RCSI CNS Phys 2024 Blood Brain Barrier CSF Intracranial Pressure PDF
Document Details
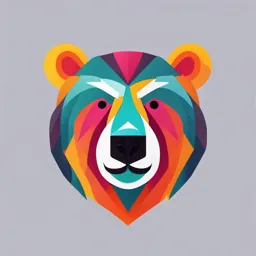
Uploaded by FormidablePennywhistle
RCSI Medical University of Bahrain
2024
RCSI
Tags
Summary
This document is a past paper from RCSI for the year 2024, covering the topics of the blood-brain barrier, cerebrospinal fluid, and intracranial pressure. It includes multiple questions and diagrams. It's an undergraduate-level neuroscience exam paper.
Full Transcript
RCSI Royal College of Surgeons in Ireland Coláiste Ríoga na Máinleá in Éirinn Blood brain Barrier, Cerebrospinal fluid, Intracranial Pressure Class Year 2 Semester 1 Course CNS Code CNS Title Blood Brain Barrier, Cerebrospinal fluid, Intracranial Pressure Lecturer Dr. Melanie...
RCSI Royal College of Surgeons in Ireland Coláiste Ríoga na Máinleá in Éirinn Blood brain Barrier, Cerebrospinal fluid, Intracranial Pressure Class Year 2 Semester 1 Course CNS Code CNS Title Blood Brain Barrier, Cerebrospinal fluid, Intracranial Pressure Lecturer Dr. Melanie Föcking (RCSI-IE): Dr. Colin Greengrass (RCSI-BH) Date November 2024 ALO173 Describe the different functions of the CSF Describe how and where CSF is formed in the ALO174 brain ALO175 Describe the formation of brain interstitial fluid DON’T FORGET ALO176 Outline the importance of the blood-brain barrier THE SIGNS Describe intracranial pressure and the ALO177 consequences of increased intracranial pressure ALO178 Explain Cushing’s reflex Functions of the Cerebrospinal Fluid (CSF) CSF is a clear, colourless fluid found in the brain and spinal cord, serving as a cushion and providing basic mechanical and immunological protection to the brain. CSF is located in the subarachnoid Cerebrospinal Fluid (CSF) space, ventricles of the brain, and the central canal of the spinal cord You don’t have to learn the content of this diagram Reduces the effective Functions of the weight of the brain Cerebrospinal from about 1.4 kg to Fluid (CSF) about 50g Mechanisms/Functions: Buoyancy: CSF counteracts the weight of the brain, ensuring it is neutrally buoyant. This protects the brain from injury due to gravity. Functions of the Cerebrospinal Fluid (CSF) Mechanisms/Functions: Protection: Acts as a cushion against sudden impacts or blows. It supports and provides mechanical cushioning for the brain. Cushioning effect allows the brain to withstand normal minor traumas associated with everyday living. CSF in the spinal subarachnoid space prevents the spinal cord from coming into direct contact with vertebral bones Abnormal Trauma Abnormal trauma leads to brain injury. Examples: Contrecoup injury: CSF, surrounds and cushions the brain, but can affect the dynamics of contrecoup injuries. It acts as a shock absorber and can mitigate the force of the brain's impact against the skull. However, rapid deceleration or acceleration of the head can cause the brain to slosh within the CSF, leading to contrecoup injuries. These injuries involve damage to brain tissue on the side opposite to the initial impact. Depressed skull fracture: When skull is fractured and bone is driven into brain tissue. Functions of the CSF contains 1. Glucose Cerebrospinal Fluid CSF Water very little protein 2. Albumin 99.13% 3. Urea (CSF) compared to blood 4. Amino acids Maintaining Chemical Organic 5. Globulins immunoglobulins Stability 6. Lactate: Maintains an optimal 7. Enzymes 8. Neurotransmitters ionic concentration for 9. Cells: mostly white blood cells neuronal functioning Solutes 1. Sodium (Na+) Helps in buffering pH 0.87% 2. Chloride (Cl-) and removing waste 3. Potassium (K+) products Inorganic 4. Bicarbonate (HCO3-) 5. Calcium (Ca2+): CSF is higher in sodium, chloride, and magnesium compared to 6. Magnesium (Mg2+) plasma and lower in potassium, calcium, and glucose 7. Trace metals (zinc, copper, iron) The Blood Brain Barrier (BBB) The endothelial cells surrounding the capillaries are connected by tight junctions This combined with astrocytes and other glia, form the blood brain barrier The BBB acts as a continuous sheath of tissue covering and sealing the capillary wall. This restricts free movement of substances from blood into CSF Formation of CSF in the Brain ALO174 – Describe how and Subarachnoid space where CSF is formed in the brain. Choroid Plexus: The primary site of CSF production. Formed by tufts of capillaries and their associated membranes which invaginate into the lateral, third and fourth ventricles. The majority of CSF is formed in the lateral venticle Choroid plexus forms 70% of CSF. Remainder by other brain capillaries You don’t have to learn the content of this diagram What is the blood-CSF barrier? This is the barrier formed by the choroid plexus and certain other brain capillaries and their The B-CSF-B consists associated transporters. of two cell layers Transporters promote entry of some substances and restrict entry of others into CSF from blood. Endothelial Choroid cells of the Plexus Therefore, the barrier regulates capillary wall Epithelial cells the entry of substances from blood into CSF Choroid Plexus The endothelial cells lining capillaries in the choroid plexus are fenestrated, which means they have small pores that allow for the transfer of water and small solutes. CPECs or Choroid Plexus Epithelial Cells are connected by tight junctions. CPECs actively transport ions and water to generate CSF, while also facilitating the uptake of nutrients and the excretion of waste, ensuring brain homeostasis. Villi: CPECs extend into the ventricles as villi, increasing surface Formation of CSF in the Brain. area for CSF production and secretion. 1: Filtration by Endothelial Cells Allow plasma filtration, Fenestrated capillaries excluding larger blood in the choroid plexus cells/proteins At Choroid Plexus Epithelial Cells (CPECs) Allow active ion Tight junctions forming transport and osmotic the BCSFB gradient creation Choroid Plexus Epithelial Cell The diagram depicts the blood-CSF barrier at the choroid plexus, illustrating: Tight junctions in epithelial cells for selective permeability. Fenestrated capillaries allowing substance exchange. Ion transporters and aquaporin for CSF ionic balance and water regulation. Specific transporters managing nutrient uptake and waste removal. Carbonic anhydrase maintaining CSF pH balance. Exosome release for cellular communication You don’t have to learn the content of this diagram Role of the Barrier Formation: CPEC Cells CPECs form the blood-cerebrospinal fluid barrier (BCSFB) with tight junctions, restricting substance movement between blood and CSF. Ion Transport: Actively transport ions (Na⁺, K⁺, HCO₃⁻) into CSF to maintain electrolyte balance and pH. Selective Secretion: Secrete ascorbic acid (vitamin C) and growth factors, enhancing CSF’s nutritive composition. Selective Transport: Utilize transporters for selective molecule entry into CSF, e.g., glucose transporters. Waste Removal: Remove waste products and harmful substances, preventing their buildup in CSF. P-glycoprotein Expression: Express P-glycoproteins to pump out lipid-soluble xenobiotics and drugs, enhancing barrier protection. Permeability SAME AT CHOROID PLEXUS AND BLOOD BRAIN BARRIER High permeability to lipid-soluble solutes and small molecules Sterols such as Oestradiol O2 CO2 Lipid Soluble Drugs Inhaled Anaesthetics etc. Free and unrestricted movement in and out of the cell and nucleus Permeability SAME AT CHOROID PLEXUS AND BLOOD BRAIN BARRIER Minimal permeability Permeability to water- to larger water- soluble solutes via a soluble solutes specific transporter Proteins Glucose Most Drugs Amino Acids Blood Cells Ions Free and unrestricted movement in and out of the cell and nucleus Phenytoin Is highly lipid P Glycoproteins soluble P-Glycoprotein Changes shape (conformation change) And expels to lipid soluble molecule Lipid soluble molecules can traverse the membrane easily ATP (via kinases) Drives the p-glycoprotein efflux pump You don’t have to learn the content of this table Plasma (Typical CSF Composition Component Values) CSF (Typical Values) Notes Sodium concentrations are Sodium (Na+) In Detail 135-145 mmol/L 138-145 mmol/L fairly similar in both fluids. Potassium is lower in CSF Potassium (K+) 3.5-5.0 mmol/L 2.5-3.0 mmol/L than in plasma. Chloride concentration is Chloride (Cl-) 98-107 mmol/L 119-132 mmol/L usually higher in CSF. The composition of Similar levels in both fluids but can vary based on acid- cerebrospinal fluid (CSF) and Bicarbonate (HCO3-) 22-30 mmol/L 18-27 mmol/L base balance. plasma is quite different due Calcium is lower in CSF than Calcium (Ca2+) 2.2-2.6 mmol/L 1.1-1.3 mmol/L in plasma. to the selective permeability Exact concentration in CSF can vary but is generally of the blood-brain barrier. Magnesium (Mg2+) 0.65-1.05 mmol/L Less than plasma lower. 3.9-5.5 mmol/L (70- 2.2-3.9 mmol/L (40-70 Glucose in CSF is about 60% Glucose 100 mg/dL) mg/dL) of the plasma level. [Na+] and [Cl-] > [Na+] and Protein is significantly lower [Cl-] in plasma Proteins 6.0-8.3 g/dL 0.15-0.45 g/dL in CSF; Albumin is the most common protein in CSF. [K+] < [K+] in plasma Urea in CSF is roughly About 60% of plasma proportional to plasma but [glucose] < [glucose] in Urea 3.6-7.1 mmol/L level slightly less. Slight differences in pH plasma pH 7.35-7.45 Approximately 7.3 values. Almost no protein Osmolarity 275-295 mOsm/kg Similar to plasma Both CSF and plasma have a similar osmolarity. CSF normally has very few cells, increased white cells Primarily red and in CSF can indicate Cells white cells Few white cells disease. Osmotic Pressure and the CSF Primary site: Increased Na+ in Water follows the Free water Choroid plexus ventricles creates osmotic gradient. molecules epithelial cells. a high solute Aquaporins combine with ions Active secretion of concentration. facilitate water to form CSF. Establishing Osmotic Water Movement via Circulation Ion Transport in Choroid Aquaporins Plexus Gradient CSF Formation and ions (especially Osmotic gradient transport from Na+) into formed across cells to ventricles. ventricles. semipermeable Na+/K+ ATPase membranes. pump central to ion transport. Mechanism of Water Influx into the Cerebrospinal Fluid Key Structures at the Choroid Plexus Ependymal Cells: Ependymal cells line the ventricles of the brain and are involved in the circulation of CSF They are not a direct part of the blood-CSF barrier. Instead, they line the ventricular system and help in the movement of CSF through the ventricles, but they do not have the same regulatory or barrier functions as the choroid plexus epithelial cells. Volume of CSF CSF Production: CSF Replacement: Every 6-8 hours. ~500 mL/day. Turnover rate is about 3-4 times daily CSF Volume: Total of Subarachnoid space: ~117 mL 140 mL at any time. Ventricles: ~23 mL Balance: Secretion rate = Absorption rate. Circulation - Anatomical CSF Production and You don’t have to learn the content of this diagram Production: CSF Production and CSF is produced mainly by the choroid plexus located within the brain's ventricles Circulation – In detail Circulation within Ventricles: CSF flows From the lateral ventricles, CSF flows through the interventricular foramina (foramina of Monro) CSF flows into the third ventricle. CSF then moves through the cerebral aqueduct (aqueduct of Sylvius) CSF flows into the fourth ventricle. Entry into Subarachnoid Space: From the fourth ventricle, CSF flows into the subarachnoid space Circulation Over Brain and Spinal Cord: In the subarachnoid space, CSF circulates around the brain and spinal cord Absorption into Venous System: CSF is absorbed into the venous blood system through arachnoid granulations (villi) You don’t have to learn the content of this diagram The CSF moves in a pulsative manner throughout the system CSF Circulation – Process in Brief Once it has passed all these points, the lateral ventricle, the third and fourth ventricle and the subarachnoid space, the CSF is reabsorbed by bulk flow by various arachnoid villi and transferred to the blood stream in the large venous sinuses located in folds by the dura mater You don’t have to learn the content of this diagram Arachnoid granulations, also known as arachnoid villi, play a crucial role in the circulation and drainage of cerebrospinal fluid (CSF) within the brain. Location: Arachnoid granulations are located primarily along the superior sagittal sinus, which is a large venous channel within the dura mater of the brain. Structure: They are projections of the arachnoid membrane into the venous sinuses. These projections are covered by endothelial cells. CSF Absorption: The CSF flows from the subarachnoid space through the arachnoid granulations into the venous blood in the dural sinuses. This flow is driven primarily by the pressure gradient between the CSF in the subarachnoid space and the lower pressure in the venous system. One-Way Flow: The cells that cover arachnoid granulations can act like valves, combined with the force from the pressure gradient, this allows CSF to enter the venous system but prevents blood from flowing back into the subarachnoid space. You don’t have to learn the content of this diagram Sampling CSF – Lumbar Puncture A hollow needle is inserted between 3rd and 4th or 4th and 5th lumbar vertebrae so that the tip lies in the sub- arachnoid space. You don’t have to learn the content of this diagram Spinal cord extends only to 1st lumbar vertebra Opening Pressure Measured in cm of water (cm H₂O) or mmHg. Normal Range: Generally, 6–18 cm H₂O for adults. Opening pressure is the initial pressure of cerebrospinal fluid (CSF) measured during a lumbar puncture (spinal tap) when the needle first enters the subarachnoid space. It reflects the pressure within the CSF system and provides an indirect measure of intracranial pressure (ICP). Characteristics of a normal CSF sample Clear, colourless fluid. A few white blood cells. No red blood cells. Very little protein. [Glucose] = 70% of plasma [glucose] Changes in CSF composition resulting from CNS disease/disorder Sub-arachnoid haemorrhage: CSF becomes discoloured—”yellowish” a few hours later. This is a sign of blood. ↑ WBCs (especially neutrophils) CSF and Bacterial Infection ↑ Protein levels ↓ Glucose levels ↑ Lactate levels Presence of bacteria (visible on Gram stain or culture) ↑ CSF pressure Importance of the Blood-Brain Barrier (BBB) ALO176 – Outline the importance of the blood-brain barrier. The BBB is a highly selective semipermeable barrier that separates circulating blood from the brain and extracellular fluid in the CNS. This is formed by the walls of the cerebral capillaries, their associated transporters and astrocytes. Regulates the entry of substances into the brain cells and interstitial fluid from the blood The Blood Brain Barrier (BBB) The endothelial cells surrounding the capillaries are connected by tight junctions This combined with astrocytes and other glia, form the blood brain barrier The BBB acts as a continuous sheath of tissue covering and sealing the capillary wall. This restricts free movement of substances from blood into CSF Maintains a constant environment for the brain by strictly BLOOD BRAIN BARRIER regulating the entry and exit of substances. BLOOD BRAIN BARRIER VS PERIPHERAL CAPILLARY Endothelial cells in the BBB have special properties compared to endothelial cells in other parts of the body. They are tightly packed with tight junctions, lack fenestrations, have minimal pinocytic vesicular transport. P-glycoproteins are primarily expressed in the endothelial cells. You don’t have to learn the content of this diagram Blood Brain Barrier Versus Choroid Plexus – Structural Differences Blood-Brain Barrier (BBB): Endothelial Cells: Form a continuous lining with tight junctions. Astrocytic End-Feet: wrap capillaries, contributing to the barrier. Basement Membrane: Provides structural support. Choroid Plexus: Fenestrated Capillaries… Epithelial Tight Junctions… Villi… Ion Pumps and Transporters: Equip CPECs to actively transport ions and molecules into the CSF Blood Brain Barrier Versus Choroid Plexus – Functional Differences Blood-Brain Barrier (BBB): Selective Permeability: Strictly regulates ion and molecule passage. Protection: Shields brain tissue from pathogens and toxins. Homeostasis: Maintains CNS environment by controlling nutrient and waste transport. Choroid Plexus: CSF Production: Generates cerebrospinal fluid for the CNS. Blood-CSF Barrier: Modulates CSF composition and performs immune functions. Transport Mechanisms: Facilitates selective secretion into CSF and waste removal. The importance of knowledge of the permeability characteristics of the blood- brain barrier Protection: Prevents many pathogens, as well as potentially harmful substances from the blood, from entering the brain. Treatment: Permeability required for effective treatment of CNS disease (only drugs which cross the barrier will be effective) Avoidance of CNS side effects of drugs Neuro-oncology (Barrier is absent in blood vessels associated with tumours. Therefore, plasma proteins, which do not normally cross capillaries, will enter interstitial fluid of brain tumours) You don’t have to learn the content of this diagram Formation of Brain Interstitial Fluid Brain Interstitial Fluid (ISF) surrounds the cells of the brain, occupying the spaces between neurons and glial cells. ISF is a vital component that provides an environment for cellular exchange and plays a pivotal role in waste removal, among other functions. You don’t have to learn the content of this diagram Formation of Brain Interstitial Fluid ALO175 – Describe the formation of brain interstitial fluid Derived mainly from the blood, the brain interstitial fluid is formed when water, ions, and small molecules pass across the capillary walls and spread around the cells of the brain. Filtration: Plasma from the brain's capillaries is filtered through the BBB. Large molecules (like proteins) are generally excluded, water and smaller solutes can pass more easily. Composition: The ISF is primarily composed of water, ions (like potassium, sodium, calcium, and magnesium), amino acids, glucose, and specific small proteins. Its composition is delicately maintained to ensure proper neuronal function. Brain Interstitial Fluid Origin from Blood Plasma: The primary origin of ISF is the blood plasma. The blood-brain barrier (BBB) selectively filters blood to produce ISF. Active Transport: Specialised protein transporters in the BBB move specific ions, amino acids, and other essential nutrients into the brain parenchyma, contributing to the ISF composition. Diffusion: Small, lipid-soluble molecules can diffuse directly through the endothelial cell membranes of the BBB into the ISF. Brain Interstitial Fluid Astrocytes Role of Astrocytes: Astrocytes, a type of glial cell, play a crucial role in ISF formation and maintenance. End-feet: The processes of astrocytes, known as end-feet, envelop over 99% of the brain's capillaries. They help regulate the passage of substances from the blood into the ISF. Ion Homeostasis: Astrocytes actively take up or release ions to maintain the ionic balance in the ISF. You don’t have to learn the content of this diagram Glymphatic System Recent studies have revealed the presence of the glymphatic system, a waste clearance pathway in which ISF plays a significant role. Waste Clearance: The glymphatic system facilitates the removal of waste products from the brain, utilizing the ISF as a medium for waste transportation. Sleep Dependency: The function of the glymphatic system is notably more active during sleep, emphasizing the importance of sleep in brain health and waste clearance. https://doi.org/10.1016/j.ebiom.2022.103931 Glymphatic System Glymphatic System Clinical Relevance for ISF Abnormalities in the formation or composition of ISF can contribute to various neurological conditions. Alzheimer's Disease: Impaired ISF dynamics can lead to an accumulation of beta-amyloid proteins, associated with Alzheimer's. Oedema: Brain injury can disrupt the BBB and lead to an abnormal accumulation of ISF, causing swelling Intracranial Pressure and its Consequences ALO177 – Describe intracranial pressure and the consequences of increased intracranial pressure Intracranial pressure is the pressure exerted within the skull. Intracranial pressure (ICP) reflects the pressure within the skull from the brain tissue, blood, and CSF. Intracranial Pressure (ICP) Maintaining a balanced ICP is crucial for ensuring adequate blood perfusion to the brain Normal ICP ranges from 7-15 mm Hg in adults lying flat (supine). Variations beyond this range, particularly increases due to factors like brain oedema, haemorrhage, or excessive CSF, can lead to elevated ICP Intracranial Pressure (ICP) The Monro-Kellie doctrine postulates that the cranial cavity's volume is incompressible. Any increase in one component's volume must be counterbalanced by a decrease in another to maintain a constant ICP The Monro-Kellie Doctrine underlines the finite space within the cranial cavity. If one component's volume increases without a decrease in another, ICP will rise. Elevated ICP is a critical condition that can compress cerebral vessels, thereby reducing blood flow to the brain (cerebral ischemia). This ischemia can lead to brain tissue damage due to insufficient oxygen and nutrient supply. It can lead to brain herniation, which can be fatal. Immediate medical intervention is required to mitigate these effects. Causes of Increased ICP Traumatic brain injury Brain tumours Haemorrhage (e.g., subdural, epidural, intracerebral) Hydrocephalus Infections (e.g., meningitis, encephalitis) CT scans showing examples of tumours, haemorrhages Dangers of a rise in ICP Increased ICP Cerebral vessels compressed Cerebral blood flow Cerebral ischaemia Consequences of Increased ICP Brain herniation syndromes (e.g., uncal, central, tonsillar) Compressed brainstem → Impaired consciousness, respiratory depression Herniation is a condition in which a portion of the brain, cerebrospinal fluid (CSF) and blood vessels is displaced because of increased pressure inside the skull. Examples of causes of raised ICP Brain haemorrhage – Build-up of blood within the skull can lead to increases in intracranial pressure – Which can crush delicate brain tissue or limit its blood supply. – Severe increases in intracranial pressure can cause brain herniation Examples of causes of raised ICP Tumour – Any tumour, extra tissue, or fluid can cause pressure on the brain – Results in increased intracranial pressure may result from one or more of the ventricles that drain cerebral spinal fluid becoming blocked and causing the fluid to be trapped in the brain. Head Injury, ICP and Management Primary Injury Direct damage from initial trauma (e.g., contusions, lacerations, axonal injury). Secondary Injury Delayed damage due to cerebral ischemia, often caused by increased intracranial pressure (ICP). Focus of Management Prevent secondary injury by improving cerebral blood flow. Lower ICP by reducing cerebrospinal fluid (CSF) volume. Outcome: Effective management minimizes long-term damage and improves recovery. Manifestations of a rise in ICP Headache Nausea & vomiting Altered consciousness (from drowsiness to coma) Pupillary abnormalities Seizures Cushing's triad: hypertension, bradycardia, irregular respiration Diagnosis Clinical examination (e.g., fundoscopy for papilledema) Imaging: CT, MRI – enlarged ventricles Lumbar puncture (contraindicated if raised ICP suspected due to risk of herniation) NOTE: Cerebrospinal fluid (CSF) pressure is a component of intracranial pressure (ICP). Intracranial pressure refers to the overall pressure within the skull and thus includes the pressure exerted by the brain tissue, the CSF, and the blood within the cerebral CSF Pressure vasculature. CSF pressure is specifically the pressure exerted by the cerebrospinal fluid within the subarachnoid space and the brain's ventricular system. May be measured at lumbar puncture. Normally about 10 mm Hg Obstruction to CSF circulation leads to increased CSF pressure. Papilloedema As ICP continues to rise, it can lead to neural dysfunction, including confusion and drowsiness. Papilledema, the swelling of the optic disc, may be observed but is not always present Obstruction to CSF flow at any point may lead to hydrocephalus i.e. an increase in CSF volume. Results in enlargement of the ventricles. Hydrocephalus Brain becomes flattened against the skull leading to brain damage. Hydrocephalus Prevalence: Common in infants and adults over 60 Causes in Infants Aqueductal Stenosis: Narrowing or inflammation of the aqueduct of Sylvius. May be congenital or acquired. Genetics: Inherited cases, e.g., congenital aqueductal stenosis. Associated Conditions: Neural tube defects like spina bifida. Clinical examination (e.g., fundoscopy Hydrocephalus for papilledema) Imaging: CT, MRI Lumbar puncture Prevalence: Common in infants and adults over 60 Causes in Older Adults: A Case: Mary Aged 75 Clogged arachnoid granulations, also known as arachnoid villi, can lead to hydrocephalus, particularly in the elderly, This is a common underlying mechanism for Normal Pressure Hydrocephalus (NPH) MAP Mean arterial pressure (pressure of What is the goal of cerebral perfusion? blood entering the brain). ICP Intracranial pressure (resistance from pressure within the The brain requires a constant supply skull). of oxygen and nutrients to function. Blood flow (cerebral perfusion) is driven by a pressure gradient, primarily determined by mean arterial pressure (MAP) and opposed by intracranial pressure (ICP). MAP Mean arterial pressure (pressure of blood entering the Rigid Cranium brain). ICP Intracranial pressure (resistance from pressure within the The skull is a fixed, enclosed space skull). containing: Brain tissue. Cerebrospinal fluid (CSF). Blood vessels. The Monro-Kellie Doctrine states that an increase in one component (e.g., brain swelling) must be balanced by a decrease in another to maintain normal pressure. MAP Mean arterial pressure (pressure of blood entering the Intracranial Pressure (ICP) brain). ICP Intracranial pressure (resistance from pressure within the ICP is the pressure within the skull, skull). influenced by: Brain tissue, blood, and CSF. Elevated ICP: Decreases CPP by reducing the pressure gradient between MAP and ICP. Can lead to brain ischemia (insufficient oxygen and nutrients to brain tissue). MAP Mean arterial pressure (pressure of Artery (Mean Arterial Pressure - blood entering the brain). MAP) ICP Intracranial pressure (resistance from pressure within the MAP is the driving pressure of blood skull). entering the brain through arteries. For cerebral blood flow to occur: MAP must exceed intracranial pressure (ICP). MAP Mean arterial pressure (pressure of blood entering the Vein (Central Venous Pressure - CVP) brain). ICP Intracranial pressure (resistance from pressure within the CVP reflects the pressure in veins as skull). blood exits the brain. Blood flow is driven by the gradient between: MAP (arterial inflow). CVP (venous outflow). Compression of veins due to rising ICP can impede drainage, worsening the pressure. MAP Mean arterial pressure (pressure of blood entering the Cerebral Perfusion Pressure (CPP) brain). ICP Intracranial pressure (resistance from Cerebral Perfusion Pressure (CPP) is pressure within the the pressure gradient driving blood skull). flow to the brain. It ensures delivery of oxygen and nutrients to brain tissue. Calculated as: CPP=MAP−ICPMAP MAP Mean arterial pressure (pressure of blood entering the Cerebral Perfusion Pressure (CPP) brain). ICP Intracranial pressure (resistance from Normal CPP Range between 60–80 pressure within the mmHg. skull). A CPP < 50 mmHg can lead to cerebral ischemia (insufficient blood flow). If ICP increases to levels close to or exceeding MAP, CPP drops, leading to brain ischemia and cellular dysfunction. A CPP > 100 mmHg can indicate excessive perfusion and risk of damage. How does high ICP affect CPP? Blood Vessel Compression: High ICP compresses cerebral vessels, reducing blood flow. Loss of Autoregulation: Brain's ability to maintain consistent blood flow fails at high ICP. Venous Outflow Obstruction: Compressed veins impede blood drainage, increasing ICP further. Reduced Arterial Driving Pressure: Higher ICP narrows the difference between MAP and ICP, decreasing cerebral blood flow. Mechanical Brain Compression: Deformation of brain tissue exacerbates vessel compression and ischemia. Raised ICP Increased ICP arises from trauma, haemorrhage, tumours, or other conditions. This compresses blood vessels and reduces Cerebral Perfusion Pressure (CPP). Hypoxia (lack of oxygen) and hypercapnia (CO₂ buildup) occur due to restricted cerebral blood flow. What does the body prioritize when CPP is critically low? The body’s first principle is survival: preserving blood perfusion to the brain takes precedence. This requires an immediate physiological adjustment to counteract rising ICP. 6. Cushing’s Reflex ALO178 – Explain Cushing’s reflex. Clinical Manifestations of Rising ICP Cushing’s Reflex is a physiological response to increased intracranial pressure (ICP) and is an attempt by the body to maintain cerebral perfusion (blood flow to the brain) when intracranial pressure threatens to restrict it. Purpose of Cushing's Reflex This reflex aims to preserve cerebral perfusion by ensuring that arterial pressure remains higher than ICP. Activation of the Reflex: Cushing's Reflex in Intracranial Hypertension Slowed Heart Rate (Bradycardia): This is a bit counterintuitive. When the blood pressure rises, baroreceptors sense this increase and reflexively slow down the heart rate to prevent too high a blood pressure. Cushing’s Reflex Triad of Responses (Cushing’s Triad) Hypertension: The body increases arterial blood pressure to push blood into the brain despite the high ICP, attempting to maintain perfusion. Bradycardia: Baroreceptors (pressure- sensitive receptors) detect the elevated blood pressure and slow the heart rate as a compensatory mechanism. Irregular Respiration: Increased ICP compresses the brainstem, specifically the respiratory centers, leading to abnormal breathing patterns. Clinical Perspective The appearance of Cushing's reflex in a patient often indicates a critical and potentially life-threatening increase in ICP. If unaddressed, further ICP elevation can lead to Brain ischemia (lack of oxygen and nutrients). Herniation (displacement of brain structures through rigid boundaries). Death. The brain is attempting to protect itself, but these changes can quickly lead to brain herniation and death if not promptly addressed. Management Restoring equilibrium requires addressing the underlying causes of ICP rise Reduce ICP: Hyperosmolar therapy (e.g., mannitol), draining CSF, or surgical decompression. Elevate the head of the bed (30°) Osmotherapy: Mannitol, hypertonic saline Sedation & ventilation to maintain appropriate PaCO2 levels Surgery: Decompressive craniectomy, evacuation of hematomas, shunt placement for hydrocephalus Bibliography