Ultrasound Physics and Instrumentation (Part 1) PDF
Document Details
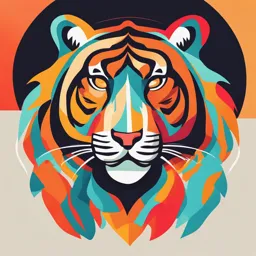
Uploaded by GloriousRhodochrosite
Dr. Ibrahim Hadadi
Tags
Summary
This document presents a lecture on ultrasound physics and instrumentation, covering topics such as the history and evolution of ultrasound technology, different types of waves, and their interactions with tissue. The presentation includes key parameters like frequency, period, wavelength, and power.
Full Transcript
3-RAD6124 Ultrasound physics and instrumentation (part 1) Radiological Sciences Department By Dr. Ibrahim Hadadi 3-RAD6124 - Ultrasound physics and instrumentation (part 1) Radiological Sciences Department By Dr. Ibrahim Hadadi The evolution of ultrasound technology...
3-RAD6124 Ultrasound physics and instrumentation (part 1) Radiological Sciences Department By Dr. Ibrahim Hadadi 3-RAD6124 - Ultrasound physics and instrumentation (part 1) Radiological Sciences Department By Dr. Ibrahim Hadadi The evolution of ultrasound technology World War I's naval warfare, Spallanzani's exploration led particularly the destruction The medical industry began Dussik published the to the discovery of sound wrought by U-boats, experimenting with pioneering study on the beyond the audible spurred the advancement ultrasound for medical ultrasound examination of spectrum. of SONAR technology. purposes. the brain. 1880 1917 Late 1940s 1794 1912 1930s 1942 The Curie brothers, Pierre Utilizing piezoelectric Diagnostic uses for and Jacques, identified the principles, Langevin created ultrasound started to piezoelectric effect, an early ultrasound device. emerge, marking a new era foundational for later in medical imaging. ultrasound technology. The evolution of ultrasound technology Ultrasound technology Institutions worldwide The real-time B-scan expanded with the advent of developed pulsed ultrasound ultrasound was developed three-dimensional (3D) and technology, leading to 'B and introduced in obstetric four-dimensional (4D) Mode' imaging. imaging. imaging. 1956 1980s 1994 1950 1965 1990s Clinical adoption of Advancements made real- Steven Kapral and his team ultrasound commenced in time ultrasound imaging pioneered the use of B-mode Glasgow, paving the way for feasible. ultrasound for brachial plexus broader medical applications. blockade procedures What is sound? Sound is an energy form generated through vibration, a mechanical action that transmits energy from one location to another. It displays as a mechanical or longitudinal wave, requiring a medium—solid, liquid, or gas. Unlike electromagnetic waves, sound cannot propagate through a vacuum. · i Sound waves are characterized by alternating compressions and rarefactions. · Ju Compressions signify an increase in pressure or density, while rarefactions occurs during the troughs of the sound wave, where the vibrating source of the sound wave moves away from the molecules, causing them to become less densely packed. What is sound wave? 9. l, el ji A wave is characterized as a disturbance or fluctuation that transfers energy from one location to another within a medium. This transfer occurs without the need for physical contact between the points. We refer to mechanical waves as longitudinal waves. These are · vsI waves in which the displacement of the medium is in the same direction as the direction of the wave's propagation. · 17 · j in O Wave Formation When a vibration occurs, it disrupts the particles within a medium. This disturbance leads to the - creation of waves that propagate - through the medium - - - Wave Formation All matter, including air, comprises molecules—tiny particles that are interconnected through elastic intermolecular forces. Classification of Waves Mechanical Waves: ~ 1 Defined by the disturbance of a physical medium. Examples include: Ocean waves Sound waves L Seismic waves Electromagnetic Waves (transverse wave): ~2 2 - These waves do not require a medium and can propagate through a vacuum. Examples include: Radio waves X-rays Light ~ longitudinal vs. transverse wave Longitudinal Waves: - Particle displacement occurs parallel to the wave's direction of energy movement. Transmission Mechanics: Requires an initial vibration from a source object. Requires a material for wave travel. The speed depends on the type and state of the medium. Transverse Waves: L Particle displacement occurs perpendicular to the wave's direction of propagation. Transmission Mechanics: Velocity is relatively constant at approximately 299,792.456.2 m/s in a vacuum, which is the speed of light. zu - & d Understanding Parameters in Acoustics Parameters can exhibit Directly Proportional: A parameter is a direct or inverse When one parameter quantifiable factor or proportional decreases, the other also characteristic. relationships. decreases. Inversely Proportional: In Key Parameters of Sound Waves: Important this relationship, a parameters to consider in sound waves include decrease in one parameter results in an Frequency, Period, Wavelength, Propagation increase in the other. Speed, Amplitude, Power, and Intensity Sound Wave Parameters: Frequency Frequency (f): Frequency measures the occurrence rate of an event. In sound, it refers to the number of complete cycles of pressure variation (or any other acoustic variable) in one second. Sound Wave Parameters: Frequency M & - A cycle is a full variation in pressure or another acoustic variable, encompassing both compression (increased density) and rarefaction (decreased density). - -- - Units of Frequency: Measured in hertz (Hz), kilohertz (kHz), and megahertz (MHz), where one hertz is equivalent to one cycle per second, one kilohertz equals 1,000 Hz, and one megahertz is 1,000,000 Hz. Sound Wave Parameters: Frequency Typical Frequency Values in medical The frequency is determined by the ultrasound: Ranging from 2 to 15 MHz. sound source. Y Frequency-Period Relationship: The product of frequency and period equals 1 second. Frequency X Period = 1 second Kov A, Frequency is the number of complete variations (cycles) that an acoustic variable (pressure, in this case) goes through in 1 second. - B, Five cycles occur in 1 second; thus the frequency is five cycles per second, or 5 Hz. C, If five cycles occur within one millionth of a second, also known as a microsecond (1 μs) (i.e., five million cycles occurring in 1 second), the frequency is 5 MHz. Resonance Frequency in Ultrasound Transducers 1650 P - The resonance frequency of an ultrasound transducer is primarily determined by its piezoelectric crystals. Thinner crystals in the transducer vibrate at higher frequencies compared to thicker crystals Frequency plays a crucial role in determining the resolution and penetration of sonographic images. It is adjustable based on the transducer and sonographic s instrument used. Els Sound Wave Parameters: Period - The period value The period is The period is cannot be altered determined by represented by by the the sound source. the symbol (T). - sonographer & Each cycle occurs in 0.2 μs, so the period is 0.2 μs. If one cycle takes 0.2 (or 1⁄5) millionths of a second to occur, it means that five million cycles - occur in 1 second, so the frequency is 5 MHz. Sound Wave Parameters: Wavelength W ① Wavelength (λ) is the length of a cycle in space. m Units for Wavelength: Measured in meters, millimeters, or any standard unit of length. Typical Values in Soft Tissue: Ranges from 0.1 to 0.8 mm. -. Control by Sonographer: The wavelength cannot be modified by the sonographer. - 55% Factors Influencing Wavelength: 1. Transducer Frequency. 2. Speed of Sound in the Medium. No Sound Wave Parameters: Wavelength Wavelength is calculated as Speed divided by Frequency Wavelength (λ) (mm) = c (mm/μs) ÷ f (MHz) - - - ·- s I. I Wavelength is a crucial parameter that influences the diagnostic quality of M siggBog ultrasound images. Shorter-wavelength sound waves have superior spatial - & resolution but less penetration. - - In this figure, each cycle covers 0.31 mm. Thus the wavelength is 0.31 mm. This figure differs from for a propagation speed of 1.54 mm/μs and a frequency of 5 MHz, the wavelength is 0.31 mm. & Sound Wave Parameters: Propagation speed 561 % E Propagation speed (c) refers to the rate at which a sound wave moves through a medium. & Sus jaS Within a specific medium, sound waves travel at a consistent speed, regardless of their frequency. This means that sound waves at 20 Hz and those at 20 MHz - - - - move at the same speed in the same medium. - The speed of sound wave propagation varies across different mediums. It is generally fastest in solids, like bone, and slowest in gases or gas-containing & - - - structures, such as the lungs - - = - ad T ↓ - - - G The average propagation speed of sound in tissues & Material speed (m/sec) Air L 330 Fat L 1450 Water W 1480 Soft tissue L 1540 Bone - 4100 Sound Wave Parameters: Amplitude & The amplitude of a sound is created by the number of molecules displaced by a vibration · j g To Amplitude is indicative of the strength or intensity of a sound wave. -a Amplitude is typically measured in units of pressure, such as Mega Pascals (MPa). - - Sound Wave Parameters: Power & O M - : ② Power is the rate at which work is performed or energy is transferred. - In ultrasound, power refers to the generation of ultrasound waves by the - transducer and their propagation through tissues. These waves carry mechanical - energy that facilitates the displacement of particles within the medium. g -I The higher the power, the greater the wave's capacity to perform this work of displacing particles Sist Sound Wave Parameters: Power O The standard unit of power is the Watt (W). > Power in diagnostic ultrasound is commonly expressed in - - milliwatts (mW). One milliwatt equates to one-thousandth of a Watt, meaning there are-1,000 milliwatts in a single Watt. ↳ & 16 %I d z Sound Wave Parameters: Intensity ~ Intensity (I) is the rate at which energy passes through a unit area. It is equal to the - power in a wave divided by the area (A) over which the power is spread. I Ultrasound is generated by transducers in the form of beams. Beam area is expressed in centimeters squared (cm2). Chil - 6 Intensity units include milliwatts per centimeter squared (mW/cm2) and watts per - centimeter squared (W/cm2). wham mini An increase - in area decreases intensity because power is less concentrated. A - - - decrease in area (focusing) increases intensity because power is more concentrated. - I - An ultrasound pulse is weakened (reduction of amplitude) as it travels through a medium (in this case from left to right). This weakening is - - called attenuation. - A, Amplitude is the maximum amount of variation that occurs in an acoustic variable (pressure, in this case). In this figure, the amplitude is 2 megapascals (Mpa). ud B, Intensity is the power in a sound wave divided by the area over which the power is spread (the beam area). - Pulsed wave - A pulse, by definition, must have a distinct beginning and end - Pulsed ultrasound comprises two main components: 1. The Cycle: This is the "on" or "transmit" time during which the ultrasound wave is - & > emitted. & - 2. The Dead Time: Also known as the "off" or "receive" time, this is the period during - - - which the transducer awaits the return of the echoes. Pulsed transducers are designed to generate multiple, sequential, short pulses, allowing for the simultaneous - - - use of the same crystal or group of crystals for both sound transmission and echo reception. - => & - Pulsed wave In pulsed mode, a singular crystal or a specific group of crystals is used for both the transmission of sound and the reception of echoes. -x - S - = - A pulsed transducer emits ultrasound waves that span a variety of - - frequencies. This spectrum is referred to as the 'frequency bandwidth. => - # Pulsed wave transducers are responsible for generating all types of ultrasound diagnostic images, including both-real-time and static. - - & E95 : Y , > - , - Contentious wave (CW) Cardios15 96 popleur Continuous wave (CW) ultrasound is It is important to note that predominantly employed continuous wave sound is in echocardiography for incapable of creating acquiring CW Doppler anatomic images information. Pulsed Repetition Frequency (PRF) RRE Pulse Repetition Frequency (PRF) refers to the number of sound pulses generated by the transducer per second. - The determination of PRF is attributed to the sound source and can be - = adjusted by the sonographer. There is an inverse relationship between imaging depth and PRF, meaning as - & imaging depth increases, PRF decreases. - - F The sonographer can change PRF, and the adjustment is particularly relevant to - achieve optimal imaging depth. - T 1 + 25 - 493x + p 6755 ↑ & is I + 7 c p()) & g /) increase dep - PRE- decrease > PRP -increase PRP-decreas the time decrees Pulse repetition period (PRP) PRP Pulse-repetition period (PRP) refers to the time - from the beginning of one pulse to the beginning & - of the next one - & PRP The pulse-repetition period decreases while PRF & - increases because, when more pulses occur in a - second, the time between them decreases. Pulse Repetition Period (PRP) 39 Pulse Repetition Period (PRP) is the reciprocal of Pulse Repetition Frequency (PRF), expressed in milliseconds or any unit of time. un ~ PRP = 1 / PRF - The determination of&PRP is influenced by the sound source, and it can be => - adjusted by the operator. - In clinical imaging, typical values for PRP range from 100 microseconds to 1 - millisecond. - => ⑤ - - Pulse duration ⑧ Pulse duration (PD) is the time that it takes for one pulse to occur - PD is equal to the period (the time for one cycle) times the number of cycles in the - pulse (n) and is expressed in microseconds. - Sonographic pulses are typically two or three cycles long. - ⑤ 2 - Doppler pulses are typically 5 to 30 cycles long. E PD(μs) = n ×T(μs) Pulse duration PD decreases if the number of cycles in a pulse is decreased or if the frequency is increased (reducing the period). - Consider a 3.5 MHz transducer - with a 5-cycle pulse. Calculate the Pulse pulse duration: ⑳ 5 duration 14 3. I uxT O O 5 PO = Ms - Answer Period = 1 ÷ frequency = 1 ÷ 3.5 MHz =0.28 µs PD = 0.28 µs × 5 = 1.4 µs. Duty factor (DF) D nf The duty factor is the percentage of time that the ultrasound system transmits sound. = - DF is the fraction of the PRP that the sound is on. The remainder of the time to the next pulse is the * - - listening time for reception of echoes that will form a scan line on the instrument display. - DF = Pulse duration/pulse repetition period - Continuous wave ultrasound is on 100% of the time. - Duty factor (DF) * Typical DFs for sonography are in the range of 0.1% to - 1.0%. For Doppler ultrasound, because of longer pulse - - - durations, the range of typical DFs is 0.5% to 5.0%. - - Changed by Sonographer? Yes, the sonographer can adjust it when changing imaging depth. You are performing an ultrasound examination with a pulsed ultrasound system. The pulse - duration is 2 microseconds (µs), Duty factor - and the pulse repetition period is 1 X 1600 · (DF) - millisecond (ms). Calculate the Duty Factor (DF) · for this ultrasound examination. L - O - & G Answer Pulse Duration = 2 µs Pulse Repetition Period = 1 ms = 1000 µs DF = (2 µs) / (1000 µs) DF = 0.002 So, the DF for this ultrasound examination is 0.002 or 0.2%. This means that the ultrasound system is actively transmitting sound waves for only 0.2% of the time during the examination. Spatial pulse length (SPL) * SPL is the length of a pulse from front to back SPL is equal to the length of each cycle times the number of cycles in the pulse. SPL(mm) = n × wavelength (mm) Because wavelength decreases with increasing frequency, SPL decreases with increasing - frequency - SPL determines axial resolution m Pe Spatial pulse length (SPL) A, Spatial pulse length (SPL) is the length of space over which a pulse occurs. SPL is equal to wavelength multiplied by the number of cycles in the pulse. In this figure, the wavelength is 0.5 mm, there are two cycles in each pulse, PD TXN = and the SPL is 0.5 × 2, or 1 mm. * T= Ultrasound Interaction with Tissue Attenuation in Ultrasound O genti Attenuation refers to the progressive reduction in amplitude or intensity of ultrasound waves as they travel through a medium. This effect is due to the absorption of sound energy, the reflection and scattering of sound waves. apate win Factors Influencing Attenuation: 1. Path Length: Longer travel distances result in more attenuation 2. Frequency: Higher frequencies undergo more attenuation Attenuation in Ultrasound 00 Attenuation is measured in decibels (dB). O Attenuation Coefficient (dB/cm) = Half the frequency (MHz) An average loss of 0.5 dB/cm per MHz frequency. Equation: Total attenuation (dB) = Attenuation Coefficient (dB/cm) x Path length (cm) dB = dB(m) e XPath /34]55546 - >-Y, % , Factors Contributing to Attenuation Attenuation of ultrasound waves is influenced by three primary processes: - 1. Absorption: The predominant mechanism of attenuation where ultrasound energy is converted to heat within the tissue. 2. Reflection: The redirection of part of the ultrasound wave back towards the transducer when it encounters a boundary between different media. - 3. Scattering: The spreading out of the sound wave in various directions, & often occurring with rough or irregular surfaces. - & Levels of Ultrasound Attenuation -N - High Attenuation (Materials like bone, air, scars, fibrous - - - - tissue, fat, stones, calcifications, metal, and plastic.) us Moderate Attenuation (Typical of organ parenchyma.) & Low Attenuation (Fluids exhibit less attenuation - compared to other tissues.) - 559559 / Acoustic impedance mismatch JS - - Acoustic impedance (Z) quantifies tissue stiffness and elasticity, determined by the product of tissue density (ρ) and the speed of sound (C). - sus Sa s & Formula: Z = ρ x C, where8 ρ is measured in kg/m³ and C in m/s. Units for Z are - expressed in rayls (1 rayl = 1 kg/(m²·s)). The transmission and reflection of ultrasound at tissue interfaces are influenced -- by acoustic impedance disparities. Similar impedance values across tissues result in greater transmission; substantial differences cause increased reflection - & - - & -- - - - Acoustic impedance Using the values for density and Density Speed of the speed of ultrasound given in Medium Ultrasound (kg/m3) the table, calculate the acoustic (m/s) Air 1.3 330 impedance/ calculate the acoustic Water 1000 1500 impedance mismatch of muscle Blood 1060 1570 and bone. Fat 925 1450 C 3+ & Muscle 1075 1590 2 : - Bone 1400 4080 Barium titanate 5600 5500 (transducer material) Acoustic impedance * Calculate the intensity reflection coefficient Speed of of ultrasound when transitioning from Density Ultrasound Medium (kg/m3) muscle to bone, and provide an (m/s) Air 1.3 330 interpretation of your findings Water 1000 1500 a = (Z2−Z1)2 ÷ (Z1+Z2)2 Blood 1060 1570 = (5712000 – 1709250)2 ÷ (1709250 + 5712000)2 X Fat 925 1450 = (1.6022008 × 10^13) ÷ (5.5074952 × 10^13) S Muscle 1075 1590 & 6 Bone 1400 4080 = 0.029 = 2.9% # Barium titanate (transducer material) 5600 5500 Incident Sound in Ultrasound - - - - Incident sound refers to the ultrasound beam before it interacts with a boundary between two different media. Types of Incidence: Normal (perpendicular) and oblique. Normal Incidence occurs when the ultrasound path is perpendicular to the boundary. & Incident Sound in Ultrasound Oblique Incidence occurs when the ultrasound beam strikes the interface between two media at an angle other than 90°. Incident Angle: The angle at which the ultrasound enters the medium. The degree of reflection and refraction is influenced by the acoustic impedance differences of the two media 70 & Factors Contributing to Attenuation: Absorption Gr Absorption is conversion of sound energy into heat within a medium. : Factors Affecting Absorption: - 1. Relaxation Time: Slower molecular relaxation requires more energy, leading to - - - increased absorption. 2. Frequency: - Higher frequencies produce more heat due to friction and molecular - motion, enhancing absorption - - Factors Contributing to Attenuation: Reflection Process Reflection is the redirection of part of the ultrasound wave back - - towards the source. - More likely occurs when the boundary's dimension is significant relative to the wavelength (large, flat, and smooth boundary). - - - The degree of reflection is influenced by the acoustic impedance -- difference between the two media. Types of Ultrasound Reflection Occurs at large and smooth interfaces. 1. Specular - - When the incident angle is perpendicular, and the Reflection interface is larger than the beam's width, partial reflection back to the source occurs. 2. Non-Specular Happens with rough or irregular surfaces. - - - (Diffuse) # The ultrasound beam is scattered in multiple - directions, leading to a range of reflection angles Reflection - D (A) Illustration of specular versus diffuse reflection. The smooth surface in specular reflection results in more return of the reflected sound waves to the transducer (green arrows) creating a more hyperechoic (brighter) image. The less uniform tissue in diffuse reflection results in less return of the reflected sound waves and a more hypoechoic (darker) image. (B) Sonogram showing the appearance of specular reflection. Note that the large smooth surface of the bone (yellow arrows) leads to a bright signal due to the significant impedance difference between it and the surrounding tissue. (C) Sonogram showing the appearance of more diffuse reflection in muscle tissue. Note that the smaller differences in acoustic impedance reflect various shades of gray rather than the bright signal noted with the interface of bone. Factors Contributing to Attenuation: scattering Process Scattering refers to the way sound waves spread out in different directions when they encounter tissues with irregular surfaces or interfaces similar in size - to the wavelength of the sound. This phenomenon leads to the reflection of ultrasound waves back to the transducer, which is known as backscatter. The image artifacts produced by backscatter are called 'speckle.’ - - - - & Scattering intensity increases when the tissue interface size is comparable to or - smaller than the wavelength of the incident ultrasound wave. => => Factors Contributing to Attenuation: Refraction Process Refraction is the change in direction of a sound wave as it passes across a boundary between two different media at an oblique angle, not perpendicular to the boundary. Refraction only occurs if the sound wave hits the boundary at an angle that is not 90 - degrees (Oblique Incidence). The speed of sound must differ between the two media; if the speeds are the same, - no refraction will occur. - Refraction occurs due to the difference in propagation speeds of sound in different media, as defined by Snell's Law. - Factors Contributing to Attenuation: Refraction Process & Factors Contributing to Attenuation: Refraction Process The direction of the refracted beam depends on the speed of sound - in the second medium relative to the first medium. & E end & 939