Cell Reproduction & Cell Cycle PDF
Document Details
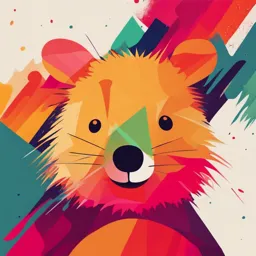
Uploaded by AdequateTimpani1511
Al-Azhar
Tags
Related
- 2023 JC1 Biology Lecture Notes - Cell Division & The Cell Cycle PDF
- Biology Module 1 Lesson 2: Cell Cycle and Cell Division (2022-2023) PDF
- CBSE Class 11 Biology - Cell Cycle and Cell Division PDF
- Cell Cycle and Cell Division (Class 11 Biology) PDF
- Biology: Cell Cycle and Cell Division Past Paper PDF
- General Biology 1 Past Paper Fall 2024 PDF
Summary
This document describes the processes of cell reproduction, including binary fission and mitosis. It covers the steps of DNA replication, chromosome segregation, and cytokinesis, explaining how these processes ensure the creation of genetically identical daughter cells. The document also highlights the cell cycle, detailing the stages of interphase and the mitotic phase in eukaryotic cells.
Full Transcript
Reproduction is the life process in which living things produce other living things of the same species. Cell reproduction is the process by which a cell divides to form two new cells (daughter cells) Types of cell reproduction (cell division) 1 - Prokaryotes (bacteria) Binary fi...
Reproduction is the life process in which living things produce other living things of the same species. Cell reproduction is the process by which a cell divides to form two new cells (daughter cells) Types of cell reproduction (cell division) 1 - Prokaryotes (bacteria) Binary fission - Divides forming two new identical cells 2 - Eukaryotes A - Mitosis - Organism growth - Replacement or repair of damaged cells B - Meiosis - Formation of sex cells, or gametes 1 - DNA overload - If cells grow without limit, an information crisis would develop - DNA cannot serve the needs of the increasing size of cell 2 - Exchange of materials - Food and oxygen have to cross membrane very quickly - Waste must get out If cell is too large, this occurs too slowly and cell will die Is the type of cell division that takes place in prokaryotes. Genetic material (nucleoid) in these cells is arranged in a single circular chromosome of the DNA. Genetic material is duplicated before division. As the prokaryote elongates, the two chromosomes attached to the plasma membrane also move apart, and once the two copies have separated (the original and replicate chromosomes), the cell divides, a process referred to as cytokinesis. Except in a case of mutation, the two resultant cells are identical. 3 main steps: A: DNA Replication - DNA is copied, resulting in 2 identical chromosomes B: Chromosome Segregation - 2 chromosomes separate, move towards ends (poles) of cell C: Cytokinesis (splitting of cells) - cytoplasm divides, forming 2 cells genetically identical to parent cell Cell division the process by which cells reproduce The cell cycle is a repeated pattern of growth and division that occurs in eukaryotic cells. Eukaryotic cells are more complicated given that they contain more organelles as compared to the prokaryotic cells. This cycle is also composed of all the steps required for the reproduction of a eukaryotic cell. In both unicellular and multicellular eukaryotes, cellular reproduction is a repeated process of growth, mitosis (nuclear division) and (usually, but not always) cell division (cytokinesis). The cycle of growth, mitosis, and cell division is called the cell cycle. Two fundamental processes occur with each cell cycle chromosomes replicate, and then they segregate equally to two daughter cells. The mechanisms by which these processes occur are similar in all eukaryotic cells. Processes occurring during the cell cycle are highly regulated and coordinated. The cell cycle is regulated primarily at the DNA replication and mitosis steps. In the absence of regulation, cells replicate and divide uncontrollably, leading to diseases such as cancer. The duration of the cycle, however, varies from organism to organism and cell to cell. Both the initiation and inhibition of cell division are triggered by events external to the cell when it is about to begin the replication process. 1. An event may be as simple as the death of a nearby cell or release of growth- promoting hormones, such as human growth hormone (HGH). A lack of HGH can inhibit cell division, resulting in dwarfism, whereas too much HGH can result in gigantism. Crowding of cells can also inhibit cell division. 2. Another factor that can initiate cell division is the size of the cell; as a cell grows, it becomes ineffective due to its decreasing surface-to-volume ratio. The solution to this problem is to divide. Whatever the source of the message, the cell receives the signal, and a series of events within the cell allows it to proceed into mitosis. To divide, a cell must complete several important tasks: it must grow, copy its genetic material (DNA), and physically split into two daughter cells. Cells perform these tasks in an organized steps that make up the cell cycle. The cell cycle is a cycle, rather than a linear pathway, because at the end of each go-round, the two daughter cells can start the exact same process over again from the beginning. In eukaryotic cells, or cells with a nucleus, the stages of the cell cycle are divided into two major phases: interphase and the mitotic (M) phase. During interphase, the cell grows and makes a copy of its DNA. During the mitotic (M) phase, the cell separates its DNA into two sets and divides its cytoplasm, forming two new cells. Interphase (known as the resting phase of the cell cycle). It occupies around 95% time of the overall cycle. Period of growth and DNA replication between cell divisions. The interphase is divided into three phases:- 1 - G1 phase (Gap 1) Is the phase of the cell between mitosis and initiation of replication of the genetic material of the cell. During this phase, the cell is metabolically active and continues to grow without replicating its DNA. cell increases in size 2 - S Phase (Synthesis) DNA replication takes place during this phase. Replication of chromosomes. 3 - G2 phase (Gap 2) organelles double new cytoplasm forms All other structures needed for mitosis form During this phase, the RNA, proteins, other macromolecules required for multiplication of cell organelles, spindle formation, and cell growth are produced as the cell prepares to go into the mitotic phase. Put another way, chromosome replication takes place In interphase and then mitosis occurs, resulting in the distribution of a complete chromosome set to each of two progeny nuclei. Different cells take different lengths of time to complete the cell cycle. In a given organism, variation in the length of the cell cycle depends mainly on the duration of G1. The duration of S plus G2 plus M is approximately the same in all cell types. For example, some cancer cells and early fetal cells of humans spend minutes In G1; whereas some differentiated adult cells (such as nerve cells) spend years in G1. Some cells exit the cell cycle from G1 and enter a dormant, non-dividing state called G0 (like heart and nerve cells). G0 cell is not actively preparing to divide, it’s just doing its job. For instance, it might conduct signals as a neuron or store carbohydrates as a liver cell. G0 is a permanent state for some cells, while others may re-start division if they get the right signals. Mitosis = nuclear division The steps of mitosis ensure that each new cell has the exact same number of chromosomes as the original. The mitotic phase is divided into four overlapping stages. The relative time spent in each of the four stages of mitosis varies greatly among cell types. Mitosis is followed by cytokinesis (cell division) In cytokinesis, the cytoplasm of the cell is split in two, making two new cells. Cytokinesis usually begins just as DNA found on chromosomes located in nucleus of cell Cell cycle continuous process – Cells grow – DNA replicated – Organelles duplicated – Divide to form daughter cells – 2 Main steps: 1: Mitosis (4 steps - Prophase, Metaphase, Anaphase, Telophase) Nucleus divides 2: Cytokinesis - Cytoplasm divide, forming 2 cells Each new daughter cell is genetically identical to parent cell Mitosis is a process where a single somatic cell (non-reproductive cells) divides into two identical daughter cells (cell division) in eukaryotic organisms. During mitosis one cell divides once to form two identical daughter cells. Continuous process ensure each new daughter cell has the exact same number of chromosomes as the original (parent cell). The major purpose of mitosis is for growth and to replace damaged out cells. If not corrected in time, mistakes made during mitosis can result in changes in the DNA, that can potentially lead to genetic disorders. The mitotic phase is divided into four overlapping stages (PMAT): 1 - Prophase 2 - Metaphase 3 - Anaphase 4 - Telophase 1 - Chromosomes which was duplicated during the preceding S phase of interphase condensed and become visible. 2 - Due to DNA replication during interphase, each chromosome consists of two identical sister chromatids connected at the centromere. 3 - Centrioles migrate to the opposite poles of cell (only in animals) ; higher plant cells usually lack centrioles, but they do have a mitotic spindle. 4 - Spindle fibers begin to form and attach to chromosomes near the centromere. 5 - Phase ends with the breakdown of the nuclear membrane The nuclear membrane disappears. The developing spindle enter the nuclear area. Chromosomes are more condensed. A specialized multiprotein complex called a kinetochore binds to each centromere. The kinetochores are the sites for the attachment of the chromosomes to spindle microtubules known as kinetochore microtubules. For a pair of sister chromatids, one to many kinetochore microtubules from one pole attach to the kinetochore of one chromatid, and an equivalent number of kinetochore microtubules from the other pole attach to the kinetochore of the other chromatid. 1 - The centrioles are now at opposite poles of the cell with the mitotic spindle fibers extending from them. 2 - The developing spindle enters the former nuclear area. 3 - Kinetochores, which are specialized regions in the centromeres of chromosomes, are the sites for the attachment of the chromosomes to spindle microtubules known as kinetochore microtubules. 4 - The chromosomes line up carefully end-to-end along the center (equator) of the cell. 5 - The mitotic spindle fibers attach to each of the sister chromatids. 1 - The paired chromosomes (sister chromatids) separate and begin moving to opposite ends (poles) of the cell. 2 - Once the paired sister chromatids separate from one another, each is considered a "full" chromosome. 4 - The daughter chromosomes migrate centromere first and the kinetochore fibers become shorter as the chromosomes near a pole. 5 - In preparation for telophase, the two cell poles also move further apart during the course of anaphase. At the end of anaphase, each pole contains a complete compilation of chromosomes. 1 - The two sets of chromosomes are assembled into two groups at opposite ends of the cell. 2 - A membrane forms around each set of chromosomes to create two new nuclei. 3 - Spindle microtubules disappear. 4 - Nucleoli also reappear. 5 - After these changes, telophase/mitosis is completed. The genetic contents of one cell have been divided equally into two; the chromosomes are segregated into distinct new nuclei in the emerging daughter cells. Cytokinesis is division of the cytoplasm. Cytokinesis follows the nuclear division stage of mitosis. Cytokinesis sorts the two new nuclei into separate daughter cells, finishing mitosis and cell division. In cytokinesis in animal cells, a constriction forms in the middle of the cell; the constriction continues until two daughter cells are produced. In cytokinesis in plant cells, a new cell membrane and cell wall are accumulated between the two new nuclei to form a cell plate. Cell wall material coats each side of the plate and the result is two progeny cells. Chromosome Appearance & Phase Important Events Location DNA replication, cell grows and Interphase DNA copies itself; chromatin replicates organelles Nuclear envelope disappears, spindle Prophase Chromosomes coil up fibers form Chromosomes line up in the middle Spindle fibers connect to chromosomes Metaphase Chromosome copies divide and Spindle fibers pull chromosome copies Anaphase move apart apart to opposite poles Nuclear envelopes reform, 2 new nuclei Telophase Chromosomes uncoil back into are formed, spindle fibers disappear chromatin Division of the rest of the cell: Cytokinesis Chromatin cytoplasm and organelles Organisms control cell growth and division very carefully (way to increase number of cells and size of organism). This provides the replacement of cells that wear out or are broken down. Protein group known as Cyclins regulates the cell cycle. Cyclins regulate the timing of the cell cycle in eukaryotic cells. Two main groups of protein regulators: 1. Internal Regulators: respond to events inside the cell. Ex. Make sure cell doesn’t enter mitosis until chromosomes have all replicated 2. External Regulators: respond to events outside the cell. Ex. Embryonic growth and healing Regulatory cyclins control the cell cycle at checkpoints. Checkpoints determine whether cell cycle proceeds or is halted. checks to make sure cell is ready to keep going, checking for errors in replication, growth or division. Checkpoints at G1, S and M G1 Checkpoint - decides whether or not cell will divide S Checkpoint - determines if DNA has been properly replicated Mitotic Spindle - Checkpoint - ensures chromosomes are aligned at mitotic plate When good cells go bad: Cancer cells ignore normal signals of cell cycle and undergo rapid propagation 1 - Cell Growth is so controlled because when it is not controlled things go very, very wrong. 2 - Cancer is a disorder in which some of the body’s own cells lose the ability to control growth. 3 - Cancer cells do not respond to the signals that regulate the growth of most cells. 4 - When cells divide uncontrollably they form masses of cells called tumors that can damage the surrounding tissue. 5 - Cancer cells can break off and spread throughout the body disrupting normal activities and causing serious medical problems or even death. 6 - Cancer is a disease of the cell cycle. Result of uncontrolled cell division of cells that have lost ability to regulate cell cycle Reproduce more rapidly than normal cells Cancer cells do not carry out necessary functions. Cancer cells come from normal cells with damage to genes involved in cell-cycle regulation. Masses formed called ‘tumors’ Malignant tumors are cancerous – these cells break loose and can invade and destroy healthy tissue in other parts of the body (called metastasis) Benign tumors remain clustered, not cancerous, cells do not spread to other parts of the body and can be removed. Standard cancer treatments typically kill both cancerous and healthy cells. normal cell cancer cell bloodstream The cell cycle is a repeating series of events, characterizing the life of a eukaryotic cell. Binary fission is a form of cell division in prokaryotic that produces identical offspring. As a eukaryotic cell prepares to divide, the DNA and associated proteins coil into a structure, known as a chromosome. The DNA copies during the S phase of the cell cycle, resulting in a chromosome that consists of two identical chromatids, attached at a region called the centromere. The cell cycle has five phases: the first growth (G1) phase, the synthesis (S) phase, the second growth (G2) phase, mitosis and cytokinesis. Mitosis is the division of the nucleus; four distinct phases of mitosis have been recognized: prophase, metaphase, anaphase, and telophase. Cytokinesis is the division of the cytoplasm. The cell cycle is controlled through specific mechanisms. Cancer results from uncontrolled cell division, due to the loss of regulation of the cell cycle. Genetics Genetics the scientific study of genes and heredity. It includes the study: What are genes How genes carry information. how genes replicated and passed to subsequent generations of cells. How gene expression of their information within an organism determines the particular characteristics of that organism. The genetic information in a cell is called the genome. Heredity is the transmission of genetic information and traits (such as eye color and an increased chance of getting a certain disease) from parents to offspring. Through heredity, variations between individuals can accumulate and cause species to evolve by natural selection. Genome - complete set of DNA instructions found in a cell. In humans, the genome consists of 23 pairs of chromosomes(in nucleus) 1 chromosome (in mitochondria) In plants, there are three genomes: 1 - In the nucleus 2 – In the mitochondria 3 – In the plastids Genotype & Phenotype Genotype Phenotype It is the s genetic makeup of an It is the actual, expressed organism. properties, such as the the information that codes for organism's ability to all the particular characteristics perform a particular of the organism. chemical reaction. The genotype represents Phenotype is the potential properties, but not the appearance of genotype. properties themselves. The observable expression of genes producing distinguished characteristics in individual. Chromosomes ▪ Structures containing DNA that physically carry hereditary information. Chromosomes contain the genes. Genes ▪ Segments of DNA (except in some viruses, in which they are made of RNA) that code for functional products. ▪ The basic physical and functional unit of heredity passed from parent to child. Chromatin Substance within a chromosome consisting of DNA and protein. The repeating (building blocks) unit of chromatin is the nucleosome, The major proteins in chromatin are histones (which help package the DNA in a compact form that fits in the cell nucleus). The compaction of genomic DNA into chromatin has profound implications for the regulation of key processes such as transcription, replication and DNA repair. Chromosomes Carry the Genes Gene A Gene B Gene C Gene D Gene E Gene F Chromosome The genes belonging to each cell are arranged on chromosomes. Lower organisms fit all their genes onto a single chromosome. higher organisms need several chromosomes to accommodate all their genes. Haploid and Diploid Organisms CopyCopy 1 1 Gene A1 Gene B1 Gene C1 Gene D1 Gene E1 Gene F1 Chromosome Duplicated Genes Gene A2 Gene B2 Gene C2 Gene D2 Gene E2 Gene F2 Copy 2 Lower organisms like bacteria have only a single copy of each gene (haploid organisms). Higher organisms have duplicate copies of each gene (diploid organisms). In some cases, there are some cells with more than two copies of each gene (triploid, tetraploid,……..etc.). The genes located at the same locus on homologous chromosomes. Allele: is one of two or more versions of a gene. Alleles are alternative forms of a gene and causes different phenotypic expression of a given trait. When A gene exists in the same locus with more than two alleles and controlled the single type of phenotypic trait is called as multiple alleles. Homozygous and Heterozygous An individual inherits two alleles for each genetnerap hcae morf eno ,. If the two alleles are the same si laudividni eht ,homozygous for that gene. If the two alleles are different si laudividni eht ,heterozygous for that gene. Homozygous and Heterozygous R R R r Homozygous Dominant Heterozygous (RR = red flowers) (Rr = red flowers) r r r R Homozygous recessive Heterozygous (rr = white flowers) (rR = red flowers) Dominant allele: The allele whose properties are expressed as the phenotype. Recessive allele: The allele whose properties are not observed because they are masked by the dominant allele. Homozygous: Having two identical alleles of the same gene. Heterozygous: Having two different alleles of the same gene. Nucleic acids In 1944, American microbiologists Oswald Avery, Colin Macleod and Maclyn Mccarty discovered that a substance called deoxyribonucleic acid (DNA) is the substance of which genes are made. While the abbreviations DNA and RNA are considered standard nomenclature these days, their names underwent a long evolution, from nuclein to thymus nucleic acid to nucleic acid to deoxyribonucleic acid and ribonucleic acid. DNA was first called thymus nucleic acid because it was isolated from thymus gland of cattle, while RNA first identified as yeast nucleic acid. Nine years later, James Watson and Francis Crick, working with molecular models and X-ray information supplied by Rosalind Franklin, identified the physical structure of DNA. Crick suggested a mechanism for DNA replication and how it works as the hereditary material. Nucleic acids are a long chain or polymer of repeating subunits, called nucleotides. DNA is composed of two chains of nucleotides that form a double helix shape. The two strands are antiparallel. The complimentary nitrogenous bases form hydrogen bonds between the strands. A is complimentary to T and G is complimentary to C. The backbone of the DNA molecule is composed of alternating phosphate groups and sugars. Chemical and Physical Structure of DNA deoxyribonucleic acid DNA ❖DNA is along chain of repeating units called nucleotides. ❖DNA is a polymer. The monomer units of DNA are nucleotides, and the polymer is known as a (polynucleotide). ❖Each nucleotide is composed of three parts: - 1.Five-Carbon Sugars (Pentose). 2.Base (Nitrogenous bases). 3.Phosphate group. There are four different types of nucleotides found in DNA, differing only in the nitrogenous base. The four nucleotides are given one letter abbreviations as shorthand for the four bases. A is for adenine G is for guanine C is for cytosine T is for thymine DNA is packaged in chromosome Nucleotide subunits of DNA contain the sugar deoxyribose. Nucleotide subunits of RNA contain a pentose (five-carbon) sugar called ribose. The sugars differ only in the presence or absence (“deoxy”) of an oxygen in the 2′ position. This minor distinction between RNA and DNA dramatically influences their function. The five carbon atoms in each pentose sugar are assigned numbers 1′ through 5′. Primes are used in the numbering of the ring positions in the sugars to differentiate them from the ring positions of the bases. 2 - Nitrogenous Bases The bases are nitrogen- containing molecules having the chemical properties of a base (a substance that accepts an H+ ion or proton in solution). Two of the bases, adenine (A) and guanine (G) have a double carbon–nitrogen ring structure; these are called purines. The other three bases, thymine (T), cytosine (C), and uracil (U) have a single ring structure; these are called pyrimidines. Thymine is found in DNA only, while uracil is specific for RNA. The phosphate functional group (PO4) gives DNA and RNA the property of an acid (a substance that releases an H+ ion or proton in solution) at physiological pH, hence the name "nucleic acid". The linking bonds that are formed from phosphates are esters that have the additional property of being stable. Further, after the phosphodiester bond is formed, one oxygen atom of the phosphate group is still negatively ionized. The negatively charged phosphates are extremely insoluble in lipids, which ensure the retention of nucleic acids within the cell or nuclear membrane. A few years before Watson and Crick proposed the three- dimensional structure of DNA, Erwin Chargaff developed a paper chromatography method to analyze the amount of each base present in DNA. Chargaff observed certain regular relationships among the molar concentrations of the different bases. These relationships are now called Chargaff's rules. 1- The base composition of the DNA of an organism is constant throughout all the somatic cells of that organism and is characteristic for a given species. 2- The base composition of DNA varies from an organism to another which is expressed by the dissymmetry ratio, (A+T)/(G+C) i.e. differing base compositions among organisms are reflected by variance in their dissymmetry ratio. 3- The base composition in a given species does not change with age, nutritional state, or changes in the environment. 4- The amount of adenine in the DNA of a given organism is always equal to the amount of thymine (A=T). 5- The amount of purine bases in the DNA of a given organism is always equal to the amount of pyrimidine bases [A] + [G] = [T] + [C]. The base composition of DNA, defined as the “percent G + C,” differs among species but is constant in all cells of an organism within a species. The G + C content can vary from 22 to 73%, depending on the organism. Nucleoside and nucleotide A DNA or RNA chain is formed in a series of three steps. 1 - In the first reaction, each base is chemically linked to one molecule of sugar at the 1′-carbon of the sugar, forming a compound called a nucleoside. 2 - When a phosphate group is also attached to the 5′-carbon of the same sugar, the nucleoside becomes a nucleotide. 3 - Finally, nucleotides joined (polymerized) by condensation reactions to form a chain. The hydroxyl group on the 3′-carbon of a sugar of one nucleotide forms an ester bond to the phosphate of 5′-carbon of another nucleotide, eliminating a molecule of water. This chemical bond linking the sugar components of adjacent nucleotides is called a phosphodiester bond, or 5′→3′ phosphodiester bond, indicating the polarity of the strand. Nucleotides may contain one phosphate unit (monophosphate), two such units (diphosphate), or three (triphosphate). When free in the cell pool, nucleotides usually occur as triphosphates. The triphosphate form serves as precursor building block for a DNA or RNA chain during synthesis. When incorporated into a nucleic acid chain, a nucleotide contains one each of the three components. Double helix is the description of the structure of a DNA molecule. 1 - DNA consists of two strands that wind around each other like a twisted ladder. 2 - alternating sugar (deoxyribose) and phosphate groups form the outside backbone of the ladder (helix). 3 - The rungs of the ladder are formed by complementary pairs of nitrogen bases. 4 - The two DNA strands are complementary and antiparallel. 5 - The helix diameter is 2.0 nm, Adjacent base pairs are spaced by 0.34 nm. DNA is Complimentary The inner portion of a DNA molecule is composed of a series of 4 nitrogenous bases: adenine (A), guanine (G), thymine (T), and cytosine (C). These bases are non-polar and therefore hydrophobic (they don't like water). Inside a DNA molecule these bases pair up, A to T and C to G, forming hydrogen bonds that stabilize the DNA molecule. Because the interior bases pair up in this manner, we say the DNA double helix is complimentary. This sequence of bases inside the DNA double helix that we refer to as the genetic code. DNA is Antiparallel 1 - The two strands of DNA have opposite chemical polarity, or, their sugar-phosphate backbones run in opposite directions. 2 - Direction in nucleic acids is specified by referring to the carbons of the ribose ring in the sugar-phosphate backbone of DNA. ( where, 5' specifies the 5th carbon in the ribose ring, and 3' specifies the 3rd carbon in the ring). 3 - Direction of DNA molecules is specified relative to these carbons (for example, transcription always occurs in the 5' to 3' direction. Nucleic acid polymerization cannot occur in the opposite direction, 3' to 5', because of the difference in chemical properties between the 5' phosphate group and the 3'ring-carbon with an attached hydroxyl group. The ends of a DNA or RNA chain are distinct and have different chemical properties. The two ends are designated by the symbols 5′ and 3′. The symbol 5′ refers to the carbon in the sugar to which a phosphate (PO4) functional group is attached. The symbol 3′ refers to the carbon in the sugar ring to which a hydroxyl (OH) functional group is attached. This 5′→3′ directionality of a nucleic acid strand is an extremely important property of the molecule. Understanding this directionality (polarity) is critical for understanding aspects of replication and transcription, for reading a DNA sequence and for carrying out experiments in the lab. By convention (agreement), a DNA sequence is written with the 5′ end to the left, and the 3′ end to the right. This is also the direction of synthesis. Features of the DNA Double Helix 1- Two DNA strands form a helical spiral, winding around a helix axis in a right-handed spiral. 2- Within the DNA double helix, A forms 2 hydrogen bonds with T on the opposite strand, and G forms 3 hyrdorgen bonds with C on the opposite strand. 3 - dA-dT and dG-dC base pairs are the same length, and occupy the same space within a DNA double helix. Therefore the DNA molecule has a uniform diameter. 4 - dA-dT and dG-dC base pairs can occur in any order within DNA molecules. 5 - One very important consequence of nitrogen-containing base pairing is that if the sequence of bases of one strand is known, then the sequence of the other strand is also known. B-DNA structure 1 - It is the most stable and predominant form under physiological conditions. 2 - It has alpha helix (a right-handed or clockwise), spiral. 3 - It possesses approximately 10 base pairs per 360 degree rotation of the helix. 4 - This structure exist when plenty of water surrounds the molecule. Z-DNA structure 1- In this DNA form, the sugar-phosphate backbones zigzag back and forth, giving rise to the name Z-DNA (for zigzag). 2 - It has a left-handed, reverse to B-DNA structure 2 - Z-DNA structures arise under physiological conditions when particular base sequences are present, such as stretches of alternating C and G sequences. 3 – Z-DNA may play a role in regulating gene transcription. A-DNA structure 1 - A-DNA it is formed from B-DNA under dehydration condition (less water is present). Dehydrated DNA takes an A form that protects the DNA during extreme conditions such as desiccation. 2 - It has a right-handed, like B-DNA structure 3 - A-DNA is much shorter, flatter and wider than B-DNA. The central dogma of molecular biology The central dogma states that genetic information passes from DNA to protein in one way. It indicates that genotype code for phenotype but phenotype can not code for genotype. Its include three general pathways 1. Replication 2. Transcription 3. Translation The Flow Of Genetic Information 1.DNA Replication makes the possible the flow of genetic information between sequence generation. 2. The DNA of a cell replicates before cell division so that each offspring cell receives a chromosome identical to the parent's. 3. The genetic information contained in DNA also flows in another way: it is transcribed into mRNA and then translated into protein. 4.The organism shows its properties through process of translating it into proteins. Mechanisms for transfer of information in the cell 1- It should be present in every cell. 2 - It should be able to store (keep) information in the coded form for the control of biological functions of the cells. 3 - Genetic material should chemically and structurally be stable. 4 - The genetic material should be able to generate its own kind (replication) and also new kinds of molecules. 5 - It should provide the scope for slow mutations that are required for evolution. 6 - Genetic expression: It should be able to express itself in the form of a Mendelian character. 7 - It should be capable of differential expression so that the various parts of an organism may acquire specific form, structure and functions in-spite of having the same genetic material. DNA makes DNA (DNA Replication) DNA replication is the process by which a double-stranded DNA molecule is copied to produce two identical DNA molecules. Replication is an essential process because it correlated to cell division. DNA replication of the mother cell is duplicated to give two identical copies. The replication process relies on the fact that each strand of DNA can serve as a template for duplication. DNA replication initiates at specific points, called origins, where the DNA double helix is unwound. Mode of DNA Replication There were three models for how organisms might replicate their DNA. 1 - Conservative replication In this model, DNA replication results in one molecule that consists of both original DNA strands and another molecule that consists of two new strands (with exactly the same sequences as the original molecule). 2 - Semi-conservative replication In this model, the two strands of DNA unwind from each other, and each acts as a template for synthesis of a new complementary strand. This results in two DNA molecules with one original strand and one new strand. 3 - Dispersive replication In the dispersive model, DNA replication results in two DNA molecules that are mixtures, or “hybrids,” of parental and daughter DNA. In this model, each individual strand is a patchwork of original and new DNA. The term chromosome comes from the Greek words (chroma, "colour") and (soma, "body"), describing their strong staining by particular dyes used in research. Chromosomes vary in number and shape among living organisms. Most bacteria have one or two circular chromosomes. Humans, along with other animals and plants, have linear chromosomes that are arranged in pairs within the nucleus of the cell. The principal feature that distinguishes a eukaryotic cell from a prokaryotic cell is the presence of a membrane- bound nucleus. Most eukaryotic cells, DNA (or genome) is arranged in multiple linear chromosomes. A chromosome consists of a single double-helix DNA molecule wrapped around histone protein, giving the chromosome a more compact shape. Number of chromosomes typically is characteristic of the species. Many eukaryotes have two copies of each type of chromosome in their nucleus, so their chromosome complement is said to be diploid, or 2N. Diploid eukaryotes are produced by the fusion of two haploid gametes, one from the female parent and one from the male parent. Each gamete has only one set of chromosomes and is said to be haploid (N). The fusion produces a diploid zygote, which then undergoes embryological development. Examples of diploid organisms are humans, with 46 chromosomes (23 pairs), and Drosophila melanogaster, with 8 chromosomes(4 pairs). In diploid organisms, the members of a chromosome pair that contain the same genes and that pair during meiosis are called homologous chromosomes; each member of a pair is called a homolog, and one homolog is inherited from each parent. Chromosomes that contain different genes and that do not pair during meiosis are called nonhomologous chromosomes. Sex chromosomes—the chromosomes that are represented differently in the two sexes in many eukaryotic organisms. One sex has a matched pair of sex chromosomes, and the other sex has an unmatched pair of sex chromosomes or a single sex chromosome. For example, human females have two X chromosomes (XX), whereas human males have one X and one Y (XY). Chromosomes other than sex chromosomes are called autosomes. chromosomes are seen to differ in size and morphology within and between species. Each chromosome has a constriction along its length called a centromere, which is important for the behavior of the chromosomes during cellular division. The location of the centromere in one of four general positions in the chromosome is useful in classifying eukaryotic chromosomes. 1. Metacentric chromosome: centromere is present in the middle (center)and divides the chromosome into two approximately equal arms and V shape may appeare. 2. Submetacentric chromosomes : centromere is located near the middle. As a result, the arms are slightly unequal in length (one arm longer than the other) and may also form an L-shape. 3. Acrocentric chromosome: the centromere is located near the end of the chromosome, giving rise to one very long arm and one very short arm. 4. Telocentric chromosomes: the centromere is placed at the end of the chromosome, which presented only one arm. Karyotype A complete set of all the metaphase chromosomes in a cell of an individual is called the cell’s karyotype. Chromosomes are typically identified during metaphase, the stage of mitosis at which they are most condensed, which makes them easier to see under the microscope following staining. Karyotype also refers to a laboratory-produced image of a person’s chromosomes isolated from an individual cell and arranged in numerical order. A karyotype may be used to look for abnormalities in chromosome number or structure Karyotype for the cell of a normal human It is customary in karyotypes to arrange chromosomes in order according to their sizes and positions of their centromere. This karyotype shows 46 chromosomes: two pairs of each of the 22 autosomes and one of each of the X and Y sex chromosomes (which differ greatly in size). The resultant karyogram is a graphic depiction of the chromosomal complement, with each chromosome’s quantity and appearance representing the person’s genetic makeup. G banding This technique is used commonly in the generation of karyotypes in clinical analysis of human chromosomes. In G banding, chromosomes are treated with mild heat or proteolytic enzymes to digest the chromosomal proteins partially and then are stained with Giemsa stain to produce dark bands called G bands. In humans, approximately 300G bands can be distinguished in metaphase chromosomes, and approximately 2,000 G bands can be distinguished in chromosomes from the prophase stage of mitosis. Furthermore, a standard nomenclature based on the banding patterns has been established for the chromosomes so that scientists can talk about gene and marker locations with reference to specific regions and subregions. Each chromosome has two arms separated by the centromere. The smaller arm is designated p, and the larger arm is designated q. Numbered regions and numbered subregions are then assigned from the centromere outward; that is, region 1 is closest to the centromere. For example, the breast cancer susceptibility gene BRCA1 is at location 17q21, meaning that it is on the long arm of chromosome 17 in region 21. Subregions are indicated by decimal numerals after the region number. For instance, the cystic fibrosis gene spans subregions 7q31.2–q31.3; that is, it spans both subregions 2 and 3 of region 31 of the long arm of chromosome 7. A prokaryotic cell is a cell that lacks a nucleus and membrane-bound organelles. Most prokaryotes have a single circular chromosome (round in shape), and thus a single copy of their genetic material (single circular double stranded DNA), they can replicate faster than eukaryotic cells. Prokaryotes lack a membrane-bound nucleus to hold their chromosomes. As a substitute, the chromosome of a prokaryote is found in a part of the cytoplasm called a nucleoid. Nucleoid (meaning nucleus-like) is an irregularly-shaped region within the cell of a prokaryotes that contains most of the genetic material. Only one single chromosome is present in (genome of it is haploid). It is very compact in nature to fit inside the small cell by supercoiling and has less junk genetic material. The length of a genome varies widely, but generally at least a few million of base pairs. Addition to the chromosome, many prokaryotes contain small rings of double-stranded extra-chromosomal DNA called (outside the chromosome) plasmids. Plasmids carry a small number of non-essential genes and are copied independently of the chromosome inside the cell. They can be transferred to other prokaryotes in a population, sometimes spreading genes that are beneficial to survival. For instance, some plasmids carry genes that make bacteria resistant to antibiotics. (These genes are called R genes). When the plasmids carrying R genes are exchanged in a population, they can quickly make the population resistant to antibiotic drugs. While beneficial to the bacteria, this process can make it difficult for doctors to treat harmful bacterial infections. Difference between prokaryotic and eukaryotic chromosome Prokaryotes have double stranded mostly circular chromosome while the Eukaryotes have double stranded linear chromosomes which contain all the basic information. Prokaryotic cells usually have only one chromosome while the eukaryotic cells can have multiple chromosomes, and Prokaryotic chromosome is very simple compared to the eukaryotic one. Since prokaryotic cells have only one chromosome, it doesn't have homologous pair. On the other hand, eukaryotic chromosome are usually paired called homologous pair. These paired chromosomes are also called as sister chromosomes. Chromosomes in the prokaryotic cells are found in cytoplasm in a spot called as nucleoid since there is no specified organelle. But in case of eukaryotic cells, chromosomes are found in membrane bound organelle called nucleus. The packaging of chromosome in prokaryotic cell is rather simpler than eukaryotic cell. Prokaryotic chromosome is packed with the help of nucleoid associated proteins which helps in packaging. Eukaryotic chromosomes are much larger in size and requires special proteins called histone proteins which forms a special structure called nucleosomes when DNA is wrapped around it. Prokaryotic chromosomes encodes for fewer proteins as compared to larger eukaryotic chromosomes which codes for thousands of different proteins. Eukaryotic Chromosome Prokaryotic Chromosome Shape Linear Circular Size Large Small Number Multiple Single Location Nucleus Nucleoid (region in cytoplasm) Storage proteins Histones Nucleoid-associated proteins Origin of Fidelity of replication replication Requirements of Types of replication replication Steps of Direction of replication replication On chromosomes, plasmids, or viruses, initiation of replication begins at particular DNA sequence known as origins of replication (“origins”, OriC) where initiator proteins bind to DNA to start the process of replication. Two strands open forming Replication Forks (Y-shaped region). New strands grow at the fork. Origins are targeted by proteins that separate the two strands. initiate DNA synthesis. How the initiator proteins work? They bind to OriC and cause a short section of DNA to unwind initiator of replication forks forming a bubble. Two Y-shaped replication forks making a replication bubble. Prokaryotes Chromosome have a single bubble. Two replication forks Eukaryotes Chromosomes have many bubbles. Hundreds of replication fork. →This process (unwinding) allows helicase and other single-strand- binding proteins to attach to the polynucleotide strand. Origin tends to be (AT-rich) why? Because (A-T) bases form pairs of hydrogen bonds rather than (C-G). so, they want a little energy to be broken. Prokaryotes Eukaryotes 1 - Raw material Four types of nucleotides; Adenine (A), guanine (G), thymine (T) and cytosine (C). 2 - DNA template strands serves as a template for synthesis of a complementary DNA strand at any given time. 3-Replication apparatus DNA dependent DNA polymerase the main enzyme involved in catalyze the synthesis of DNA. Catalyzes formation of phosphodiester bond between 3’-OH of the deoxyribose (on the last nucleotide) and the 5’-phosphate of the dNTP. New nucleotides are joined in the direction of → 3` of the newly strand (5`→3`) by the effect of DNA polymerases enzymes (synthesis done at the direction of (5`→3`) of the newly strand). Because of the 2 DNA strands are anti parallel, and the elongation only in direction 5`→3`; if one template proceed from left to right, the other template proceed from right to left. As DNA unwinds can get 2 direction 1 - 3`→5` direction in template strand Allow new strand to synthesized continuously in 5`→3` direction of new strand called leading strand. 2 - 5`→3` direction in template strand After short length of DNA has unwound, synthesis must proceed in direction 5`→3` of new strand, which is opposite to the direction of unwinding, so the new strand synthesized discontinuously in 5`→3` called lagging strand. Lagging strand composed of short segment of DNA called okazaki fragments. Which are linked together to create a continuous new DNA molecule. Enzyme Function in DNA replication Known helix destabilizing enzyme. Unwinds the DNA double helix at the Replication Fork. Builds a new duplex DNA strand by adding nucleotides in the 5' to 3' direction. Also performs proof-reading and error correction. There exist many different types of DNA Polymerase, each of which perform different functions in different types of cells. RNA Polymerase Provides a starting RNA short primers for DNA polymerase to begin (Primase) synthesis of the new DNA strand. Protein complex that serves as a processivity-promoting factor in DNA replication. he clamp protein binds DNA polymerase and prevents this enzyme.from dissociating from the template DNA strand. Bind to ssDNA and prevent the DNA double helix from reannealing after Single-Strand DNA helicase unwinds it, thus maintaining the strand separation, and Binding(SSB) Proteins facilitating the synthesis of the nascent strand. Relaxes the DNA from its super-coiled nature. DNA Ligase Re-anneals and and joins Okazaki Fragments of the lagging strands. DNA dependent DNA polymerase DNA dependent RNA polymerase RNA (RdRp or RNA-replicase) dependent RNA polymerase RNA dependent DNA polymerase DNA Polymerase α is a repair polymerase, with 3’ to 5’ exonucleases activities. has polymerase activity 5’ to 3’. also acts as a primase to synthesis primer. DNA Polymerase β is a repair polymerase. DNA Polymerase γ shows polymerase activity 5’ to 3’. exonucleases activity 3’ to 5’. involved in Mitochondrial DNA replication. DNA Polymerase δ shows 3’ to 5’ exonuclease activity shows 5’ to 3’ polymerase activity. involved in lagging strand synthesis. DNA Polymerase ε shows 3’ to 5’ and 5’ to 3’ exonucleases activities. it not only repair but also synthesize the leading strand efficiently in a 5’ to 3’ direction. prime enzyme for DNA replication. DNA Polymerase Ι repair polymerase with both 3’ to 5’ and 5’ to 3’ exonuclease activity. polymerase activity in 5’ to 3’ direction. this enzyme is involved in the processing of Okazaki fragments generated by lagging strand synthesis. DNA Polymerase ΙΙ has 3’ to 5’ exonuclease activity that participates in DNA repair. show polymerase activity from 5’ to 3’ direction. involved in replication of damaged DNA DNA Polymerase ΙΙΙ the primary enzyme involved in DNA replication. has 5’ to 3’ polymerase activity. has 3’ to 5’ exonuclease activity. Strand Separation 1 - Helicase enzyme Catalyze the unwinding and separation (breaking H- Bonds) of the parental double helix. 2 - Single-Strand Binding Proteins Proteins which attach and help keep the separated strands apart. 3 - Topoisomerase enzyme Relieves stress on the DNA molecule by allowing free rotation around a single strand. 4 - Priming 1. RNA primers: before new DNA strands can form, there must be small preexisting primers (RNA) present to start the addition of new nucleotides (DNA Polymerase). Primase enzyme that polymerizes (synthesizes) the RNA Primer. Primase enzyme DNA dependent RNA polymerase DNA polymerase and Sliding clamp subunit Initiation Elongation Termination Replication begins when initiator proteins bind to OriC to start the process of replication. Hydrogen bonds break and helix opens (unwinding of the two DNA strands). Each strand of DNA acts as a template for synthesis of a new, complementary strand. DNA Polymerase: with a RNA primer in place, catalyze the synthesis of a new DNA strand in the 5’to 3’ direction by adding nucleotide complementary to nucleotide in template strand. As the strands are separated, the polymerase enzyme start synthesizing the complementary sequence in each of the strands. The parental strands will act as a template for newly synthesizing daughter strands. Elongation is unidirectional i.e. DNA is always polymerised only in the 5′ to 3′ direction. Therefore, one strand (the template 3‘→5‘) it is continuous (leading strand), while on the other strand (the template 5‘→3‘) it is discontinuous replication (lagging strand). Prokaryotic termination In circular bacterial chromosomes, Termination of DNA replication occurs when two oppositely orientated replication forks meet and fuse, to create two separate and Prokaryotic termination DNA molecules. complete double-stranded Eukaryotic termination DNA replication terminates when replisomes from adjacent replication origins converge. Termination involves local completion of DNA synthesis, decatenation of daughter molecules and replisome disassembly Completing the Lagging Strand After the replication fork has passed, the lagging strand is left as a series of short pieces with gap known as Okazaki fragments. These fragments joined together to give a complete strand of DNA by two enzymes working in succession: 1 - DNA polymerase I 2 - DNA ligase. DNA polymerase I fills in the gaps DNA ligase joins the ends. A common type of replication that takes place in circular DNA, such as that found in E. coli and other bacteria, is called theta replication, because it generates a structure that resembles the Greek letter theta (θ(. In theta replication, double-stranded DNA begins to unwind at the replication origin, producing single-stranded that serve as templates on which new DNA can be synthesized. The unwinding of the double helix generates a loop, termed a replication bubble. DNA replication on both of the template strands is simultaneous with unwinding, forming replication fork. Direction of Replication in Theta Model In the theta model, the DNA unwinds at one particular location, the origin, and a replication bubble is formed. If the bubble has two forks, one at each end, synthesis takes place simultaneously at both forks (bidirectional replication). At each fork, synthesis on one of the template strands proceeds in the same direction as that of unwinding; the newly replicated strand is the leading strand with continuous replication. On the other template strand, synthesis is proceeding in the direction opposite that of unwinding; this newly synthesized strand is the lagging strand with discontinuous replication. Notice that synthesis on this template strand is continuous at one fork but discontinuous at the other. Rolling circle replication takes place in some viruses and in the a small circle of extra chromosomal DNA that controls mating of E. coli. This form of replication is initiated by a break in one of the nucleotide strands that creates a 3'-OH group and a 5'-phosphate group. New nucleotides are added to the 3' end of the broken strand, with the inner (unbroken) strand used as a template. As new nucleotides are added to the 3' end, the 5' end of the broken strand is displaced from the template, rolling out like thread. The 3' end grows around the circle, giving rise to the name rolling-circle model. The replication fork may continue around the circle a number of times, producing several linked copies of the same sequence. With each revolution around the circle, the growing 3' end displaces the nucleotide strand synthesized in the preceding revolution. Eventually, the linear DNA molecule is cleaved from the circle, resulting in a double-stranded circular DNA molecule and a single- stranded linear DNA molecule. The linear molecule circularizes either before or after serving as a template for the synthesis of a complementary strand. Direction of Replication in Rolling-Circle Model Replication in the rolling-circle model is somewhat different, because there is no replication bubble. Replication begins at the 3' end of the broken nucleotide strand. Continuous replication takes place on the circular template as new nucleotides are added to this 3' end. The two strands of DNA unwind at the origin of replication. Helicase opens up the DNA-forming replication forks; these are extended bidirectionally. Single-strand binding proteins coat the DNA around the replication fork to prevent rewinding of the DNA. Topoisomerase binds at the region ahead of the replication fork to prevent supercoiling. RNA primers are synthesised by primase. These primers are complementary to the DNA strand. DNA polymerase starts adding nucleotides to the 3'-OH end of the primer. The leading and lagging strands continue to elongate. RNA primers are removed by exonuclease activity. Gaps are filled by DNA pol by adding dNTPs. The gap between the two DNA fragments is sealed by DNA ligase, which helps in the formation of phosphodiester bonds. Eukaryotic DNA Replication Prokaryotic DNA replication Occurs in eukaryotic cells. Occurs in a prokaryotic cell. This process takes place in the cell’s nucleus. This process takes place in the cell’s cytoplasm. DNA is linear and double-stranded. DNA is circular and double-stranded. There are multiple sites for the origin of There is a single site for the origin of replication per replication per DNA molecule. DNA molecule. Multiple replication forks are formed in a DNA Only two replication forks are formed in a DNA molecule. molecule. Okazaki fragments are short of around 100-200 Okazaki fragments are large, around 1000-2000 nucleotides in length nucleotides in length. It is a slow process with around 100 nucleotides It is very rapid with around 2000 nucleotides added added per second. per second. DNA polymerase involved in eukaryotic DNA DNA polymerase involved in prokaryotic DNA replication is DNA polymerases α, β, γ, δ and ε replication is DNA polymerase Ι, and ΙΙΙ. Initiation is carried out by DNA polymerase α DNA polymerase III carries out both initiation and while elongation by DNA polymerase δ and ε. elongation. RNA primer is removed by DNA polymerase β. RNA primer is removed by DNA polymerase I.