Heart Muscle & Signal Transduction PDF
Document Details
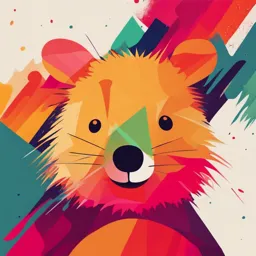
Uploaded by InstructiveTheme
BAU Medical School
Mehmet Ozansoy, Ph.D.
Tags
Summary
This document provides a comprehensive overview of heart muscle and the signal transduction processes within the heart. It discusses the characteristics of cardiac myocytes, their unique features compared to skeletal muscle, and the mechanisms regulating heart contraction. The document explores the various factors influencing the entry and efflux of calcium, including the roles of different ion channels and protein kinases. It also delves into the metabolic processes and energy requirements of cardiomyocytes.
Full Transcript
HEART MUSCLE & SIGNAL TRANSDUCTION IN THE HEART MEHMET OZANSOY, Ph.D. Dept. of Physiology CARDIAC MUSCLE Cardiac myocytes represent a type of striated muscle, so- called because crossbands or cross striations are observed microscopically. Although cardiac muscle shares some structural and f...
HEART MUSCLE & SIGNAL TRANSDUCTION IN THE HEART MEHMET OZANSOY, Ph.D. Dept. of Physiology CARDIAC MUSCLE Cardiac myocytes represent a type of striated muscle, so- called because crossbands or cross striations are observed microscopically. Although cardiac muscle shares some structural and functional similarities with skeletal muscle, it has several important differences Cardiac myocytes form a branching network of cells that is sometimes referred to as a functional syncytium, which results from a fusion of cells. Individual myocytes connect to each other by way of specialized cell membranes called intercalated disks Gap junctions within these intercellular regions serve as low resistance pathways between cells, permitting cell-to-cell conduction of electrical (ionic) currents. If one cardiac myocyte is electrically stimulated, cellto-cell conduction ensures that the electrical impulse will travel to all of the interconnected myocytes. Regulation of Contraction (Inotropy) Calcium entry into the cell through L-type calcium channels Calcium release by the sarcoplasmic reticulum Calcium binding to TN-C Myosin phosphorylation SERCA activity Calcium efflux across the sarcolemma Calcium Entry into Myocytes The amount of calcium that enters the cell during depolarization is regulated largely by phosphorylation of the L-type calcium channel. The primary mechanism for this regulation involves cyclic adenosine monophosphate (cAMP), the formation of which is coupled to β-adrenoceptors. Norepinephrine released by sympathetic nerves, or circulating epinephrine released by the adrenal glands, binds primarily to β1-adrenoceptors located on the sarcolemma This receptor is coupled to a specific guanine nucleotide- binding regulatory protein (stimulatory G-protein; Gsprotein) That activates adenylyl cyclase, which in turn hydrolyzes ATP to cAMP. The cAMP acts as a second messenger to activate protein kinase A (cAMP-dependent protein kinase, PKA), which is capable of phosphorylating different sites within the cell One important site of phosphorylation is the L-type calcium channel. Phosphorylation increases the permeability of the channel to calcium, thereby increasing calcium influx during action potentials. This increase in calcium enhances calcium release by the sarcoplasmic reticulum, thereby increasing inotropy Therefore, norepinephrine and epinephrine are positive inotropic agents. Another G-protein, the inhibitory G protein (Gi-protein), inhibits adenylyl cyclase and decreases intracellular cAMP. Therefore, activation of this pathway decreases inotropy. This pathway is coupled to muscarinic receptors (M2) that bind acetylcholine released by parasympathetic (vagal) nerves within the heart. Adenosine receptors (A1) also are coupled to the Gi-protein. Therefore, acetylcholine and adenosine are negative inotropic agents. Calcium Uptake by Sarcoplasmic Reticulum PKA phosphorylation of phospholamban, which removes the inhibitory effect of phospholamban on SERCA, increases the rate of calcium transport into the sarcoplasmic reticulum. SERCA activity can also be stimulated by increased intracellular calcium caused by increased calcium entry into the cell or decreased cellular efflux. Regulation of Calcium Efflux from the Myocyte The final mechanisms that can modulate inotropy are the sarcolemmal Na+/Ca++ exchange pump and the ATPdependent calcium pump. These pumps transport calcium out of the cell, thereby preventing the cell from becoming overloaded with calcium. If calcium extrusion is inhibited, the rise in intracellular calcium can increase inotropy because more calcium is available to TN-C Digitalis and related cardiac glycosides inhibit the Na+/K+- ATPase, which increases intracellular sodium. This leads to an increase in intracellular Ca++ through the Na+/Ca++ exchange pump, leading to enhanced inotropy Cardiomyocyte Metabolism Cardiac myocytes have an exceptionally high metabolic rate because their primary function is to contract repetitively. Unlike skeletal muscle, in which contraction is often intermittent and relatively short, cardiac muscle contracts one to three times per second throughout life Repetitive cycles of contraction and relaxation require an enormous amount of ATP, which the heart must produce aerobically. This is why cardiac myocytes contain such large numbers of mitochondria. In the absence of oxygen, myocytes can contract for no more than a minute. Unlike some types of skeletal muscle fibers (e.g., fast twitch, glycolytic), cardiac myocytes have only a limited anaerobic capacity for meeting ATP requirements. Unlike many other cells in the body, cardiac myocytes can use a variety of substrates to regenerate ATP oxidatively. For example, in an overnight fasted state, the heart uses primarily fatty acids (~60%) and carbohydrates (~40%). Following a high-carbohydrate meal, the heart can adapt to using carbohydrates (primarily glucose) almost exclusively. Lactate can be used in place of glucose, and it becomes an important substrate during exercise when circulating concentrations of lactate increase. The heart also can use amino acids and ketones (e.g., acetoacetate) instead of fatty acids. CARDIAC CONDUCTION SYSTEM Generating rhythmical electrical impulses to cause rhythmical contraction of the heart muscle Conducting these impulses rapidly through the heart The atria contract about one sixth of a second ahead of ventricular contraction, allowing filling of the ventricles before they pump the blood through the lungs and peripheral circulation. All portions of the ventricles to contract almost simultaneously, which is essential for most effective pressure generation in the ventricular chambers. Sinus (Sinoatrial) Node A small, flattened, ellipsoid strip of specialized cardiac muscle about 3 millimeters wide, 15 millimeters long, and 1 millimeter thick. Located in the superior posterolateral wall of the right atrium immediately below and slightly lateral to the opening of the superior vena cava. The fibers of this node have almost no contractile muscle filaments The sinus nodal fibers connect directly with the atrial muscle fibers, so that any action potential that begins in the sinus node spreads immediately into the atrial muscle wall. Automatic Electrical Rhythmicity of the Sinus Fibers The “resting membrane potential” of the sinus nodal fiber between discharges has a negativity of about -55 to -60 millivolts, in comparison with -85 to -90 millivolts for the ventricular muscle fiber. The cause of this lesser negativity: The cell membranes of the sinus fibers are naturally leaky to sodium and calcium ions, and positive charges of the entering sodium and calcium ions neutralize much of the intracellular negativity Cardiac muscle has three types of membrane ion channels that play important roles in causing the voltage changes of the action potential. They are fast sodium channels, slow sodium-calcium channels, and potassium channels. Opening of the fast sodium channels for a few 10,000ths of a second is responsible for the rapid upstroke spike of the action potential observed in ventricular muscle, because of rapid influx of positive sodium ions to the interior of the fiber. Then the “plateau” of the ventricular action potential is caused primarily by slower opening of the slow sodium-calcium channels, which lasts for about 0.3 second. Finally, opening of potassium channels allows diffusion of large amounts of positive potassium ions in the outward direction through the fiber membrane and returns the membrane potential to its resting level The “resting” potential of sinus nodal fibers is much less negative—only -55 millivolts in the nodal fiber instead of the -90 millivolts in the ventricular muscle fiber. Only the slow sodium-calcium channels can open (i.e., can become “activated”) and thereby cause the action potential. As a result, the atrial nodal action potential is slower to develop than the action potential of the ventricular muscle. Also, after the action potential does occur, return of the potential to its negative state occurs slowly as well, rather than the abrupt return that occurs for the ventricular fiber. Self-Excitation of Sinus Nodal Fibers Because of the high sodium ion concentration in the extracellular fluid outside the nodal fiber, as well as a moderate number of already open sodium channels, positive sodium ions from outside the fibers normally tend to leak to the inside. Therefore, between heartbeats, influx of positively charged sodium ions causes a slow rise in the resting membrane potential in the positive direction The “resting” potential gradually rises between each two heartbeats. When the potential reaches a threshold voltage of about -40 millivolts, the sodium-calcium channels become “activated,” thus causing the action potential. Therefore, basically, the inherent leakiness of the sinus nodal fibers to sodium and calcium ions causes their self-excitation Why does this leakiness to sodium and calcium ions not cause the sinus nodal fibers to remain depolarized all the time? First, the sodium-calcium channels become inactivated (i.e., they close) within about 100 to 150 milliseconds after opening. Second, at about the same time, greatly increased numbers of potassium channels open Therefore, influx of positive calcium and sodium ions through the sodium-calcium channels ceases, while at the same time large quantities of positive potassium ions diffuse out of the fiber. Both of these effects reduce the intracellular potential back to its negative resting level and therefore terminate the action potential Furthermore, the potassium channels remain open for another few tenths of a second, temporarily continuing movement of positive charges out of the cell, with resultant excess negativity inside the fiber; This is called hyperpolarization Why this new state of hyperpolarization is not maintained forever? During the next few tenths of a second after the action potential is over, progressively more and more potassium channels close. The inward-leaking sodium and calcium ions once again overbalance the outward flux of potassium ions, and this causes the “resting” potential to drift upward once more, finally reaching the threshold level for discharge at a potential of about -40 millivolts. Then the entire process begins again Internodal Pathways The ends of the sinus nodal fibers connect directly with surrounding atrial muscle fibers. Therefore, action potentials originating in the sinus node travel outward into these atrial muscle fibers. In this way, the action potential spreads through the entire atrial muscle mass and, eventually, to the A-V node. The velocity of conduction in most atrial muscle is about 0.3 m/sec, but conduction is more rapid, about 1 m/sec, in several small bands of atrial fibers. One of these, called the anterior interatrial band, passes through the anterior walls of the atria to the left atrium. In addition, three other small bands curve through the anterior, lateral, and posterior atrial walls and terminate in the A-V node Atrioventricular Node The atrial conductive system is organized so that the cardiac impulse does not travel from the atria into the ventricles too rapidly; This delay allows time for the atria to empty their blood into the ventricles before ventricular contraction begins. It is primarily the A-V node and its adjacent conductive fibers that delay this transmission into the ventricles The A-V node is located in the posterior wall of the right atrium immediately behind the tricuspid valve The impulse, after traveling through the internodal pathways, reaches the A-V node about 0.03 second after its origin in the sinus node. Then there is a delay of another 0.09 second in the A-V node itself before the impulse enters the penetrating portion of the A-V bundle, where it passes into the ventricles. A final delay of another 0.04 second occurs mainly in this penetrating A-V bundle, which is composed of multiple small fascicles passing through the fibrous tissue separating the atria from the ventricles The total delay in the A-V nodal and A-V bundle system is about 0.13 second. This, in addition to the initial conduction delay of 0.03 second from the sinus node to the A-V node, makes a total delay of 0.16 second before the excitatory signal finally reaches the contracting muscle of the ventricles The slow conduction in the transitional, nodal, and penetrating A-V bundle fibers is caused mainly by diminished numbers of gap junctions between successive cells in the conducting pathways, so that there is great resistance to conduction of excitatory ions from one conducting fiber to the next Rapid Transmission in the Ventricular Purkinje System Special Purkinje fibers lead from the A-V node through the A-V bundle into the ventricles. Except for the initial portion of these fibers where they penetrate the A- V fibrous barrier, They have functional characteristics that are quite the opposite of those of the A-V nodal fibers. They are very large fibers, even larger than the normal ventricular muscle fibers, and they transmit action potentials at a velocity of 1.5 to 4.0 m/sec, a velocity about 6 times that in the usual ventricular muscle and 150 times that in some of the A-V nodal fibers This allows almost instantaneous transmission of the cardiac impulse throughout the entire remainder of the ventricular muscle The rapid transmission of action potentials by Purkinje fibers is to be caused by a very high level of permeability of the gap junctions at the intercalated discs between the successive cells that make up the Purkinje fibers. Therefore, ions are transmitted easily from one cell to the next, thus enhancing the velocity of transmission. The Purkinje fibers also have very few myofibrils, which means that they contract little or not at all during the course of impulse transmission The impulse spreads at moderate velocity through the atria but is delayed more than 0.1 second in the A-V nodal region before appearing in the ventricular septal A-V bundle. Once it has entered this bundle, it spreads very rapidly through the Purkinje fibers to the entire endocardial surfaces of the ventricles. Then the impulse once again spreads slightly less rapidly through the ventricular muscle to the epicardial surfaces The Sinus Node as the Pacemaker of the Heart The impulse normally arises in the sinus node. A few other parts of the heart can exhibit intrinsic rhythmical excitation in the same way that the sinus nodal fibers do; This is particularly true of the A-V nodal and Purkinje fibers The A-V nodal fibers, when not stimulated from some outside source, discharge at an intrinsic rhythmical rate of 40 to 60 times per minute The Purkinje fibers discharge at a rate somewhere between 15 and 40 times per minute. These rates are in contrast to the normal rate of the sinus node of 70 to 80 times per minute. The discharge rate of the sinus node is considerably faster than the natural self-excitatory discharge rate of either the AV node or the Purkinje fibers. Each time the sinus node discharges, its impulse is conducted into both the A-V node and the Purkinje fibers, also discharging their excitable membranes. But the sinus node discharges again before either the A-V node or the Purkinje fibers can reach their own thresholds for self-excitation Thus, the sinus node controls the beat of the heart because its rate of rhythmical discharge is faster than that of any other part of the heart. Therefore, the sinus node is virtually always the pacemaker of the normal heart