Introduction to Autonomic Pharmacology Chapter 6 PDF
Document Details
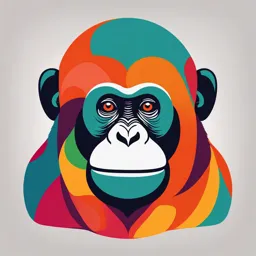
Uploaded by EruditeHydrogen
Zarqa University
2010
Dr. Haneen Basheer Dr. Shouroq Ibraheem
Tags
Summary
This document is a chapter on Introduction to Autonomic Pharmacology, discussing the autonomic nervous system (ANS), its anatomy, neurotransmitters, receptor characteristics, and functional integration. The chapter also covers the anatomy and neurotransmitter chemistry of the ANS, providing an introduction to autonomic pharmacology.
Full Transcript
Zarqa University Pharmacy school Clinical Pharmacy Department Pharmacology I Introduction to Autonomic Pharmacology Chapter 6 Dr. Haneen Basheer Dr. Shouroq Ibraheem © The McGraw-Hill Compa...
Zarqa University Pharmacy school Clinical Pharmacy Department Pharmacology I Introduction to Autonomic Pharmacology Chapter 6 Dr. Haneen Basheer Dr. Shouroq Ibraheem © The McGraw-Hill Companies, Inc., 2010 Any nerves that enter Brain and spinal cord or leave the CNS The neurons that carry The neurons that bring the signals away from the information from the CNS to the peripheral periphery to CNS tissues Are involved in the Are involved in the voluntary involuntary, unconscious control of functions control of functions Is a collection of nerve fibers that innervate the GIT, pancreas and gallbladder. Figure 3.1 (still) Chapter 3 MENU > Copyright © 2012 Wolters Kluwer Health│Lippincott Williams & Wilkins Introduction to Autonomic Pharmacology The autonomic nervous system (ANS) is the major involuntary, unconscious, automatic portion of the nervous system and contrasts in several ways with the somatic (voluntary) nervous system. The anatomy, neurotransmitter chemistry, receptor characteristics, and functional integration of the ANS are discussed in this chapter. Drugs in many other groups have significant autonomic effects, many of which are undesirable. © The McGraw-Hill Companies, Inc., 2010 Efferent neurons of ANS Figure 3.2 (still) Chapter 3 MENU > Copyright © 2012 Wolters Kluwer Health│Lippincott Williams & Wilkins Figure 4.2 (still) Chapter 4 MENU > Copyright © 2012 Wolters Kluwer Health│Lippincott Williams & Wilkins Figure 6–1. © The McGraw-Hill Companies, Inc., 2010 Figure 3.6 (still) Chapter 3 MENU > Copyright © 2012 Wolters Kluwer Health│Lippincott Williams & Wilkins ANATOMIC ASPECTS OF THE ANS The motor (efferent) portion of the ANS is the major pathway for information transmission from the central nervous system (CNS) to the involuntary effector tissues (smooth muscle, cardiac muscle, and exocrine glands). Its 2 major subdivisions are the parasympathetic ANS (PANS) and the sympathetic ANS (SANS). The enteric nervous system (ENS) is a semiautonomous part of the ANS located in the gastrointestinal tract, with specific functions for the control of this organ system. The ENS consists of the myenteric plexus (plexus of Auerbach) and the submucous plexus (plexus of Meissner); they send sensory input to the parasympathetic and sympathetic nervous systems and receive motor output from them. There are many sensory (afferent) fibers in autonomic nerves. These are of considerable importance for the physiologic control of the involuntary organs but are directly influenced by only a few drugs. © The McGraw-Hill Companies, Inc., 2010 Figure 6–2. AC, absorptive cell; CM, circular muscle layer; EC, enterochromaffin cell; EN, excitatory neuron; EPAN, extrinsic primary afferent neuron; 5HT, serotonin; IN, inhibitory neuron; IPAN, intrinsic primary afferent neuron; LM, longitudinal muscle layer; MP, myenteric plexus; NE, norepinephrine; NP, neuropeptides; SC, secretory cell; SMP, submucosal plexus. © The McGraw-Hill Companies, Inc., 2010 ANATOMIC ASPECTS OF THE ANS A. Central Roots of Origin The sympathetic preganglionic fibers originate in the thoracic (T1- T12) and lumbar (L1- L5) segments of the cord The parasympathetic preganglionic motor fibers originate in cranial nerve nuclei III, VII, IX, and X and in sacral segments (usually S2-S4) of the spinal cord. B. Location of Ganglia Most of the sympathetic ganglia are located in 2 paravertebral chains that lie along the spinal column. A few (the prevertebral ganglia) are located on the anterior aspect of the abdominal aorta. Most of the parasympathetic ganglia are located in the organs innervated more distant from the spinal cord. Because of the locations of the ganglia, the preganglionic sympathetic fibers are short and the postganglionic fibers are long. The opposite is true for the parasympathetic system: preganglionic fi bers are longer and postganglionic fibers are short. © The McGraw-Hill Companies, Inc., 2010 ANATOMIC ASPECTS OF THE ANS C. Un-innervated Receptors Some receptors that respond to autonomic transmitters and drugs receive no innervation. These include muscarinic receptors on the endothelium of blood vessels, some presynaptic receptors, and, in some species, the adrenoceptors on apocrine sweat glands and α2 and β adrenoceptors in blood vessels. © The McGraw-Hill Companies, Inc., 2010 EFFECTS OF ACTIVATING AUTONOMIC NERVES Each division of the ANS has specific effects on organ systems. Dually innervated organs such as the iris of the eye and the sinoatrial node of the heart receive both sympathetic and parasympathetic innervation. The pupil has a natural, intrinsic diameter to which it returns when the influence of both divisions of the ANS is removed. Pharmacologic ganglionic blockade, therefore, causes it to move to its intrinsic size. Similarly, the cardiac sinus node pacemaker rate has an intrinsic value (about 100-110/min) in the absence of both ANS inputs. How will these variables change (increase or decrease) if the ganglia are blocked? The answer is predictable if one knows which system is dominant. For example, both the pupil and, at rest, the sinoatrial node are dominated by the parasympathetic system. The resting pupil diameter and sinus rate are therefore under considerable PANS influence. Thus, blockade of both systems, with removal of the dominant PANS and nondominant SANS effects, result in mydriasis and tachycardia. © The McGraw-Hill Companies, Inc., 2010 Table 6-3. Should be MEMORIZED!! © The McGraw-Hill Companies, Inc., 2010 Table 6-3. © The McGraw-Hill Companies, Inc., 2010 How to memorize the table! Rationalize the sympathetic response to the fight-or-flight response. Pupil… dilate Heart… rate and contractility Bronchioles… dilate GI tract… shut down (the walls relax and sphincters contract) Bladder… shut down (the walls relax and sphincters contract) Blood… shunted from GI tract & skin to the muscles Metabolism… the supply of glucose FROM basic concepts in pharmacology, what you need to know for each drug class by Janet L. Stringer, 4th edition How to memorize the table! Effect on eye…contraction of: Radial muscle causes dilation (mydriasis)… sympathetic response Circular muscle causes constriction (miosis) (i.e. no d’s here)… parasympathetic response FROM basic concepts in pharmacology, what you need to know for each drug class by Janet L. Stringer, 4th edition Figure 3.4 (still) Chapter 3 MENU > Copyright © 2012 Wolters Kluwer Health│Lippincott Williams & Wilkins Figure 3.3 (still) Chapter 3 MENU > Copyright © 2012 Wolters Kluwer Health│Lippincott Williams & Wilkins © The McGraw-Hill Companies, Inc., 2010 © The McGraw-Hill Companies, Inc., 2010 C. Cotransmitters Many (probably all) autonomic nerves have transmitter vesicles that contain other transmitter molecules in addition to the primary agents (acetylcholine or norepinephrine) previously described. These co transmitters may be localized in the same vesicles as the primary transmitter or in a separate population of vesicles. Substances recognized to date as cotransmitters include ATP (adenosine triphosphate), enkephalins, vasoactive intestinal peptide, neuropeptide Y, substance P, neurotensin, somatostatin, and others. Their main role in autonomic function appears to involve modulation of synaptic transmission. The same substances function as primary transmitters in other synapses. © The McGraw-Hill Companies, Inc., 2010 Table 6-1. © The McGraw-Hill Companies, Inc., 2010 RECEPTOR CHARACTERISTICS A. Cholinoceptors Also referred to as cholinergic receptors, these molecules respond to acetylcholine and its analogs. Cholinoceptors are subdivided as follows: 1. Muscarinic receptors-As their name suggests, these receptors respond to muscarine (an alkaloid) as well as to acetylcholine. The effects of activation of these receptors resemble those of postganglionic parasympathetic nerve stimulation. Muscarinic receptors are located primarily on autonomic effector cells (including heart, vascular endothelium, smooth muscle, presynaptic nerve terminals, and exocrine glands). Evidence (including their genes) has been found for 5 subtypes, of which 3 appear to be important in peripheral autonomic transmission. All 5 are G-protein- coupled receptors. 2. Nicotinic receptors-These receptors are located on ion channels and respond to acetylcholine and nicotine, another acetylcholine mimic (but not to muscarine) by opening the channel. The 2 major nicotinic subtypes are located in ganglia and in skeletal muscle end plates. The nicotinic receptors are the primary receptors for transmission at these sites. © The McGraw-Hill Companies, Inc., 2010 Cholinoceptors in ANS M1, M3, M5 Gq coupled Muscarinic receptors M2, M4 Gi coupled Na+-K+ ion Nn Nicotinic channel receptors Na+-K+ ion Nm channel © The McGraw-Hill Companies, Inc., 2010 RECEPTOR CHARACTERISTICS B. Adrenoceptors Also referred to as adrenergic receptors, adrenoceptors are divided into several subtypes. 1. Alpha receptors- These are located on vascular smooth muscle, presynaptic nerve terminals, blood platelets, fat cells (lipocytes), and neurons in the brain. Alpha receptors are further divided into 2 major types, α1 and α2. These 2 subtypes constitute different families and use different G-coupling proteins. 2. Beta receptors- These receptors are located on most types of smooth muscle, cardiac muscle, some presynaptic nerve terminals, and lipocytes as well as in the brain. Beta receptors are divided into 3 major subtypes, β1, β2, and β3. These subtypes are rather similar and use the same G-coupling protein.. © The McGraw-Hill Companies, Inc., 2010 RECEPTOR CHARACTERISTICS C. Dopamine Receptors Dopamine (D, DA) receptors are a subclass of adrenoceptors but with rather different distribution and function. Dopamine receptors are especially important in the renal and splanchnic vessels and in the brain. Although at least 5 subtypes exist, the D1, subtype appears to be the most important dopamine receptor on peripheral effector-cells. D2 receptors are found on presynaptic nerve terminals. D1, D2, and other types of dopamine receptors also occur in the CNS. © The McGraw-Hill Companies, Inc., 2010 Adrenoceptors in ANS Gq α1 Alpha coupled receptors α2 Gi coupled Beta Gs β1,β2,β3 receptors coupled Dopamine Gs D1 receptors coupled © The McGraw-Hill Companies, Inc., 2010 Table 6-2. Should be MEMORIZED!! © The McGraw-Hill Companies, Inc., 2010 SITES OF AUTONOMIC DRUG ACTION Because of the number of steps in the transmission of autonomic commands from the CNS to the effector cells, there are many sites at which autonomic drugs may act. These sites include the CNS centers; the ganglia; the postganglionic nerve terminals; the effector cell receptors; and the mechanisms responsible for transmitter synthesis, storage, release, and termination of action. The most selective effect is achieved by drugs acting at receptors that mediate very selective actions (Table 6-4). Many natural and synthetic toxins have significant effects on autonomic and somatic nerve function. © The McGraw-Hill Companies, Inc., 2010 Table 6-5. © The McGraw-Hill Companies, Inc., 2010 INTEGRATION OF AUTONOMIC FUNCTION Functional integration in the ANS is provided mainly through the mechanism of negative feedback and is extremely important in determining the overall response to endogenous and exogenous ANS transmitters and their analogs. This process uses modulatory pre- and postsynaptic receptors at the local level and homeostatic reflexes at the systemic level. © The McGraw-Hill Companies, Inc., 2010 A. Central Integration: At the highest level—midbrain and medulla—the two divisions of the ANS and the endocrine system are integrated with each other, with sensory input, and with information from higher CNS centers, including the cerebral cortex. These interactions are such that early investigators called the parasympathetic system a trophotropic one (ie, leading to growth) used to “rest and digest” and the sympathetic system an ergotropic one (ie, leading to energy expenditure), which is activated for “fight or flight.” major influence on sympathetic outflow from the vasomotor center. © The McGraw-Hill Companies, Inc., 2010 B. Systemic Reflexes: Systemic reflexes include mechanisms that regulate blood pressure, gastrointestinal motility, bladder tone, airway smooth muscle, and other processes. The control of blood pressure-by the baroreceptor neural reflex and the renin-angiotensin-aldosterone hormonal response- is especially important. These homeostatic mechanisms have evolved to maintain mean arterial blood pressure at a level determined by the vasomotor center and renal sensors. Any deviation from this blood pressure "set point" causes a change in ANS activity and renin-angiotensin-aldosterone levels. These changes are very important in determining the response to conditions or drugs that alter blood pressure. For example, a decrease in blood pressure caused by hemorrhage causes increased SANS discharge and renin release. As a result, peripheral vascular resistance, venous tone, heart rate, and cardiac force are increased by norepinephrine released from sympathetic nerves. Blood volume is replenished by retention of salt and water in the kidney under the influence of increased levels of aldostetone. © The McGraw-Hill Companies, Inc., 2010 Figure 6–7. © The McGraw-Hill Companies, Inc., 2010 These compensatory responses may be large enough to overcome some of the actions of drugs. For example, the treatment of hypertension with a vasodilator such as hydralazine will be unsuccessful when the compensatory tachycardia (via the baroreceptor reflex) and the salt and water retention (via the renin system response) are not prevented through the use of additional drugs. It is therefore essential that the clinician understand this homeostatic system. © The McGraw-Hill Companies, Inc., 2010 C. Local lntegration: A. Presynaptic Regulation: Local feedback control has been found at the level of the nerve endings in all systems investigated. The best documented of these is the negative feedback of norepinephrine upon its own release from adrenergic nerve terminals. This effect is mediated by α2 receptors located on the presynaptic nerve membrane. Presynaptic receptors that bind the primary transmitter substance and thereby regulate its release are called autoreceptors. Transmitter release is also modulated by other presynaptic receptors (heteroreceptors); in the case of adrenergic nerve terminals, receptors for acetylcholine, histamine, serotonin, prostaglandins, peptides, and other substances have been found. Presynaptic regulation by a variety of endogenous chemicals probably occurs in all nerve fibers. © The McGraw-Hill Companies, Inc., 2010 © The McGraw-Hill Companies, Inc., 2010 C. Local integration: B. Postsynaptic Regulation 1. modulation by previous activity at the primary receptor. Up- regulation and down-regulation are known to occur in response to decreased or increased activation, respectively, of the receptors. An extreme form of up-regulation occurs after denervation of some tissues, resulting in denervation supersensitivity of the tissue to activators of that receptor type. Example: In skeletal muscle, for example, nicotinic receptors are normally restricted to the end-plate regions underlying somatic motor nerve terminals. Surgical denervation results in marked proliferation of nicotinic cholinoceptors over all parts of the fiber, including areas not previously associated with any motor nerve junctions. © The McGraw-Hill Companies, Inc., 2010 C. Local lntegration: B. Postsynaptic Regulation A pharmacologic supersensitivity related to denervation supersensitivity occurs in autonomic effector tissues after administration of drugs that deplete transmitter stores and prevent activation of the postsynaptic receptors for a sufficient period of time. Example: prolonged administration of large doses of reserpine, a norepinephrine depleter, can cause increased sensitivity of the smooth muscle and cardiac muscle effector cells served by the depleted sympathetic fibers. © The McGraw-Hill Companies, Inc., 2010 C. Local lntegration: B. Postsynaptic Regulation: 2. The second mechanism involves modulation of the primary transmitter-receptor event by events evoked by the same or other transmitters acting on different postsynaptic receptors. Example: Ganglionic transmission. Postsynaptic modulatory receptors, including 2 types of muscarinic receptors and at least 1 type of peptidergic receptor, have been found in ganglionic synapses, where nicotinic transmission is primary. These receptors may facilitate or inhibit transmission by evoking slow excitatory or inhibitory postsynaptic potentials (EPSPs or IPSPs). © The McGraw-Hill Companies, Inc., 2010 Figure 6–8. The postganglionic cells are activated (depolarized) as a result of binding of an appropriate ligand to a neuronal nicotinic acetylcholine receptor. The resulting fast excitatory postsynaptic potential (EPSP) evokes a propagated action potential if threshold is reached. This event is often followed by a small and slowly developing but longer-lasting hyperpolarizing after potential—a slow inhibitory postsynaptic potential (IPSP). This hyperpolarization involves opening of potassium channels by M2 cholinoceptors. The IPSP is followed by a small, slow excitatory postsynaptic potential caused by closure of potassium channels linked to M1 cholinoceptors. Finally, a late, very slow EPSP may be evoked by peptides released from other fibers. These slow potentials serve to modulate the responsiveness of the postsynaptic cell to subsequent primary © The McGraw-Hill Companies, Inc., 2010 Table 6-4. © The McGraw-Hill Companies, Inc., 2010 Complex Organ Control: The Eye The eye contains multiple tissues with various functions, several of them under autonomic control (Figure 6-5). The pupil, discussed previously, is under reciprocal control by the SANS (via α receptors on the pupillary dilator muscle) and the PANS (via muscarinic receptors on the pupillary constrictor). The ciliary muscle, which controls accommodation, is under primary control of muscarinic receptors innervated by the PANS, with insignificant contributions from the SANS. The ciliary epithelium, on the other hand, has important β receptors that have a permissive effect on aqueous humor secretion. Each of these receptors is an important target of drugs that are discussed in the following chapters. © The McGraw-Hill Companies, Inc., 2010 Figure 6-9. © The McGraw-Hill Companies, Inc., 2010 Complex Organ Control: The Eye Parasympathetic nerve activity and muscarinic cholinomimetics causes contraction by of the circular pupillary constrictor muscle…leads to miosis. Ciliary muscle contraction causes accommodation of focus for near vision. Ciliary muscle contraction reduces intraocular pressure. Alpha adrenoceptors mediate contraction of the radially oriented pupillary dilator muscle fibers in the iris…result in mydriasis. Beta adrenoceptors on the ciliary epithelium facilitate the secretion of aqueous humor. Blocking these receptors (with β-blocking drugs) reduces the secretory activity and reduces intraocular pressure, providing another therapy for glaucoma. © The McGraw-Hill Companies, Inc., 2010