest strata are found in the center and the two limbs dip away from each other. On the other hand, the syncline is a structure formed by the down folding of rock layers (Fig.). The youngest strata are found in the center and the two limbs 'dip towards each other. Anticlines and synclines are usually found in associations where the limb of an anticline is the limb of the adjacent syncline. There are different types of fold; 1- Symmetrical fold; when the limbs on either side of the axial plane diverge at the same angle. 2- Asymmetrical fold; when they diverge at different angles. 3- Overturned fold; is an asymmetrical fold in which one limb is lilted beyond the vertical. 4- Recumbent fold; is a fold in which the fold plane is horizontal. Folds don't continue forever; rather, their ends die out much like the wrinkles in cloth. Some folds plunge because the axis of the fold penetrates into the ground. When erosion removes the upper layers of the structures, the outcrop pattern of the plunging anticline points in the direction of plunging, the plunging synclinc points in the opposite direction of plunging. Monoclines are broad flexures (bendings) (hat have only one limb (Fig. ). They usJally 'result from vertical displacement and not from compressional stresses as the other folds. These monoclinal folds are produced from vertical faulting in deep-lying basement rocks. The rigid basement complex responds to vertical stress by fracturing, On the other hand folding deformed the relatively flexible sedimentary strata above these basement rocks. 34 Physical Geology Prof. Dr. Rafa M.Zaki Domes and basins are circular or elongated structures found in sedimentary rocks. They are formed as a result of broad up warping in basement rocks underlying such domes. Domes are also formed by the intrusion of magma (laccoliths) or upward migration of salt formation (salt domes). Basins on the other hand are down warped structures having a similar shape to domes. Basins are formed when large accumulations of sediments accumulate; the weight of these sediments caused the crust to subside. Some structural basins may have been the result of a large asteroid impact. Monocline: linear, strata dip in one direction between horizontal layers on each side. Anticline: linear, strata normally dip away from axial center, oldest strata in center. Syncline: linear, strata normally dip toward axial center, youngest strata in center. Chevron: angular fold with straight limbs and small hinges Dome: nonlinear, strata dip away from center in all directions, oldest strata in center. Basin: nonlinear, strata dip toward center in all directions, youngest strata in center. 35 Physical Geology Prof. Dr. Rafa M.Zaki Fig. Fold types. FAULTS Faults are fractures in rocks across which there has been some relative movement has occurred relative to a flat surface described as a fault plane. Parts of the fault are (Fig. ): 1-Hanging Wall and the Footwall: These are the two blocks on either side of an inclined fault. The hanging wall lies above the fault plane and the foolwall lies below. 2-Throw: This is the amount of relative displacement across a fault plane. 3-Strike of the fault: This is the geologic bearing of the line formed by the intersection of the fault with the horizontal plane. 4- The dip of the fault: This is the angle between the fault plane and the horizontal measured perpendicular to the strike. 36 Physical Geology Prof. Dr. Rafa M.Zaki Types of faults 1. Dip-slip faults: are faults that have an inclined fault plane and along which the relative displacement or offset has occurred along the dip direction. Normal Faults: are faults that result from horizontal tensional stresses in brittle rocks and where the hanging-wall block has moved down relative to the footwall block.. Reverse Fault: are faults that result from horizontal compressional stresses in brittle rocks, where the hanging-wall block has moved up relative the footwall block. A Thrust Fault is a special case of a reverse fault where the dip of the fault is less than 45o. Thrust faults can have considerable displacement, measuring hundreds of kilometers, and can result in older strata overlying younger strata. 37 Physical Geology Prof. Dr. Rafa M.Zaki. Reverse Fault: are faults that result from horizontal compressional stresses in brittle rocks, where the hanging-wall block has moved up relative the footwall block. 38 Physical Geology Prof. Dr. Rafa M.Zaki 1- Strike-slip fault: are faults where the relative motion on the fault has taken place along a horizontal direction and parallel to the strike of the fault surface. Such faults result from shear stresses acting in the crust. Strike Slip Faults - Strike slip faults can be of two varieties, depending on the sense of displacement.. Right-lateral slip fault, if the block on the other side has moved to the right.. Left-lateral slip fault, if the block on the other side has moved to the left. The famous San Andreas Fault in California is an example of a right-lateral strike-slip fault. Displacements on the San Andreas fault are estimated at over 600 km. 39 Physical Geology Prof. Dr. Rafa M.Zaki Horsts & Grabens - Due to the tensional stress responsible for normal faults, they often occur in a series, with adjacent faults dipping in opposite directions. In such a case the down-dropped blocks form grabens and the uplifted blocks form horsts. The East African Rift Valley is an example of an area where continental extension has created such a rift. The basin and range province of the western U.S. (Nevada, Utah, and Idaho) is also an area that has recently undergone crustal extension. In the basin and range, the basins are elongated grabens that now form valleys, and the ranges are uplifted horst blocks. Evidence of Movement on Faults Since movement on a fault involves rocks sliding past each other there may be left evidence of movement in the area of the fault plane. Fault Breccias are crumbled up rocks consisting of angular fragments that were formed as a result of grinding and crushing movement along a fault. When the rock is broken into clay or silt 41 Physical Geology Prof. Dr. Rafa M.Zaki size particles as a result of slippage on the fault, it is referred to asfault gouge. Slickensides are scratch marks that are left on the fault plane as one block moves relative to the other. Slickensides can be used to determine the direction and sense of motion on a fault. JOINTS Joints are fractures or discontinuities in a rock with no relative sliding or movement on either Side of a joint (Fig. ). They result from the release confining stresses. If the joints are planar, parallel, and very closely spaced, the fracturing process is called sheeting. If the joints are curved, concentric, parallel, and with uniform spacing, the fracturing process is termed exfoliation. Joints can also occur in igneous rocks as a result of cooling and called "columnar jointing” in which the rock cracks into columns with hexagonal cross section. Fig. : A: Columnar joints in basalt. 13: Three sets of joints 41 Physical Geology Prof. Dr. Rafa M.Zaki MOUNTAIN BUILDING AND THE EVOLUTION OF CONTINENTS The processes that produce mountain belts are called orogenesis. They include: 1-Folding Anticlines Sync lines 2-Thrust faulting 3-Metamorpliism 4-Igneous activity There are a number of types of mountains, including: 1. Volcanic mountains 2. Folded and thrust-faulted mountains 3. Fault-block mountains 4. Mountains formed as a result of erosion of less resistant surrounding rocks (such as unearthed plutons) The locations of important mountains as: 1. Appalachian Mountains 2. Cordillera (Rocky Mountains, Cascades, Sierra Nevada, Coast Ranges) 3. Andes Mountains 4. Himalayas Mountains 5. Alps 5. Ural Mountains\ 42 Physical Geology Prof. Dr. Rafa M.Zaki Causes of Mountain Building There are three primary causes of mountain building. 1. Convergence at convergent plate boundaries. 2. Continental Collisions. 3. Rifting Convergent Plate Margins when oceanic lithosphere subducts beneath continental lithosphere magmas generated above the subduction zone rise, intrude, and erupt to form volcanic mountains. The compressional stresses generated between the trench and the volcanic arc create fold-thrust mountain belts, and similar compression behind the arc create a fold-thrust belt resulting in mountains. Mountains along the margins of western North and South America, like the Andes and the Cascade range formed in this fashion. Island arcs off the coast of continents can get pushed against the continent. Because of their low density, they don't subduct, but instead get accreted to the edge of the continent. Mountain ranges along the west coast of North America formed in this fashion..Continental Collisions Plate tectonics can cause continental crustal blocks to collide. When this occurs the rocks between the two continental blocks become folded and faulted under compressional stresses and are pushed upward to form fold-thrust mountains. The Himalayan 43 Physical Geology Prof. Dr. Rafa M.Zaki Mountains (currently the highest on Earth) are mountains of this type and were formed as a result of the Indian Plate colliding with the Eurasian plate. Similarly the Appalachian Mountains of North America and the Alps of Europe were formed by such processes..Rifting Continental Rifting occurs where continental crust is undergoing extensional deformation. This results in thinning of the lithosphere and upwelling of the asthenosphere which results in uplift. The brittle lithosphere responds by producing normal faults where blocks of continental lithosphere are uplifted to form grabens or half grabens. The uplifted blocks are referred to fault-blockmountains. The Basin and Range province in the western United States formed in this manner, including the Sierra Nevada on its western edge and the Grand Tetons in Wyoming 44 Physical Geology Prof. Dr. Rafa M.Zaki CHAPTER FIVE MINERALS Minerals are useful materials in our life since every manufactured product contains materials obtained from minerals. For example, most car parts are made of iron, which is produced from the minerals hematite, magnetite, limonite and goethite. Chemical Properties of Minerals -Atom - The smallest particle of matter with constant properties. It consists of nucleus contains neutrons (o) and protons (+) and electrons (-) orbit the nucleus. In a stable atom, the number of electrons = the number of protons. -Atomic number - The number of protons in the nucleus determines the atomic number. Changing the number of protons changes the element. -Ion- A charged particle, due to a change in the number of electrons. Cationis a positively charged ion (has lost one or more electrons). Anion is a negatively charged ion (has gained one or more electrons). -Atomic weight (or mass number) = number of protons +number of neutrons. Chemical Bonding Ionic bonding: is the simplest form of chemical bond, it is formed by electrostatic attraction between ions of opposite charge, i.e, and it involves electron transfer e.g. Na +Cl. An electron has been transferred from the Na to the Cl. Covalent bonding: Compounds that achieve a stable electronic configuratipn by sharing electrons rather than gaining or losing them are 45 Physical Geology Prof. Dr. Rafa M.Zaki held together by covalent bonds. Metallic bonding: These are much weaker bonds than ionic or covalent bonds exist between all ions and atoms in solids. Here, electrons are free to move e.g. metals (conduction of electricity). A mineral is a solid, naturally occurring, inorganic substance that has an orderly internal structure and a definite (but not fixed) chemical composition (polymorphism). 1. Solid Solutions: Most minerals do not have a fixed chemical composition (quartz is a notable exception). Plagioclase feldspars are a mineral group in which composition varies between two extremes (albite is the sodium-rich end member and anorthite is the calcium-rich end member). Plagioclase is concentrated in igneous rocks from the oceanic crust. Alkali feldspars are members of a solid solution series between a potassium-rich end and a sodium-rich end and are concentrated in the continental crust. About 65% of the weight of the crust (both continenal and oceanic) is contributed by feldspar and about 15% of the continental crust is quartz. Olivine and pyroxene are both solid solution series and are common silicate minerals concentrated in the mantle. A few minerals form primarily near the surface and are concentrated in sedimentary rocks such as halite (salt), calcite (calcium carbonate – CaCO3) and dolomite (calcium and magnesium carbonate -CaMg(C03)2). 2. Polymorphism: Minerals which have the same chemical composition but different crystal structures are polymorphs. Graphite is a mineral, but coal is not a mineral. However, diamond 46 Physical Geology Prof. Dr. Rafa M.Zaki and graphite, for example, both are made up of 100% carbon and calcite and aragonite are both made up of calcium carbonate — CaCO"3 (Quartz is a mineral, but opal is not a mineral because it lacks an orderly internal structure.Oil is not a mineral because it is organic in origin. Physical Properties of Minerals The 4000 minerals discovered up till now are characterized each by certain physical properties which allow us to distinguish each mineral from the other. These are: I- Optical properties 1. Color is a diagnostic property for identifying minerals. Sulfur for example has a yellow color, and malachite has a bright green color. In many cases, color is not always diagnostic (feldspar, quartz, fluorite) where a mineral can have several colors (Fig. ) due to the presence of impurities in its crystalline structure, e.g. quartz is usually white but it has also other colors including pink, purple and even black. The mineral is colored or no color.The color is idiochromatic or allochromatic -Idiochromatic: come in just one color such as metallic and opaque minerals;silver (white), gold (golden yellow), copper (red) and malachite (green). -Allochromatic: many colors/many varieties, such as fluorite (CaF2) produce many colorsdue to impurities as rare earth elements or radium. 2-Luster is the appearance or quality of light reflected from the surface of a mineral. Metallic Luster: minerals that show the appearance of metals. 47 Physical Geology Prof. Dr. Rafa M.Zaki -Submetallic luster: minerals that appears partially metallic in luster. -Nonmetallic luster:light colored minerals which are either transparent or translucent are; A-glassy or vitreous: luster of broken glass, e.g. quartz B- Adamantine: Luster of diamond, minerals with high with high refractive index, high sp. Gr. and great hardness, e.g. diamond, Corundum. C- Resinous: luster of yellow resins, e.g. opal, sphalerite. D-Greasy: luster of oil or fats, e.g. nepheline. E- Pearly:luster of pearls. Exhibit by mineral with perfect cleavage,.g. Mica, talc. F: Silky: luster of silk.Minerals with fibrous crystal, e.g asbestos, satinspar. t- Dull or earthy: luster reflects light very poor and not shine. 3-Streak is the color of the powder produced by rubbing the mineral across a streak plate made of unglazed porcelain (Fig. ). The streak is not necessary to be same as the color of the mineral. For example some black minerals have a brown streak. The streak of a mineral is thesame whatever the colors of the mineral. Minerals with metallic luster have darker and denser streak than minerals with nonmetallic luster. 48 Physical Geology Prof. Dr. Rafa M.Zaki 4-Transparency: the amount of light transmitted or allowed to pass through minerals. A- transparent: when transmission of light is maximum and absorption is minimum. Objects can be seen. B- opaque: no transmitted light at all, e.g.metallic Minerals II- Cohesive properties 1- Tenacity: the resistance of mineral to breaking, crushing, bending, cutting. It is a mineral cohesiveness. A- brittle: a mineral that breaks and powdered easily, sulfides, calcite, Silicate and apatite. B- malleable: the mineral can be hammered out without breaking into thin sheet (native minerals, gold, silver, copper). C- sectile: the mineral that can be cut by knife into thin pieces (graphite and gypsum). D- ductile: a mineral that can be drawn into wires (silver, copper). E- flexible: a mineral that bends but retains it bent form. Does not resume its original shape(clay minerals, asbestos,chlorite). V- elastic: mineral that after bending returned to its original position (Muscovite) 2-Hardness is the degree of mineral resistance to scratching. It is determined by rubbing amineral with unknown hardness against one of known hardness or vice versa. Minerals with known hardness belong to 49 Physical Geology Prof. Dr. Rafa M.Zaki Mohs scale of hardness, Table (3). It consists of ten minerals arranged in order from 1 (softest) to 10 (hardest) as follows: Talc (1), gypsum-calcite, fluorite, apatite, orthoclase, quartz, topaz, corundum, and Diamond (10). So, a mineral with hardness 4.5 will scratch fluorite but is scratched by apatite. There are other objects of known hardness such as a fingernail (2.5), a copper penny (3), and a piece of glass 5.5. So, the mineral gypsum that has hardness (2) is scratched by the fingernail. Quartz (7) is the hardest of the common minerals and will scratch glass (5.5). Table (3): Mohs scale of hardness 2-Fracture is the shape of minerals that don’t have cleavage when broken. Some minerals break into smooth curved surface like broken glass, this is called conchoidal fracture (Fig. ) as in quartz, chert, obsidian and glass; others break into splinters or fibers but most minerals fracture irregularly. Kinds of fractures: 51 Physical Geology Prof. Dr. Rafa M.Zaki 1-concoidal: smooth surface (quartz, flint) 2-even: more or less smooth surface, may resemble cleavage, e.g. jasper. 3- uneven: rough and irregular surfaces, e.g. pyrite, hematite. 3-Cleavage is the tendency of a mineral to break along planes of weak bonding (related to crystal structure). Some minerals have one cleavage plane such as micas; some have four such as fluorite or three such as calcite. Quartz on the other hand has no cleavage (Fig. 23). The following are the different varieties of cleavage: -direction (muscovite, Biotite) -directions at 90° (feldspar, pyroxene) -directions not at 90° (amphibole at 60° and 120°) -directions at 90° (cubic) (halite, galena) -directions not at 90° (rhombohedral) (calcite, dolomite) -directions (octahedral) (fluorite) -directions (sphalerite) 4- Parting: is considered as a sort of pseudo-cleavage which leads to separation of some minerals along planes of twinning when pressure is applied. It is distinguish from cleavage by being present in twined Minerals and produced in all parts of their crystals. Only on the surfaces of twined families. Other Properties 1-Crystal form: A crystal is a solid substance that has regular faces resulting from an orderly arrangement of atoms (Fig. ). Minerals form 51 Physical Geology Prof. Dr. Rafa M.Zaki crystals with well-developed faces when they find space for crystal growth. When there is no space for crystal growth, they form inter-grown masses of crystals without a definite crystal, form. 2-Specific gravity is a number representing the ratio of the weight of a mineral to the weight of an equal volume of water. For example if a mineral weighs three times as much as an equal volume of water, its specific gravity is 3. 3-Electrical and Magnetic properties: Some minerals possess interesting electrical properties Pyroelectricity: minerals become electrified when subjected to a marked change in temperature, e.g. tourmaline.Light colored minerals develop positive and negative charges on the opposite ends when heated. Piezoelectricity: pressure induces electrical charge, e.g. quartz. 5- Magnetism:the property of minerals that allows attracting or repelling other magnetic materials, e.g. magnetite, pyrrohtite. Paramagnetic: attract other magnetic minerals Diamagnetic : repel other magnetic minerals 6- Taste: halite has a salty taste. 7- Smell: sulfur streak smells like rotten eggs. Pyrite (SO2), Arsenopyrite (Garlic) 8- Feel: Talc feels soapy and graphite feels greasy. 9- Chemical reactions to HCI: carbonates such as calciteeffervesce (fizz) with HCI. While, dolomite CaMg (C03)2 must be scratched and powdered to fizz. 10-Fluorescence: Some minerals glow' in the dark under a black light (U.V. light) due to excitation of electrons. 52 Physical Geology Prof. Dr. Rafa M.Zaki 11-Luminescence: some minerals glow or emit light when heated or exposed to in dark to ultra-violet or x-ray, e.g. fluorite (CaF2) , opal (SiO2.nH2O), diamond (C). 12-Phosphorescent, luminescence continuous even after excitation. 13-Play of colour, by turning mineral against light it appear to have several colours, labradorite, opal, diamond. The phenomena are sometimes explained by the presence of cleavage, twin lamellae, causing interference of reflected light. Mineral Groups 1-Native elements (metal): native gold (Au), native copper (Cu), native sulfur (S), native silver (Ag), graphite (C), diamond (C). 2-Silicates : quartz (Si02), potassium feldspar (KAlSiO8), Ca- Plagioclase feldspar (CaAbSi20g), Na-plagioclase feldspar (NaAISiO8). 3-Carbonates: calcite (CaC03), dolomite (CaMg(C03)2). 4-Oxides: water ice (H20), hematite (Fe203), magnetite (Fe304), corundum (AI2O3). 5-Halides: halite (NaCI), fluorite (CaF2). 6-Hydroxides: limonite (iron hydroxide), bauxite (aluminum hydroxide). 7-Sulfates: gypsum (CaS04.2H2O), anhydrite (CaS04), barite (BaS04). 8-Phosphates: apatite. 9-Sulfides: pyrite (FeS2), galena (PbS), sphalerite (ZnS). Other - borates, uranates. 53 Physical Geology Prof. Dr. Rafa M.Zaki 54 Physical Geology Prof. Dr. Rafa M.Zaki CHAPTER SIX ROCKS The minerals are the building blocks of rocks, i.e. the rock is an aggregate of one or more minerals. The rock is any solid mass of minerals or mineral like matter that occurs naturally as part of our planet. In other words, according to the number of the occurring minerals, rocks are classified into the following categories: 1-Monominerallic rock: a rock composed of one mineral, e.g. limestone is composed of the mineral calcite. 2-Polyminerallic rock: a rock composed of several minerals, e.g. granite is composed mainly of three minerals called quartz, feldspar and hornblende. 3-Nonminerallic rock: a rock composed on non-mineralic matter, e.g. Obsidian and pumice (non-crystalline glassy substances). There are three families of rocks: 1-Igneous rocks: are those rocks, which were formed from the cooling of molten material (magma or lava). 2-Sedimentary rocks: are those formed from the deposition of sediments. 3-Metamorphic rocks: are either igneous or sedimentary rocks subjected to temperature or pressure or both to form a new rock. The Rock Cycle The three major types of rocks are interrelated by a series of natural processes. Igneous rocks form from the cooling and crystallization of hot molten lava and magma. Igneous rocks undergo weathering and 55 Physical Geology Prof. Dr. Rafa M.Zaki erosion to form sediments. Sediments are deposited and lithified by compaction and cementation to form sedimentary rocks which becomes buried by additional deposition, and when they are deep within the Earth, they are subjected to heat and pressure, which causes them to become metamorphic rocks. With further burial and heating, the metamorphic rocks begin to melt. Partially molten metamorphic rocks are known as migmatite (Fig. ). As melting proceeds with increasing temperatures and depths of burial'-eventually the rock becomes molten and becomes magma, which cools and crystallizes to form plutonic igneous rock, or which is erupted onto the Earth's surface as lava, and cools and crystallizes to form volcanic igneous rock. These serial events are designated as the rock cycle (Fig. ) 1. IGNEOUS ROCKS The name igneous (fire-formed rocks) is derived from the Latin word (igneous) which means fire. This is because these rocks were formed from 56 Physical Geology Prof. Dr. Rafa M.Zaki cooling and solidification of the molten material either beneath the earth’s surface (magma) or when it risesup above the earth’s surface in the form of volcanoes (lava). Magma is the molten material beneath the earth’s surface. It is composed of liquid material with solid rocks and gases. Magma is formed by a process called partial meking within the earth’s crust and the upper mantle. This occurs at depths that may exceed 200km. Lava is similar to magma but loses its gases when erupts onto the Earth's surface through a volcano or crack (fissure). Lava cools more quickly than magma because it is receive on thesurface. Modes of igneous rock formation: -Intrusive or plutonic igneous rocks: Pluto means the god of the lower world. These areformed when magma loses its mobility before reaching the surface and crystallizes at depth. Plutonic Igneous rocks are exposed on the surface now as a result of uplift and erosion of portions of the earth’s crust. -Extrusive or volcanic igneous rocks: These are formed when molten- rock material (lava) rises up and solidifies at the earth’s surface during volcanoes. -Texture of igneous rocks: The word texture means the size and arrangement of minerals within the rock. Texture is important because it reflects the environment in which the rock formed. Cooling rates influence the texture if the igneous rock, where quick cooling leads to the formation of fine grains while slow cooling leads to the formation coarse grains. For example rocks formed beneath the earth’s surface consist of large crystals because of the slow 57 Physical Geology Prof. Dr. Rafa M.Zaki cooling of magma (tens or hundreds of thousands of years). On the other hand rocks formed at the earth’s surface consist of small crystals because of the rapid cooling of magma. Textures of igneous rocks: Aphanitic (fine- grained) Texture: the mineral grains (crystals) are too small as a result of rapid cooling, such as the case of basalt, rhyolite, and andesite (Fig. ). Fig. Aphanitic (fine- grained) Texture Phaneritic (coarse-grained) Texture: the mineral grains are larger and equal in size as a result of slow cooling, such as granite, diorite, and gabbro (Fig. ). Fig. : Phaneritic (coarse-grained) Texture 58 Physical Geology Prof. Dr. Rafa M.Zaki Porphyritic Texture: There are large crystals (phenocrystals) embedded in a matrix of small crystals (groundmass). This results from the differential crystallization when minerals crystallize at different temperatures and different rates. The rock that has this texture is termed porphyry. Porphyritic-phaneritic texture (Fig. ) implies a somewhat shallower (slightly cooler) location with continued fairly slow cooling such as granite porphyry or porphyritic granite. Porphyritic-aphanitic texture (Fig.) implies upward movement of magma from a deeper (hotter) location of extremely slow cooling such as andesite porphyryor porphyritic andesite, to a much shallower (cooler) location with fast cooling. Fig. : Porphyritic Texture Glassy Texture: There is no orderly crystal structure as a result of very rapid (instantaneous) cooling of magma that is rich in silica and has a high Viscosity (Fig.). 59 Physical Geology Prof. Dr. Rafa M.Zaki Fig.:Glassy Texture -Pegmatitic- very large crystals (many over 2 cm) such as granite pegmatite or pegmatitic granite. Fig.:Pegmatitic granite -Vesicular - contains tiny holes called vesicles, which formed due to gas bubbles in the lava or magma, very porous. May resemble a sponge. Commonly low density; may float on water (Fig. ). -Pyroclastic or Fragmental - pieces of rock and ash come out 61 Physical Geology Prof. Dr. Rafa M.Zaki of a volcano and get welded together by heat. May resemble rhyolite or andesite, but close examination shows pieces of fine- grained rock fragments in it (Fig.). May also resemble a sedimentary conglomerate or breccia, except that rock fragments are all fine-grained igneous or vesicular. Representatatives are Tuff (made of vojcanic ash) and volcanic breccia (contains fragments of fine-grained igneous rocks that are larger than ash). Fig. : Pyroclastic texture Igneous rock composition: Igneous rocks are composed mainly of silicate minerals. These silicate minerals consist of the eight elements Silicon, Oxygen, Sodium, Calcium, Potassium, Aluminum, Magnesium and Iron. Silicon and oxygen are the most abundant elements of these eight elements and together constitute about 98% 61 Physical Geology Prof. Dr. Rafa M.Zaki by weight of magma. In addition, magma contains small amounts of titanium and manganese and trace amounts of rare elements such as gold, silver and uranium. Silicate minerals, which are rich in iron and magnesium but low in silica, are called dark(or ferromagnesian) silicates. These minerals include olivine, pyroxene, amphibole and biotite.On the other hand, silicate minerals, which are rich in sodium, potassium and calcium and alsorich in silica, are called light silicates. These minerals include quartz, muscovite and feldspar group (orthoclase and plagioclase). Bowen’s Reaction Series: This is a diagram (Fig. ), which illustrates the sequence of minerals crystallization from a basaltic magma under laboratory conditions. As magma cools, the ferromagnesian minerals first crystallize (olivine followed by pyroxene, amphibole and biotite). At the same time calcium- rich feldspars also crystallize. With cooling the remaining magma becomes poor in iron and magnesium but rich in sodium, potassium and aluminum. Also it is rich in silica,which is little incorporated in the composition of the ferromagnesian minerals. As a result, light minerals such as quartz and muscovite crystallize. At the same time sodium-rich feldspars also crystallize. 62 Physical Geology Prof. Dr. Rafa M.Zaki Fig. : Bowen’s reaction series. Types of igneous rocks Mafic (basic) rocks Mafic: These are igneous rocks composed of ferromagnesian minerals (magnesium and iron silicates) like olivine and pyroxene as well as calcium-rich plagioclase. These minerals are rich in iron, magnesium or calcium and low in silicon. The mafic rocks are darker and denser than the other igneous rocks. They are characteristic of Earth's oceanic crust, Hawaiian volcanoes; forminga runny (low viscosity) lava Examples of these rocks include Gabbro (plutonic) and basalt (volcanic) (Fig. ). Felsic (acidic) rocks: Felsic: feldspar, silica (quartz). These are igneous rocks composed of potassium feldspar and quartz. They are lighter in color, dominated by silicon and aluminum (SiAl), and are: characteristic of continental crust. Examples include granite (plutonic) and rhyolite (volcanic) (Fig. ). 63 Physical Geology Prof. Dr. Rafa M.Zaki Intermediate Rocks: These are igneous rocks containing minerals found near the middle of Bowen’s reaction series like amphibole and plagioclase feldspar. Examples of intermediate igneous rocks include diorite (plutonic) and andesite (volcanic) (Fig. ). Ultramafic (ultrabasic) rocks: These are igneous rocks composed entirely of magnesium and iron silicates (ferromagnesian minerals), mostly of olivine and pyroxene (peridotite, Fig. ). They are believed to be major constituent of Earth's mantle and rarely observed on the Earth's surface. They are commonly found as xenoliths in basaltic lavas Examples include peridotite (the main constituent of the upper mantle). 64 Physical Geology Prof. Dr. Rafa M.Zaki 2. SEDIMENTARY ROCKS The word sediment means a loose particulate material (clay, sand, gravel, etc.). Sedimentary rocks are formed from deposition of sediments by processes of compaction and cementation. The word sedimentary is derived from the Latin word sedimentary, which means “settling” referring out to solid material from fluids (water or air). Sediments and sedimentary rocks Rock particles such as gravel, sand and mud, which result from weathering processes, are called sediments. Examples include sands forming the sand dunes, gravels in a streambed or mud on the floor of a swamp. Over long periods, these sediments accumulate; the materials near the bottom are compacted and finally cemented together by mineral matter (cement) deposited in the spaces between particles forming a 65 Physical Geology Prof. Dr. Rafa M.Zaki solid sedimentary rock. Sedimentary rocks are classified intothe following: -Detrital sedimentary rocks are sedimentary rocks formed from deposition of particles j resulted from weathering processes. -Chemical sedimentary rocks are sedimentary rocks formed from precipitation of solublematerial by inorganic or organic processes. -Organic Sedimentary Rocks (Coals) consists of rocks composed of organic matter (mainly plant fragments such as the coals. Because of this, they lack minerals (which must be inorganic, by definition). Other: There are several other interesting sedimentary rock types such as ironstones(oolitic hematite}, banded iron formations. 66 Physical Geology Prof. Dr. Rafa M.Zaki DETRITAL SEDIMENTARY ROCKS (also called terrigenous or clastic) A detrital sedimentary rock is a rock that is formed from grains; these grains are the products of mechanical and chemical weathering. The most common constituents of detrital rocks are clay minerals and quartz. Clay minerals are the most abundant product of chemical weathering of feldspars. Quartz is also abundant because it is very resistant to chemical weathering. Detrital sedimentary rocks are derived from the weathering of pre- existing rocks, which have been transported to the depositional basin. They have a clastic (broken or fragmental) texture (Fig. ) consisting of: -Framework elements (clasts) (larger pieces, such as sand or gravel) -Matrix (mud or fine-grained sediment surrounding the clasts) -Cement (the glue that holds it all together), such as calcite, iron oxide, silica etc. CLASSIFICATION OF DETRITAL SEDIMENTARY ROCKS Detrital sedimentary rocks are classified on the basis of particle size into the following: 67 Physical Geology Prof. Dr. Rafa M.Zaki Conglomerate or breccia: >2mm Conglomerate and breccia are similar in having grains more than 2mm in size but conglomerate is composed of rounded grains (Fig. ) whereas breccia (Fig. ) is composed of angular grains. Sandstone: 2 - 1/16 mm Sandstone is a detrital sedimentary rock consists of sand-sized grains (2 - 1/16 mm). Thedegree of similarity in particle size is called sorting (Fig). If all the grains are about the same size the rock is well sorted, on the other hand if the rock contains mixed large and small grains, it is poorly sorted. Sandstone is a good reservoir rock for the underground water. If quartz grains dominate the framework elements, the rock is quartz sandstone (also called quartz arenite, Fig. ). If dominated by feldspar grains the rock is arkose (Fig.). If dominated by sand-sized rock fragment grains, the rock is lithic sandstone (also called litharenite or greywacke, Fig. ). 68 Physical Geology Prof. Dr. Rafa M.Zaki 3. Shale or mudstone: < 1/16 mm Shale is usually fissile (able to split into thin layers) whereas mudstone is compact and breaks into blocks. Shale is the most common detrital sedimentary rock followed by sandstone then conglomerate or breccia. Shale is used as raw material for pottery, brick and china, mixed with limestone to make Portland cement, whereas oil shale will be a valuable energy resource in the future. -Shale is a fine-grained, fissile sedimentary rock consisting of silt and clay- sized particles < (1/16 mm) (Fig. ). Silt: Grain size between 1/256 and 1/16 mm (gritty), lithified rock is called Siltstone (Fig. 33B). Clay: Grain size less than 1/256 mm (smooth). The lithified rock is Claystone (if massive) or Shale (if fissile) (Fig. ). Note: Mud is technically a mixture of silt and clay. It forms a rock called mudstone (or mudshale if fissile). -It is deposited in quiet-water environment such as lakes, lagoons, swamps, river floodplains and portions of the deep-ocean basins. -Shale is impermeable and doesn’t allow fluids like water and petroleum to penetrate its microscopic tiny pores. Rocks that contain underground water are usually underlain by shale, which prevents downward movement. On the contrary, rocks that contain oil are capped by shale beds, which prevent oil and gas from escaping to the surface. 69 Physical Geology Prof. Dr. Rafa M.Zaki CHEMICAL AND BIOCHEMICAL SEDIMENTARY ROCKS This group includes the carbonates, the evaporates (limestones and dolostone), and the siliceous rocks. These rocks form within the depositional basin from chemical components dissolved in the seawater. These chemicals may be removed from seawater and made into rocks by chemical processes, or with the assistance of biological processes (such as shell growth). In some cases it is difficult to sort the two out (in carbonates or stone siliceous rocks, for example), so they are grouped together as chemical/biochemical. 1. Carbonates The carbonate sedimentary rocks are formed through both chemical and biochemical processes. They include the limestones (many types) and dolostones. The two dominant minerals in carbonate rocks are Calcite (CaC03) and Dolomite (CaMg(C03)2). Limestone is a rock that is composed chiefly of the mineral calcite (CaO) and is formed by inorganic as well as biochemical process. An example of limestone formed by biochemical processes includes coral reefs (Fig. ) which secrets huge calcareous skeletons such as the great 71 Physical Geology Prof. Dr. Rafa M.Zaki barrier reef of Australia (2000 km long). Another example is coquina, which is composed of poorly cemented shells and shell fragments. Another example of limestone formed by inorganic process is the travertine, which is deposited in caves when groundwater rich in CaCO3 losses dissolved CO2 after exposure to air causing CaCO3 to precipitate. Carbonate rock names: 1-Micrite (microcrystalline limestone) - very fine-grained; may be light gray or tan to nearly black in color. Made of lime mud, which is also called calcilutite. -Oolitic limestone (look for the sand-sized oolites) -Fossiliferous limestone (look for various types of fossils in a limestone matrix) -Coquina (fossil hash cemented together; may resemble granola) -Chalk (made of microscopic planktonic organisms such as coccolithophores. -Crystalline limestone -Travertine- composed of calcium carbonate (CaC03), and therefore, also technically a carbonate rock; travertine forms in caves and around hot springs. -Others - intraclastic limestone, pelleted limestone 71 Physical Geology Prof. Dr. Rafa M.Zaki The evaporites The evaporites (Fig. ) form from the evaporation of water (usually seawater). The most commonly encountered evaporates are the following: -Rock salt - composed of halite (NaCI). -Rock gypsum - composed of gypsum (CaSO4H2O). The siliceous rocks -The siliceous rocks are those, which are dominated by silica (SiO2). They commonly form from silica-secreting organisms such as diatoms, radiolarians, or some types of sponges. Chert is formed through chemical reactions of silica in solution replacing limestones. -Diatomite - looks like chalk, but does not fizz in acid. Made of microscopic planktonic organisms called diatoms. May also resemble kaolinile, but is much lowerin density and more porous). Chert - Massive and hard, microcrystalline quartz. May be dark or light in color. Oftenreplaces limestone. Does not fizz in acid. 72 Physical Geology Prof. Dr. Rafa M.Zaki ORGANIC SEDIMENTARY ROCKS This group consists of rocks composed of organic matter (mainly plant fragments). Because of this, they lack minerals (which must be inorganic, by definition). The following are the coals in order of increasing depth of burial (temperature and pressure): -Peat (porous mass of brownish plant fragments resembling peat moss). -Lignite (crumbly and black). -Bituminous coal (dull to shiny and black; sooty; layers may be visible). -Anthracite coal (extremely shiny and black, may have a sligfit golden shine; low density; not sooty; technically a metamorphic rock due to high temperatures and pressures to which it has been subjected). DIAGENESIS OF SEDIMENTS (TURNING SEDIMENT INTO SEDIMENTARY ROCK) Sediments are transformed to a sedimentary rock through a series of diagenetic processes (Fig. ), which are: -Lithiflcationis a process by which unconsolidated sediments are transformed into solid sedimentary rocks. This process requires two stages: compaction and cementation. -Compaction results when sediments accumulate through time and the weight of the overlying sediments compresses the deeper sediments causing reduction of the total volume as well as the pore spaces of the sediments. -Cementation follows compaction and results when cementing materials like calcite, silicaor iron oxides, dissolved in water, precipitate and fill the open spaces and join the particles together. 73 Physical Geology Prof. Dr. Rafa M.Zaki Fig. :Lithiflcation, compaction and cementation of sediments and formation of sedimentary rocks. METAMORPHIC ROCKS Metamorphic rocks are rocks that are transformed from igneous, sedimentary or even metamorphic rocks, by the effect of temperature, pressure or both. Metamorphism is the process, which causes this change (metamorphism means “changed form'"). This processalways occurs deep within the earth beyond our direct observation. The agents of metamorphism include heat, pressure and chemically active fluids. Those changes that occur are both textural and mineralogical. Rocks adjust to become more stable under new, higher temperatures and pressures. According to the degree of transformation, metamorphism may be of low-gradeor of high-grade.Under low-grade metamorphism, rocks like shale become a more compact metamorphic rockcalled slate. On the other hand high-grade metamorphism causes a transformation so complete that the identity of the original rock cannot be determined. Features as bedding planes, fossils and vesicles are obliterated. The zone of metamorphism extends from few kilometers below earth’s surface to the crust-mantle boundary. 74 Physical Geology Prof. Dr. Rafa M.Zaki Types of metamorphism -Contact metamorphism: occurs when rock is near or touching a mass of magma. In this case, changes are caused primarily by the high temperatures of the molten material (Fig. ). -Cataclastic metamorphism: This is the least common type of metamorphism and occurs along fault zone. Here the rock is broken and pulverized, as crustal blocks on opposite sides of a fault grind past each other (Fig. ). -Regional metamorphism: this occurs during mountain building processes where great quantities of rock are subjected to directed pressures and high temperatures causing large- scale deformation. Sources of the agents of metamorphism -Heat: There are several sources of heat for metamorphism. -Geothermal gradient: Temperature increases with depth at a rate of 20 - 30°C perkm in the crust. Increase of temperature and pressure with depth causes regional metamorphism. 75 Physical Geology Prof. Dr. Rafa M.Zaki Heat may come from large bodies of molten rock rising under a wide geographic area.Intrusions of hot magma can bike rocks as it intrudes them. Lava flows can also bake rocks on the ground surface. Lava or magma in contact with other rock causes contacts Metamorphism. Pressure -Burial Pressure. Pressure increases with depth due to the weight of the overlying rocks. A cubic foot of granite weighs 167.9 pounds. Increase of pressure and temperature with depth causes Regional Metamorphism.Regional metamorphism occurs at depths of 5 - 40 km. -Tectonic pressures associate with convergent plate boundaries and continental collision also causes Regional Metamorphism. Pressure along fault zones causes Dynamic Metamorphism, the crushing and ductile flow of rock. Rocks formed along fault zones are called myfbnites.. Chemical Fluids In some metamorphic settings, new materials are introduced by the action of hydrothermal solutions (hot water with dissolved ions). Many metallic ore deposits form in this way. -Hydrothermal solutions associated with magma bodies -Black smokers: Seawater percolates through newly formed oceanic crust, dissolving out metallic sulfide minerals. The hot seawater rises along fractures and pours from vents in the seafloor as black clouds of dark mineral-rich water. Sulfide minerals (such as pyrite, sphalerite, and 76 Physical Geology Prof. Dr. Rafa M.Zaki galena) and copper precipitate when the hot water comes in contact with cold seawater. Effect of metamorphism The processes of compaction and recrystallization change the texture of rocks during metamorphism. During compaction, the grains move closer together, the rock becomes more dense, and the porosity is reduced, e.g. the transformation of clay to shale to slate. During recrystallization, new crystals grow from the minerals already present. No changes in overall chemistry. A preferred orientation of minerals commonly develops under applied pressure. Platy or sheet-like minerals such as muscovite and biotite become oriented perpendicular to the direction of force. This preferred orientation is called foliation. The metamorphic processes cause many changes in rocks; these changes are either textural or mineralogical. Textural changes Texture as mentioned before means the size, shape and-distribution of particles that constitute a rock. Texture of metamorphic rocks is either foliated, nonfoliated or lineated. 77 Physical Geology Prof. Dr. Rafa M.Zaki Foliated texture Foliation is a broad term referring to the alignment of sheet-like minerals. Foliated texture is produced when rocks are subjected to pressure. Pressure causes not only realignment of mineral grains but also recrystallization and growth of larger crystals. In such case minerals are said to have preferred orientation. The new orientation will be perpendicular to the direction of the compressional force. The resultant mineral alignment usually gives the rock a layered or bandedtexture termed foliation. So, a foliated texture resultswhenever the minerals and structural features of a metamorphic rock are forced into parallel alignment. There are various types of foliation depending upon the grade of metamorphism and the mineralogy of the parent rock. The main types are: -Slaty: Under low-grade regional metamorphism, a fine- grainedsedimentary rock like shale is converted into a metamorphic rock called slate (Fig. ). The slate is more compact and thus denser. During this transformation the directed pressure and flattening causes the 78 Physical Geology Prof. Dr. Rafa M.Zaki microscopic clay minerals in shale to align into the more compact arrangement found in slate. At the same time the clay minerals recrystallize into minute mica flakes, these platy mica crystals become aligned so that their fiat surfaces are nearly parallel. Consequently, slate can be split into flat slabs; this property is called rock or slaty cleavage. -Phyllitic structure - alignment of fine-grained micas, as ina phyllite(Fig.). -Schistosity: This texture is formed under more extreme temperature- pressure regimes, where the very fine mica grains in slate will grow many times larger (Fig. ). This gives the rock a type of foliation called schistosity. The rock that has this texture is called schist such as talc schist or mica schist. -Gneissic texture: This texture is formed under high-grade metamorphism where ion migration is extreme enough to cause minerals to segregate (Fig. ). For example granite gneiss in which dark and light silicate minerals have separated giving the rock a banded appearance called gneissic texture. The rock that has this texture is called gneiss such as diorite gneiss and gabbro gneiss. -Lineation Lineation refers to the alignment of elongated, rod-like minerals such as amphibole, pyroxene, tourmaline, kyanite, etc. Lineation is a texture commonly seen in the metamorphic rock amphibolite derived from the metamorphism of basalt. -Nonfoliated texture Metamorphic rocks that don’t have a foliated texture are described as nonfoliated. They are composed of equidimensional grains such as 79 Physical Geology Prof. Dr. Rafa M.Zaki quartz or calcite. There is no preferred orientation. The grains form a mosaic. For.example marble (Fig.) which results from the metamorphism of limestone or dolostone is nonfoliated and quartzite (Fig. ), which resulted from the metamorphism of sandstone is also nonfoliated. Not all quartzites and marbles are pure. Some contain impurities that were originally mud inter-layered with or mixed with the original quartz sand or lime mud. These clay impurities metamorphose to layers of micas or other minerals, which may give marble (in particular) a banded, gneissic appearance, or which maygive a slight foliation to some quartzites. Mineralogical changes Mineralogical changes result from the recombination or reaction between the existing original minerals and the available ions in the water to form new minerals that are stable in the new environment. For example: the recrystallization of clay minerals in the shale to form mica. Examples of metamorphic rocks -Slateis a very fine-grained foliated rock, composed of minute mica flakes. It is produced from the low-grade metamorphism of shale and is characterized by its rock or slaty cleavage. Slate’s color depends on the mineral constituents; black slate contains organic material (carbon- bearing), red slate from iron oxides and green slate from chlorite (a mica- like mineral formed by the metamorphism of iron-rich silicates). -Schistis a strongly foliated rock that can be split into thin flakes or slabs. It contains mica minerals and amphibole. It is produced from a more extensive metamorphism of shale. Examples of schist include mica schist, graphite schist, talc schist and chlorite schist. 81 Physical Geology Prof. Dr. Rafa M.Zaki -Gneissis a banded metamorphic rock results from the high-grade metamorphism of shale or granite. Examples include granite gneiss and gabbros gneiss. -Marble is a coarse, crystalline nonfoliated rock results from the metamorphism of limestone. Pure marble is white in color; other colors of marble include pink, grey, green or black. These colors are due to the impurities present in the limestone. Marble is used as a building stone in banks and government buildings, to carve monuments and statues. -Quartziteis a very hard metamorphic rock formed from a moderate to high-grade metamorphism of quartz sandstone. 81 Physical Geology Prof. Dr. Rafa M.Zaki CHAPTER SEVEN WEATHERING Weathering is the disintegrationand decomposition of rocks in situ.Changing temperature, rainfall androck type have an importantinfluence on the type of weathering occurring In situ staying in the same place Disintegration breaking into smaller pieces Decomposition changing the chemicals which make up a rock The kinds of weathering are: 1-Mechanical weathering: This is the physical breakdown (disintegration) of rocks into smaller pieces without changing their mineralogical composition. 2-Chemical weathering: This is the chemical transformation of a rock into one or more new compounds. MECHANICAL WEATHERING Mechanical weathering as mentioned above means the disintegration of rocks into smaller pieces. 1-Frost wedging This is the repeated freezing and thawing of water in jointsand fractures of rocks. When water freezes, it expands about 9% of its volume. As a result, ice exerts tremendous outward pressure on the walls of fractures and joints causing their disintegration. Usually rocks are broken into angular (wedged) fragments, which accumulate into large piles at the base of steep slopes (cliffs) and cone-shaped accumulations known as talus slopes. 82 Physical Geology Prof. Dr. Rafa M.Zaki Fig. fractured along existing joints possibly by frost weathering or thermal stress exp