7-Protein Structure PDF
Document Details
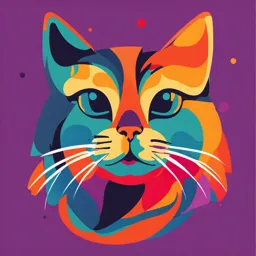
Uploaded by VersatileOnyx2535
Lokman Hekim Üniversitesi
Prof Dr Metin YILDIRIMKAYA
Tags
Summary
This document is lecture materials on protein structure. It covers different levels of protein structure (primary, secondary, tertiary, and quaternary), peptide bonds, post-translational modifications, protein denaturation, and the role of chaperones in protein folding. The document is aimed at an undergraduate level.
Full Transcript
BIOCHEMISTRY Lecture subject: Protein structure Dersin Sorumlusu: Prof Dr Metin YILDIRIMKAYA Online Lecture Materials Main issues Protein structures Primary structure and peptid bonds Secondary structure and cha...
BIOCHEMISTRY Lecture subject: Protein structure Dersin Sorumlusu: Prof Dr Metin YILDIRIMKAYA Online Lecture Materials Main issues Protein structures Primary structure and peptid bonds Secondary structure and characteristics Tertiary structure and stabilizing interactions Quaternary structure and characteristics Posttranslational modifications Protein denaturation Chaperones in protein folding Protein misfolding OVERVIEW Proteins are composed of amino acids that are joined together by peptide bonds in a linear sequence, and then folded into a unique three-dimensional shape that determines function. The protein structure is best analyzed by four organizational levels: primary, secondary, tertiary, and quaternary. Amino acids in proteins can be modified by phosphorylation, carboxylation, or other reactions after the protein is synthesized (posttranslational modifications). Alterations in the genetic code may lead to mutations in the protein’s primary structure, which can affect the protein’s function. Proteins with the same function but different primary structure (isoforms and isozymes) can exist in different tissues or during different phases of development. Protein structures Protein structures are made by condensation of amino acids forming peptide bonds. The sequence of amino acids in a protein is called its primary structure. The secondary structure is determined by the dihedral angles of the peptide bonds The tertiary structure is determined by the folding of protein chains in space. Association of folded polypeptide molecules to complex functional proteins results in quaternary structure. Protein structure is defined as a polymer of amino acids joined by peptide bonds PRIMARY STRUCTURE OF PROTEİNS The linear sequence of amino acids in a protein is the primary structure of the protein. Many genetic diseases result in proteins with abnormal amino acid sequences, causing improper folding and loss or impairment of normal function. If the primary structures of the normal and the mutated proteins are known, this information may be used to diagnose or study the disease. Peptide bond In proteins, adjacent amino acids are joined covalently by peptide bonds (amide linkages) Peptide bond occurs between the α-carboxyl group of one amino acid and the α-amino group of the next amino acid. For example, valine and alanine can form the dipeptide valylalanine through the formation of a peptide bond. Peptide bonds are resistant to conditions that denature proteins, such as heat and high concentrations of urea. Prolonged exposure to a strong acid or base at elevated temperatures is required to nonenzymatically break these bonds. Peptide bond characteristics: The peptide bond: has a partial double- bond character; that is, it is shorter than a single bond is rigid and planar; this prevents free rotation around the bond between the carbonyl carbon and the nitrogen of the peptide bond. is almost always in the trans configuration (instead of the cis), are uncharged, and neither accept nor release protons over the pH range of 2 to 12. -NH3+ -COO- Determining the amino acid composition of a polypeptide The first step in determining the primary structure of a polypeptide is to identify and measure its constituent amino acids. A purified sample of the polypeptide to be analyzed is first hydrolyzed by strong acid to cleave the peptide bonds, and release the individual amino acids. These can then be separated by cation-exchange chromatography. Sequencing the peptide from its N-terminal end Sequencing is a stepwise process of identifying the specific amino acid at each position in the peptide chain, beginning at the N-terminal end. Automated sequencers are used now; the historical process to produce amino acid derivatives is shown in Figure Determination of the amino (N)-terminal residue of a polypeptide by Edman degradation. PTH = phenylthiohydantoin. Phenyl-isothiocyanate: Edman reagent Cleaving the polypeptide into smaller fragments Many polypeptides have a primary structure composed of more than 100 amino acids. Such molecules cannot be sequenced directly from end to end. However, these large molecules can be cleaved at specific sites and the resulting fragments sequenced. Enzymes that hydrolyze peptide bonds are termed peptidases or proteases. (Note: Exopeptidases cut at the ends of proteins and are classified into aminopeptidases and carboxypeptidases. Carboxypeptidases are used in determining the C-terminal amino acid. Endopeptidases cleave within a protein.) SECONDARY STRUCTURE OF PROTEİNS The α-helix, β-sheet, and β-bend (or, β-turn) are examples of secondary structures commonly encountered in proteins. Each is stabilized by hydrogen bonds between atoms of the peptide backbone. Amino acids that disrupt an α-helix and β-sheet : Proline, glycine, For example: Keratins: rigid, fibrous proteins; α-helical; major component of hair and skin. Myoglobin: α-helical, globular, flexible molecule; found in muscles A: Structure of a β-sheet. B: An antiparallel β-sheet with the β-strands Structure of an α-helix. represented as broad arrows. C: A parallel β-sheet formed from a single polypeptide chain folding back on itself. Supersecondary structures (motifs) Supersecondary structures are intermediate between secondary and tertiary structures. These structures result from packing specific adjacent secondary structure motifs, such as helix-turn-helix, β-barrel, leucine zipper, and zinc zipper These form primarily the core (interior) region of the molecule. TERTIARY STRUCTURE This structure arises from further folding of the secondary structure of the protein. H-bonds, electrostatic forces, disulphide linkages, and Vander Waals forces stabilize this structure. The primary structure of a polypeptide chain determines its tertiary structure. The term tertiary refers both to the folding of domains (the basic units of structure and function;) and to the final arrangement of domains in the polypeptide. The tertiary structure of globular proteins in aqueous solution is compact, with a high density (close packing) of the atoms in the core of the molecule. Hydrophobic side chains are buried in the interior, whereas hydrophilic groups are generally found on the surface of the molecule. Schematic diagram of the primary, secondary, tertiary and quartenary structures of a protein Domains Domains are the fundamental functional and three-dimensional structural units of polypeptides. Polypeptide chains that are more than 200 amino acids in length generally consist of two or more domains. The core of a domain is built from combinations of supersecondary structural elements (motifs). Folding of the peptide chain within a domain usually occurs independently of folding in other domains. Therefore, each domain has the characteristics of a small, compact globular protein that is structurally independent of the other domains in the polypeptide chain. Stabilizing interactions The unique three-dimensional structure of each polypeptide is determined by its amino acid sequence. Interactions between the amino acid side chains guide the folding of the polypeptide to form a compact structure. The following five types of interactions cooperate in stabilizing the tertiary structures of globular proteins. 1. Disulfide bonds 2. Hydrophobic interactions 3. Hydrogen bonds 4. Ionic interactions 5. Vander Waals forces Interactions betvveen amino acid residues in a polypeptide chain: 1. electrostatic interactions, 2. hydrogen bonds, 3. hydrophobic interactions, and 4. a disulfide bond. Disulfide bonds: Formation of a disulfide bond by the oxidation of two cysteine residues, producing one cystine residue. A disulfide bond (–S–S–) is a covalent linkage formed from the sulfhydryl group (−SH) of each of two cysteine residues to produce a cystine residue. The two cysteines may be separated from each other by many amino acids in the primary sequence of a polypeptide or may even be located on two different polypeptides. The folding of the polypeptide(s) brings the cysteine residues into proximity and permits covalent bonding of their side chains. A disulfide bond contributes to the stability of the three- dimensional shape of the protein molecule and prevents it from denaturation Hydrophobic interactions: Amino acids with nonpolar side chains tend to be located in the interior of the polypeptide molecule, where they associate with other hydrophobic amino acids. In contrast, amino acids with polar or charged side chains tend to be located on the surface of the molecule in contact with the polar solvent. In each case, a segregation of R groups occurs that is energetically most favorable. Hydrogen bonds: Amino acid side chains containing oxygen- or nitrogen-bound hydrogen, such as in the alcohol groups of serine and threonine, can form hydrogen bonds with electron-rich atoms, such as the oxygen of a carboxyl group or carbonyl group of a peptide bond. Formation of hydrogen bonds between polar groups on the surface of proteins and the aqueous solvent enhances the solubility of the protein. Ionic interactions: Negatively charged groups, such as the carboxylate group (−COO−) in the side chain of aspartate or glutamate, can interact with positively charged groups such as the amino group (−NH3+) in the side chain of lysine Van der Waals forces are weak intermolecular attractions between atoms or molecules, arising from temporary or permanent dipoles. Van der Waals forces are the weakest intermolecular forces Steps in protein folding (simplified) Interactions between the side chains of amino acids determine how a linear polypeptide chain folds into the three-dimensional shape of the functional protein. Protein folding, which occurs within the cell in seconds to minutes, involves nonrandom, ordered pathways. As a peptide folds, secondary structures form, driven by the hydrophobic effect; hydrophobic groups come together as water is released. These small structures combine to form larger structures. Additional events stabilize secondary structure and initiate formation of tertiary structure. In the last stage, the peptide achieves its fully folded, native (functional) form characterized by a low-energy state. Protein denaturation Denaturation results in the unfolding and disorganization of a protein’s secondary and tertiary structures without the hydrolysis of peptide bonds. Denaturing agents heat, urea, organic solvents, strong acids or bases, detergents, and ions of heavy metals such as lead. Denaturation may, under ideal conditions, be reversible, such that the protein refolds into its original native structure when the denaturing agent is removed. However, most proteins remain permanently disordered once denatured. Denatured proteins are often insoluble and precipitate from solution. Select the true statements about denaturation A. Denaturation of an enzyme will cause a loss of its catalytic activity B. Protein denaturation involves cleavage of its peptide bonds C. Denaturation and digestion refer to the same process D. A compeptitive enzyme inhibitor acts by denaturing the enzyme E. A denatured protein has a different tertiary structure than its native state Chaperones in protein folding The information needed for correct protein folding is contained in the primary structure of the polypeptide. However, most denatured proteins do not resume their native conformations even under favorable environmental conditions. This is because, for many proteins, folding is a facilitated process that requires ATP hydrolysis and a specialized group of proteins, referred to as molecular chaperones. The chaperones, also known as heat-shock proteins (HSPs), interact with a polypeptide at various stages during the folding process. Some chaperones bind hydrophobic regions of an extended polypeptide and are important in keeping the protein unfolded until its synthesis is completed (e.g., Hsp70). Chaperone proteins participate in the folding of over half of all mammalian proteins. Calnexin is a chaperone protein in the endoplasmic reticulum membrane; binding to calnexin prevents a glycoprotein from aggregating. The soluble endoplasmic reticulum protein calreticulin performs a similar function to that of calnexin. QUATERNARY STRUCTURE Two or more polypeptide chains that may be structurally identical or totally unrelated. The arrangement of these polypeptide subunits is the quaternary structure of the protein. Subunits are held together primarily by noncovalent interactions including hydrogen bonds, ionic bonds, and hydrophobic interactions. Subunits may function independently of each other or may work cooperatively, as in hemoglobin the binding of oxygen to one subunit of the tetramer increases the affinity of the other subunits for oxygen Summary of Protein Structure The primary structure of protein is the hierarchy’s basic level, and is the particular linear sequence of amino acids comprising one polypeptide chain. Secondary structure is the next level up from the primary structure, and is the regular folding of regions into specific structural patterns within one polypeptide chain. Hydrogen bonds between the carbonyl oxygen and the peptide bond amide hydrogen are normally held together by secondary structures. Tertiary structure is the next level up from the secondary structure, and is the particular three- dimensional arrangement of all the amino acids in a single polypeptide chain. This structure is usually conformational, native, and active, and is held together by multiple noncovalent interactions. Quaternary structure is the next ‘step up’ between two or more polypeptide chains from the tertiary structure and is the specific spatial arrangement and interactions. Posttranslational modifications (PTM) of proteins PTM is the covalent process of changing proteins following protein biosynthesis PTMs may involve enzymes or occur spontaneously. PTMs are important components in cell signalling, as for example when prohormones are converted to hormones Post-translational modifications can occur on the amino acid side chains or at the protein's C- or N- termini. They can expand the chemical set of the 20 amino acids by changing an existing functional group or adding a new one such as phosphate. Phosphorylation is highly effective for controlling the enzyme activity and is the most common change after translation. Many proteins also have carbohydrate molecules attached to them in a process called glycosylation, which can promote protein folding and improve stability as well as serving regulatory functions. Attachment of lipid molecules, known as lipidation, often targets a protein or part of a protein attached to the cell membrane Some types of post-translational modification are consequences of oxidative stress Types of post-translational modification Based on the addition of chemical groups Phosphorylation Acetylation Hydroxylation Methylation Based on the addition of complex groups Glycosylation AMPylation Lipidation Based on the addition of polypeptides Ubiquitination Based on the cleavage of proteins Proteolysis Based on the amino acid modification Deamidation Common types of protein posttranslational modifications PROTEIN MISFOLDING Protein folding is a complex process that can sometimes result in improperly folded molecules. These misfolded proteins are usually tagged and degraded within the cell. However, this quality control system is not perfect, and intracellular or extracellular aggregates of misfolded proteins can accumulate, particularly as individuals age. Deposits of misfolded proteins are associated with a number of diseases: Amyloid diseases Prion diseases A. Amyloid diseases Misfolding of proteins may occur spontaneously or be caused by a mutation in a particular gene, which then produces an altered protein. In addition, some apparently normal proteins can, after abnormal proteolytic cleavage, take on a unique conformation that leads to the spontaneous formation of long, fibrillar protein assemblies consisting of β- pleated sheets. Accumulation of these insoluble fibrous protein aggregates, called amyloids, has been implicated in neurodegenerative disorders such as Alzheimer disease (AD) and Parkinson disease. AlzheimerB s disease is a debilitating condition characterized by loss of memory, disorientation, and accumulation in the brain of tangles of protein called: A. Amyloid deposits B. Calcification C. Atherosclerosis D. Hydroxyapatite E. Protein bundling B. Prion diseases A prion is a type of protein that can trigger normal proteins in the brain to fold abnormally. Prion diseases can affect both humans and animals and are sometimes spread to humans by infected meat products. The most common form of prion disease that affects humans is Creutzfeldt-Jakob disease (CJD); scrapie in sheep, and bovine spongiform encephalopathy, popularly called “mad cow disease,” in cattle. It is highly resistant to proteolytic degradation and tends to form insoluble aggregates of fibrils, similar to the amyloid found in some other diseases of the brain. Prion diseases occur when normal prion protein, found on the surface of many cells, becomes abnormal and clump in the brain, causing brain damage. This abnormal accumulation of protein in the brain can cause memory impairment, personality changes, and difficulties with movement. Unknown etiology; rare; Fatal (generally) https://www.brightfocus.org/alzheimers/article/tau-protein- and-alzheimers-disease-whats-connection When considering protein structure: A. Proteins with one polypeptide have quaternary structure stabilized by covalent bonds. B. Peptide bonds that link amino acids most commonly occur in the cis configuration. C. Disulfide bonds in proteins are between cysteine residues adjacent in the primary structure. D. Denaturation of proteins leads to irreversible loss of secondary structural elements. E. The primary driving force for protein folding is the hydrophobic effect. Correct answer = E. E. The hydrophobic effect, or the tendency of nonpolar entities to associate in a polar environment, is the primary driving force of protein folding. A. Quaternary structure requires more than one polypeptide, and, when present, it is stabilized primarily by noncovalent bonds. B. The peptide bond is almost always trans. C. The two cysteine residues participating in disulfide bond formation may be a great distance apart in the amino acid sequence of a polypeptide (or on two separate polypeptides) but are brought into close proximity by the three-dimensional folding of the polypeptide D. Denaturation may be reversible or irreversible. A particular point mutation results in disruption of the α- helical structure in a segment of the mutant protein. The most likely change in the primary structure of the mutant protein is: A. Glutamate to aspartate. B. Lysine to arginine. C. Methionine to proline. D. Valine to alanine. Correct answer = C. C. Proline, because of its secondary amino group, is incompatible with an α-helix. Glutamate, aspartate, lysine, and arginine are charged amino acids, and valine is a branched amino acid. Charged and branched (bulky) amino acids may disrupt an α-helix. The flexibility of glycine’s R group (a H) can also disrupt an α-helix. Which statement is true of β-sheets only, and not α-helices? A. They may be found in typical globular proteins. B. They are stabilized by interchain hydrogen bonds. C. They are examples of secondary structure. D. They may be found in supersecondary structures. Correct answer = B. B. The β-sheet is stabilized by interchain hydrogen bonds formed between separate polypeptide chains, and by intrachain hydrogen bonds formed between regions of a single polypeptide. The α-helix, however, is stabilized only by intrachain hydrogen bonds. Statements A, C, and D are true for both of these secondary structural elements. When compared to fatty acids, nucleic acids, and carbohydrates, proteins are found to contain more: A. Carbon B. Hydrogen C. Oxygen D. Phosphorus E. Nitrogen Asp-Ala-Ser-Glu-Val-Arg At physiologic pH (7.4), this hexapeptide will contain a net charge of: A. -2 B. -1 C. 0 D. +1 E. +2 In a polypeptide at physiological pH, hydrogen bonding may occur between which one of the following? A. The side chains of a leucine residue and a lysine residue B. The side chains of an aspartyl residue and a glutamyl residue C. The amide group in the peptide bond and an aspartyl side chain D. The terminal α-amino group and the terminal α- carboxyl group E. The SH groups of two cysteine residues Links https://www.khanacademy.org/science/biology/macromolecules/pro teins-and-amino-acids/v/peptide-bond-formation https://www.khanacademy.org/science/biology/macromolecules/pro teins-and-amino-acids/v/overview-of-protein-structure https://www.khanacademy.org/science/biology/macromolecules/pro teins-and-amino-acids/v/tertiary-structure-of-proteins THANK YOU FOR LISTENING Online Lecture Materials