Free Energy and Equilibrium (PDF)
Document Details
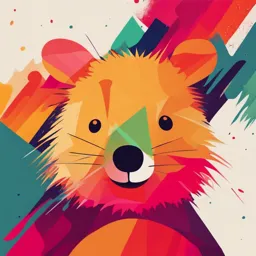
Uploaded by FragrantSpessartine
null
Tags
Related
- Soil 240 Chapter 1.1 Chemical Equilibria and Thermodynamics of Reactions PDF
- DAT/OAT General Chemistry Outlines PDF
- AIPMT 2012 Chemistry Past Paper PDF
- Chemical Equilibrium BSc 1st Sem PDF
- Energetics of Chemical Processes (10 L) - University of Colombo - First Semester, May 2024 PDF
- Chemical Equilibrium and Redox Processes Part 1 PDF
Summary
This document discusses free energy and equilibrium concepts in chemistry, including enthalpy and entropy considerations, and provides examples and solutions.
Full Transcript
Equilibrium and Energy Equilibrium Energy and Thermodynamics Gibbs Free Energy and Equilibrium: Points about Gibbs Free Energy and Equilibrium: 1. True at Equilibrium: 2. If Not at Equilibrium: Question 1) G° for the isomerization of glucose-6-phosphate (G6P) to fructose6-phosphate (F6P)...
Equilibrium and Energy Equilibrium Energy and Thermodynamics Gibbs Free Energy and Equilibrium: Points about Gibbs Free Energy and Equilibrium: 1. True at Equilibrium: 2. If Not at Equilibrium: Question 1) G° for the isomerization of glucose-6-phosphate (G6P) to fructose6-phosphate (F6P) is 2.1 kJ mol^-1. What concentrations of G6P and F6P will be present at equilibrium at 25 °C starting with 0.1 M G6P? Solution 1) Question 2) The concentration of a solution of G3P was initially 0.05 M. Isomerase was added. After the mixture came to equilibrium at 25 °C, the concentration of G3P was 0.002 M. Calculate ΔG° for the reaction. What are the implications of the values of ΔG° and Keq for the reaction as given above? Solution 2) Energy and equilibrium of systems, so far… Criterion for the direction of spontaneous change (Gibbs) Free energy Enthalpy vs. Entropy In the context of the analysis of Mg²⁺ ions using complexation with EDTA, the concepts of enthalpy and entropy play important roles in determining the spontaneity of the reaction and how it proceeds. Process Overview: Magnesium ions (Mg²⁺) in an aqueous solution can form a stable complex with EDTA (ethylenediaminetetraacetic acid) when it binds to the Mg²⁺, displacing water molecules and forming a coordination complex. The EDTA tetra sodium salt is used to bind to Mg²⁺ ions. The process is often used in pharmaceutical and biochemical analysis to determine the concentration of Mg²⁺ in solution. Enthalpy (ΔH) Considerations: The reaction involves a change in enthalpy because it results in the formation of new bonds between Mg²⁺ and EDTA, and the solvation energy of Mg²⁺ in water is altered when it binds to EDTA. o Breaking of solvation bonds (water molecules surrounding Mg²⁺) is an endothermic process, as it requires energy input. o Formation of new bonds between Mg²⁺ and EDTA may release energy, making the overall process exothermic or endothermic depending on the magnitude of these interactions. Entropy (ΔS) Considerations: The entropy change (ΔS) is critical for determining whether the process is spontaneous at a given temperature. o Increase in disorder (positive ΔS): When Mg²⁺ is complexed with EDTA, the water molecules previously surrounding Mg²⁺ become less organized as they are displaced, which generally leads to an increase in entropy. o Formation of a complex: The complexation with EDTA might also lead to an increase in the number of particles (e.g., the breakdown of solvated water molecules into individual ions and EDTA), leading to an overall increase in entropy of the system. Combined Effect (ΔG = ΔH - TΔS): For the complexation to be spontaneous (i.e., go essentially to completion), the change in Gibbs free energy (ΔG) must be negative. This means that: o The enthalpy change (ΔH) must favor the formation of the complex (e.g., negative or exothermic reaction), o The entropy change (ΔS) must also favor the complexation (e.g., positive ΔS due to the release of ordered water molecules). In this reaction, the process is driven by both the enthalpy (exothermic nature of bond formation) and the entropy (increased disorder due to displacement of water molecules and complex formation). Therefore, the reaction moves essentially to completion, forming the stable Mg-EDTA complex. Conclusion: In summary, the enthalpy and entropy changes during the complexation of Mg²⁺ with EDTA are important for determining the spontaneity of the reaction. The process is driven by both: Enthalpy: Heat released from bond formation, Entropy: Increased disorder from the displacement of water molecules and formation of the complex. This results in a spontaneous reaction where the complexation of Mg²⁺ by EDTA goes essentially to completion. In this analysis of the complexation of Mg²⁺ with EDTA, you are essentially examining how enthalpy and entropy contribute to the overall Gibbs free energy (ΔG), which determines the spontaneity of the process. Let's break down the process: 1. Enthalpy (ΔH): ΔH is small and positive: This suggests that the process is not strongly driven by heat release (it's not highly exothermic). In terms of molecule-level processes, this could mean that the formation of bonds between Mg²⁺ and EDTA or the displacement of water molecules from the Mg²⁺ ion is not strongly exothermic. The breaking of solvation bonds between Mg²⁺ and water requires energy input (endothermic), but this is likely compensated by the bond formation between Mg²⁺ and EDTA. 2. Entropy (ΔS): ΔS is significant and positive: The overall disorder of the system increases as a result of the process. Specifically, the release of five H₂O molecules and two NaCl molecules into the solution increases the entropy. o The water molecules that were previously structured around the Mg²⁺ ion are now free to move, thus increasing the disorder (positive ΔS). o The formation of NaCl (from Na⁺ and Cl⁻) also adds to the increase in the number of components in the system, which increases the disorder (entropy). 3. Gibbs Free Energy (ΔG): ΔG is negative, meaning the process is spontaneous as written (left to right). Despite ΔH being small and positive, the significant positive ΔS results in a negative ΔG because the TΔS term dominates. This ensures that the overall process is spontaneous at a given temperature (as ΔG = ΔH - TΔS). Conclusion: Thermodynamic driving forces: The entropy change (ΔS) is the main driving force for the process, making it spontaneous. Molecule-level processes: The displacement of water molecules (disorder increases) and the formation of NaCl contribute to the significant positive ΔS. Overall process: Even though enthalpy (ΔH) is slightly positive (endothermic), the large increase in entropy (ΔS) results in a negative ΔG, driving the spontaneous complexation of Mg²⁺ with EDTA. This analysis shows how the entropy change dominates and drives the spontaneity of the process, despite the positive enthalpy change. Standard states of free energies The concept of standard states for free energies refers to the reference conditions under which the free energy values are measured. Here’s a more detailed breakdown: 1. Standard Free Energy (G°): G° refers to the Gibbs free energy of a pure substance under standard conditions: o A pressure of 1 atmosphere (atm). o A temperature of 298 K (25 °C). o For substances in their standard state (the most stable form at 1 atm and 298 K). Example: o G° of H₂O (liquid) at 298 K is measured under standard conditions. o G° of CO₂ (gas) at 298 K is also under standard conditions. 2. Standard Free Energy Change (ΔG°): ΔG° is the change in Gibbs free energy for a reaction or process when: o The reaction proceeds with 1 mole of reactants in their standard states being converted into 1 mole of products in their standard states. ΔG° can be calculated for a reaction using the standard free energies of formation (ΔG°f) of the reactants and products: Where: o ΔG°f is the standard free energy of formation (the free energy change when 1 mole of a compound is formed from its elements in their standard states). 3. Interpretation: ΔG° < 0: The reaction is spontaneous under standard conditions. ΔG° > 0: The reaction is non-spontaneous under standard conditions. ΔG° = 0: The system is at equilibrium under standard conditions. Example: In summary: G° is the free energy of a pure substance at standard conditions. ΔG° refers to the change in free energy during a reaction, and its sign determines the spontaneity of the reaction under standard conditions. Using ΔG values, example This positive value indicates that the reaction is non-spontaneous under standard conditions. Question 1) ATP → ADP + Pi G° = −30.5 kJ mol^−1 (from thermal analysis) H° = −20.1 kJ mol^−1 Calculate S° = (all at 37 °C) Comment on the significance of the values of G, H and S. Solution 1) Question 2) H° and S° for the unfolding of a protein are 250.8 kJ mol^−1 and 752 J K^−1 mol^−1 respectively. Above what temperature (in °C) will unfolding of the protein be spontaneous? Comment on the values. Solution 2) This shows that temperature plays a crucial role in determining whether the protein will unfold spontaneously, with higher temperatures favoring the unfolding due to the significant increase in entropy.