Biochemistry PDF
Document Details
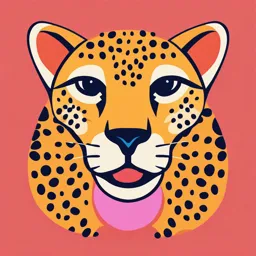
Uploaded by RefreshedJudgment
The University of Jordan, Faculty of Medicine
2022
Lajneh Teejan
Tags
Summary
This document explains biochemistry topics, specifically enzyme regulation. It discusses different types of regulation, including allosteric regulation and covalent modification. The document also presents examples and mechanisms for these processes.
Full Transcript
34 Lajneh Teejan Lajneh Teejan Nafez Abu-Tarboush Regulation Through Conformational Changes ➢ Another way of regulating the enzymatic function, is to change geometry of the enzyme itself (conformational change) so you can control the enzymatic catalysis, enzyme action and enzyme processivity. The...
34 Lajneh Teejan Lajneh Teejan Nafez Abu-Tarboush Regulation Through Conformational Changes ➢ Another way of regulating the enzymatic function, is to change geometry of the enzyme itself (conformational change) so you can control the enzymatic catalysis, enzyme action and enzyme processivity. There is more than one way regulating the enzymatic reaction through playing in the conformation of the enzyme. ➢ These regulatory mechanisms include: A. Allosteric (Allo-far away, steric-far away) activation and inhibition. B. Phosphorylation or other covalent modification. C. Protein-protein interactions between regulatory & catalytic subunits or between two proteins. D. Proteolytic cleavage. (irreversible) ➢ These types of regulation can rapidly change an enzyme from an inactive form to a fully active conformation or a fully active form to an inactive form 3.A-Not All Enzymes Follow Michaelis-Menten equation ➢ As we said before when Michaelis-Menten studied huge number of enzymes it appeared to them that we have two classes of enzymes according to their behavior: certain enzymes gave a hyperbolic plot and we called them Michaelis-Menten enzymes (simple enzymes) because they follow the Michaelis-Menten kinetics and equation. ➢ And other enzymes don’t follow it (complex enzymes) and we called them allosteric enzymes and their plot is sigmoidal. ➢ Chymotrypsin: Specificity for aromatic residues mainly. Also, hydrolysis of ester bonds. (simple enzyme) ➢ Aspartate transcarbamoylase (ATCase): synthesis of CTP & UTP for RNA and DNA synthesis. (complex/sigmoidal) cooperative platea u y و كبير بxتغيير قليل ب y و قليل بxتغيير كبير ب ➢ What does a sigmoidal plot mean? That this enzyme has two states (high affinity, low affinity), this enzyme has a cooperative behavior (Cooperativity is a phenomenon displayed by enzymes or receptors that have multiple binding sites where the affinity of the binding sites for a ligand is increased, positive cooperativity, or decreased, negative cooperativity, upon the binding of a ligand to a binding site), and since it has cooperative behavior it’s structure should be quaternary, it should have more than one subunit. ➢ All enzymes that have a sigmoidal plot we call them allosteric ➢ ❖Allosteric Regulation ➢ You change things far away from the reaction (like a remote control) ➢ Allosteric enzymes have quaternary structure with multiple subunits. (Allosteric means controlling the enzyme from far place). ➢ These enzymes have regulatory and catalytic subunits. • Catalytic subunits: are responsible of the reaction, can act as a regulatory subunit for the other subunits (like Hemoglobin when one subunit binds an oxygen it will motivate the other subunits to bind even though it was not a catalytic enzyme but both have the same cooperative behavior). • Regulatory subunits: are responsible of binding regulatory materials which affects the reaction either negatively (inhibitors) or positively (activators), these materials are called effectors (modifiers). ➢ Binding of something on regulatory subunit will change behavior of the other subunit, it triggers a conformational change in the active site (activation or inhibition) ➢ If the effector material is the same material that binds to the active site(substrate), then we will call it homotropic effector (such as O2 in hemoglobin) and when it’s not the same we call it heterotopic. ➢ Homotropic (same substrate) vs Heterotropic (different substrate) ➢ Allosteric enzymes have sigmoidal plot (S curve), Michaelis-Menten can’t explain the kinetic properties ➢ When an inhibitor binds to regulatory subunit and affect the catalytic subunit the sigmoidal curve will shift to the right (more sigmoidal, less hyperbolic). ➢ While binding of activators will shift the curve to the left (less sigmoidal, more hyperbolic, higher velocity/lower The effect of the modulators concentration) (allosteric modifiers) delay ➢ However, allosteric enzymes plot will never become hyperbolic as simple enzymes (it will only become more hyperbolic less sigmoidal, and when zooming you will see the lag/delay in the x-axis). ➢ Allosteric inhibitors have a much stronger effect on the enzyme velocity ➢ Vmax in allosteric enzymes will stay the same, in the presence of inhibitor initial velocity will be less and we will need more substrate concentration to reach Vmax, while in the presence of activators we can reach Vmax with lower substrate concentration, so Vmax value is not changing. ➢ The substrate concentration at half of the Vmax is called (K0.5). ➢ What about Km?? K0.5 is the same as Km but we don’t use Km because its specific for Michaelis-Menten kinetics and it doesn’t apply on allosteric enzymes so just for the naming to be right we don’t use Km. ➢ In the presence of inhibitor K0.5 will increase, while activators will decrease it, in both cases Vmax stays the same. ❖How do allosteric enzymes work? ➢ Two conformations: more active (R) & less active or inactive (T) ➢ Allosteric enzymes are proteins in their nature, so they exist in two states: 1) R state: it means relaxed; this state has high affinity toward its substrates. 2) T state: it means tight; this state has low affinity for substrate The equilibrium ratio (T/R) is called L and assumed to be high, this ratio is kept high (T is more than R to control the enzymatic action and to keep it regulated). ➢ As L (T/R) increases, the shape becomes more sigmoidal (it will shift more and more to the right, like the inhibitors) -green graph ➢ As L (T/R) decreases, the shape becomes more hyperbolic (it will shift more and more to the left) -blue graph ➢ L will never =1 because that means that it follows the Michaelis-Menten eqn (hyperbolic plot) ❖Concerted or Sequential ➢ Proteins and enzymes that are composed of multiple subunits follow two models in changing their structure: 1) Concerted model: all subunits change their structure at the same time. 2) Sequential model: changing of one subunit structure changes the structure of the next one and so on ➢ Some proteins and enzymes follow both models, such as hemoglobin.(proteins and enzymes act the same) ➢ Either substrate or activator must be increased to overcome the effects of the allosteric inhibitor ➢ Conformational change ❖Allosteric regulation-ATCase “synthesis of pyrimidine nucleotides” ➢ We will talk about ATCase (aspartate transcarbamoylase) which is an allosteric enzyme. ➢ ATCase and Hb are allosteric proteins (cooperative behavior) ➢ Catalytic can be separated from regulatory (hyperbolic) ➢ Cooperativity in relation to substrate ➢ It transfers a carbamoyl group to aspartate (Aspartate is the substrate as the name of ATCase implies) and the end results of the reaction are UTP & CTP. ➢ The product is the inhibitor ➢ UTP & CTP are used in DNA and RNA synthesis. ➢ This allosteric enzyme is composed of 12 subunits (6 catalytic (3 over another 3 subunits) in green & 6 regulatory in brown) they have a cooperative behavior (activator or inhibitor on the regulatory while the substrate on the catalytic) ➢ CTP is an inhibitor of ATCase -when its concentration increases- (feedback inhibition), ATP is the activator (it needs to be added because it’s not a part of the main pathway), because UTP & any substance will need a high concentration in order to regulate a CTP are nitrogenous bases that are used to reaction make DNA & RNA and when the ATP has a high concentration to regulate the reaction ➢ ➢ ➢ ➢ ➢ we need more of the others (UTP,CTP,GTP…) to couple them with the ATP and make the DNA &RNA In the photo: 1)The uninhibited enzyme is shown in blue. 2)In the presence of the inhibitor which is CTP (it’s a heterotopic inhibitor because the substrate is aspartate) the shape becomes more sigmoidal. 3)The activator of this enzyme is ATP, so once you have a lot of ATP it activates ATCase to make more nitrogenous bases (CTP & UTP) to combine with the other nitrogen bases to make DNA and RNA Remember: ATP is not used as energy provider only; it’s also used in synthesizing DNA & RNA. CTP makes feedback inhibition when it increases above physiological limits, because logically it should inhibit the enzyme from making more UTP & CTP because we have enough. Once we have more ATP and we don’t have UTP & CTP the enzyme will work to form more of them. Experiment was made by separating regulatory subunits from catalytic subunits in an allosteric enzyme, so what happened? It became simple enzyme, so Michaelis-Menten equation can be applied on it, and the result of its plot will be hyperbolic. 3.B-Conformational Changes from covalent modification ➢ Another way of regulating enzymatic function through playing with conformational changes is the covalent modification. ➢ Covalent modification happens by binding materials with the enzyme covalently (not in the active side), thus changing its conformation, accordingly active site conformation will change, resulting in altering enzymatic activity. 1)Phosphorylation ➢ Is the most common covalent modification that happens on enzymes, it follows the general mechanism (Illustrated above), but does it activate or inhibit enzymes? • It activates some and inactivate others, why? Because enzymes in pathways are different from each other, and you know that there are metabolic and anabolic reactions in the cells, and it doesn’t make sense to have both reactions at the same time, so phosphorylation activates some enzymes and inhibits others according to the cell needs. ➢ For example, when we want to build up glucose in any cell, phosphorylation activates enzymes that are responsible of building glucose up and inhibits other enzymes that destroy it. ❖ why is it effective? a) Adds two negative charges: new electrostatic interactions and accordingly conformation b) Can form three or more hydrogen bonds: specific interactions with hydrogen-bond donors c) Can take place in less than a second or over a span of hours d) Often causes highly amplified effects, because it occurs through kinases (enzymes) that can convert reactants into products in huge amounts e) Because the phosphate is big, bulky and carries negative charge so it can change the conformation it can change the activity rapidly ➢ Rapid and transient regulation of enzyme activity – REVERSIBLE (even though it’s covalent but it binds to the regulatory site and not the active one) ➢ Phosphorylation: (Ser, Thr, & Tyr), phosphorylation happens on specific places in proteins, as it needs oxygen to bind to it, so it works specifically on (Ser, Thr, Tyr)because they have a hydroxyl group in their side chains, and it does not occur randomly ✓ Mostly, ATP is the donor ✓ Kinases vs. phosphatases, you should know that kinases (transferases) add phosphate, while phosphatases (hydrolases) remove it ✓ Phosphorylation does not lead always to activation of enzymes ✓ An example on enzymes that make phosphorylation is glycogen phosphorylase. • As its name implies, its substrate is glycogen, and its function is phosphorylating glycogen. So, it comes to the last glucose and phosphorylates it (peripheral/trminal glucose), then it goes to the next one and so on. • When we need glucose in blood, glycogen phosphorylases do their function and release glucose to blood. Also, this enzyme releases glucose when epinephrine (adrenaline) concentration is high in blood when you are afraid. • Glycogen phosphorylase has two serine residues in its structure where they can be phosphorylated by Glycogen phosphorylase kinase enzyme (the substrate is Glycogen phosphorylase), when they are phosphorylated, the enzyme becomes more active and we call it ▪ ➢ ➢ ➢ • phosphorylase a, the unphosphorylated one in the less active form and it is called phosphorylase b, unphosphorylated by Glycogen phosphorylase phosphatase. • Glycogen phosphorylase kinase is the responsible enzyme of adding phosphate, while phosphatase is the responsible of removing it • Glycogen phosphorylase-reaction (two forms; a & b). Ser is away from the active site The two forms of the enzyme Both phosphorylase b and phosphorylase a exist as equilibria between an active R state and a less-active T state Phosphorylase b is usually inactive because the equilibrium favors the T state (it has both states) Phosphorylase a is usually active because the equilibrium favors the R state (it has both states) Note: The transition of phosphorylase b between the T and the R state is controlled by the energy charge of the muscle cell. The longer arrow represents the T state The longer arrow represents the R state ❖Protein Kinase A (PKA) ➢ Protein kinase A (PKA): refers to a family of enzymes whose activity is dependent on cellular levels of cyclic AMP (cAMP), A refers to cAMP, allosteric ➢ cAMP: referred to as a hormonal 2nd messenger ➢ Either dedicated or not ➢ Has several functions in the cell, including regulation of glycogen, sugar, & lipid metabolism ➢ Hormone binds to a receptor causing changes in its structure→ effects on G protein → affects adenylate cyclase enzyme so it converts ATP to cAMP (2nd messenger) → cAMP binds to protein kinase A which is composed of 4 subunits, 2 catalytic, 2 regulatory (regulatory subunits bind to 4 cAMP) → when cAMP binds to regulatory subunits it causes conformational change which causes dissociation of catalytic subunits from regulatory subunits and now catalytic subunits become active → phosphorylates glycogen phosphorylase kinase which becomes more active →they phosphorylate glycogen phosphorylase which becomes more active → phosphorylates glycogen → releasing glucose to the solution. (This is called phosphorylation cascade). ➢ Adrenaline (epinephrine) → ↑cAMP → activates protein kinase A →phosphorylates & activates glycogen phosphorylase kinase →phosphorylates & activates glycogen phosphorylase ➢ Phosphorylation cascade: phosphorylation followed by another one ➢ This signaling is stopped by many things, one of them is phosphodiesterase (PDE) which breaks cAMP converting it to AMP (inactive form) by breaking the cycle in cAMP, so they won’t activate more and more protein kinase (Catalytic subunit is responsible of activating phosphodiesterase). ❖Other Covalent Modifiers 1. Adenylylation (addition of adenylyl group). AMP (from ATP) is transferred to a Tyr hydroxyl by a phosphodiester linkage. The addition of bulky AMP inhibits certain cytosolic enzymes. 2. Uridylylation (addition of uridylyl group). 3. ADP-ribosylation: inactivates key cellular enzymes 4. Methylation: masks a negative charge & add hydrophobicity on carboxylate side chains 5. Acetylation (-ve charge): masks positive charges when added to lysine residues 3.C-Conformational Changes from Protein-Protein Interaction ➢ G protein: a family of trans-membrane proteins causing changes inside the cell. They communicate signals from hormones, neurotransmitters, and other signaling factors ➢ Why did we talk about G-protein in an enzyme lecture?! Because they work as enzymes; the Alpha subunit has an internal hydrolytic activity which works slowly ➢ G-protein is usually 3 subunits: 1- Alpha which stands by itself, and it has a fatty acid that connect it to the membrane from inside and works as an enzyme 2- Beta 3- Gamma: Beta is connected to Gamma and Gamma has a fatty acid that connect it to the membrane from inside. ➢ So, Beta-Gamma works as a dimer and Alpha works as a monomer, the three subunits are associated together. ➢ When they bind guanosine triphosphate (GTP), they are 'on', and, when they bind guanosine diphosphate (GDP), they are 'off‘ ➢ α-Subunit can be stimulatory or inhibitory ➢ When a hormone binds to the cell surface, the receptor (G protein coupled receptor in blue) will have conformational changes because it’s a protein→ the conformational change will affect its relationship with the G-protein (it will affect alpha subunit because alpha is the one that is attached to it directly) → the conformational change in Alpha will make it exclude the GDP (leave it), it can’t phosphorylate the GDP which it has→ it removes the GDP(low affinity) and replace it with a new GTP(high affinity) so we exchange them→ once it’s attached to GTP another conformational change will happen, the affinity between the Alpha & Beta-Gamma now is very low →Alpha will be released(not released exactly, it starts moving in the inner surface membrane) because it’s detached from Beta-Gamma (always bound to each other) and looks for a protein or an enzyme which it can bind to (ex. Adenylate cyclase which can convert the ATP into cAMP (Recall: the cAMP has an effect on PKA)) ➢ So, how to end this? →Because Alpha subunit has an internal enzymatic/hydrolatic activity which acts slowly (it has a slow hydrolytic activity, the Alpha subunit works as a hydrolase; it hydrolysis phosphoanhydride bond which present between the phosphate groups) → it removes one phosphate from GTP and we will have GDP again → once it’s GDP; it comes back to its original shape →conformational change will happen again and the affinity for Beta-Gamma will return high, and Alpha will bind again to Beta-Gamma and the receptor and we are finished. ❖Monomeric “G” Protein ➢ There are other forms of G-protein which are not trimeric in their nature, we call them monomeric G-proteins (has one monomer/subunit); it behaves exactly like the Alpha subunit (exchange between the GDP and GTP) → once the GTP is attached slow hydrolases of GTP into GDP occurs → if the GTP is bond its active, if the GTP is hydrolyzed to GDP it’s inactive and it gets back to its original shape. ➢ Same as Gα ➢ Hydrolysis (GTP to GDP) vs. exchange (GDP to GTP) ➢ Activation or inhibition ➢ Example: RAS protein /RAPH 3.D-Proteolytic Cleavage Zymogens (pro-or -gen) ➢ Irreversible cut usually at the N-terminus: You cut something from the enzyme irreversibly usually at the N-terminus (hydrolytic reaction); it won’t come back again; you produce an inactive form of an enzyme then you cut a piece of it to become active. ▪ Why do I synthesize an enzyme in an inactive form and then when I need it, I will convert it to an active form? 1.Place of synthesis for the enzyme is different than the place of acting, I’ll send it to another place to work on, so I should synthesize it in an inactive ▪ ▪ ▪ ▪ • • • • • ▪ form, once it reaches the place where it should act it will be activated by proteolytic cleavage. Ex: intestinal enzymes (released by Pancreas) 2.To reduce time response; in the case of blood clotting, I won’t wait for a cut to happen to produce the enzyme (I have bleeding, life threatening) so you need the enzymes to be there all the time; you produce them in an inactive form and keep them there once you need them you will only activate them.(Pepsinogen in the stomach/Fibrinogen and prothrombin) Zymogens: enzymes that are synthesized in an inactive form and then they will be converted to their active form through proteolytic cleavage (I’ll cleave part of the enzyme to open the active site). How can I know these zymogens? From their name, you must memorize them, the name either will be preceded by the prefix (pro) or it will be followed by the suffix (gen). Examples: Pepsinogen in the stomach will become pepsin, why do we need it to stay in the stomach? so once you eat you won’t go and produce the enzyme you will only activate it. If you will wait to produce the enzyme you may enter a hypoglycemic shock while waiting because you’re eating to absorb energy. When you eat, the parietal cells will start secreting HCl in the stomach, and it will activate some pepsinogen and it becomes pepsin, once the pepsin is active it will activate all other molecules of pepsinogen (we call it auto catalytic activation). Trypsinogen becomes Trypsin. Chymotrypsinogen becomes Chymotrypsin. Pro-Carboxy peptidase a becomes Carboxy Peptidase a. Pro-Carboxy peptidase b becomes Carboxy Peptidase b. Pro-Elastase becomes Elastase. These enzymes are secreted from Pancreas (digestive enzymes) ➢ Trypsin,chymotrypsin,pepsin (trypsinogen, pepsinogen chymotrypsinogen) ✓ Chymotrypsinogen: single polypeptide chain (245 residues), 5 (S—S) bonds ➢ Blood clotting ✓ The soluble protein fibrinogen is converted to the insoluble protein fibrin End Of Sheet 3