Epithelial Tissue & Body Water - PDF
Document Details
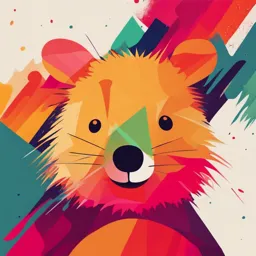
Uploaded by FragrantSpessartine
null
Tags
Summary
This document provides a detailed overview of epithelial tissue, its functions, locations, and characteristics. It describes various types of epithelial tissue found in different parts of the body, such as skin, sweat glands, mouth, lips, lungs, and the gut. The document also outlines the distinguishing features of epithelial tissue, including its avascular nature, rapid reproduction, tight cell packing, and role in defining bodily compartments.
Full Transcript
Epithelial Tissue Function of Epithelial Tissue: Protects: Acts as a barrier to physical damage, harmful substances, and pathogens. Secretes: Secretes substances like mucus, enzymes, and hormones. For example, glandular epithelium forms the secretory tissue in glands....
Epithelial Tissue Function of Epithelial Tissue: Protects: Acts as a barrier to physical damage, harmful substances, and pathogens. Secretes: Secretes substances like mucus, enzymes, and hormones. For example, glandular epithelium forms the secretory tissue in glands. Absorbs: Found in areas like the intestines, where it absorbs nutrients and fluids from the digestive tract. Excretes: Helps in the removal of waste products, as seen in the skin and kidney tubules. Location: Covers body surfaces: The outer layer of the skin (epidermis) is composed of epithelial tissue. Lines body cavities: Epithelial tissue lines organs and cavities such as the lungs, digestive tract, and blood vessels. Glands: Forms the secretory tissue of glands like sweat glands, salivary glands, and endocrine glands. Distinguishing Characteristics: No blood vessels (Avascular): Epithelial tissue does not have its own blood supply and relies on diffusion from nearby tissues for nutrients and oxygen. Reproduces readily: Epithelial cells have a high rate of division and turnover, which is important for repair and regeneration. Tightly packed cells: The cells are closely joined together, forming continuous layers or sheets that protect underlying tissues. Defines boundaries: It forms boundaries that define body compartments, such as the outer layer of skin or the lining of internal organs, helping to separate and protect different bodily regions. Epithelial tissue is essential for many functions, including protection, absorption, and secretion, and it forms the structural basis of many body surfaces and organs. Your examples illustrate the variety and specialization of epithelial cells in different parts of the body, each adapted to meet specific functional needs. Here's a breakdown of how these epithelial cells differ in structure and function across various organs: 1. Skin (Normally Dry) Type of Epithelium: Stratified squamous epithelium. Function: Protects underlying tissues from physical damage, dehydration, and pathogens. The skin's outermost layer (epidermis) is primarily composed of keratinized epithelial cells, providing a tough, waterproof barrier. Characteristics: Dry, keratinized, and resistant to abrasion. Epithelial cells here are constantly being shed and replaced. 2. Sweat Glands Type of Epithelium: Simple cuboidal or columnar epithelium. Function: Secretes sweat onto the skin's surface for temperature regulation. Characteristics: Sweat glands are found in the dermis and have ducts lined with epithelial cells. These cells help secrete water and salts (sweat) through the sweat ducts to the skin's surface. 3. Mouth (Normally Moist) Type of Epithelium: Non-keratinized stratified squamous epithelium. Function: Provides protection against physical stress, while also allowing moisture retention and nutrient absorption. Characteristics: The epithelial cells are moist and more flexible, as the mouth is constantly exposed to moisture from saliva. This tissue is also important for protecting against abrasions during chewing and swallowing. 4. Lips (Interface between Skin and Mouth) Type of Epithelium: Transitional epithelium (where the skin meets the mucous membrane). Function: Acts as a boundary between the dry skin and moist mucous membranes. Lips are more sensitive due to the lack of sweat glands, which makes them more prone to dryness. Characteristics: The transition from keratinized skin epithelium (on the outer lips) to non-keratinized epithelial cells (on the inner side, closer to the mucosal lining of the mouth). This zone is important for the flexibility and sensation of the lips. 5. Lungs (Gas Exchange) Type of Epithelium: Simple squamous epithelium. Function: Facilitates gas exchange (O₂ and CO₂) between the alveoli and blood. Characteristics: The epithelial cells in the lungs are extremely thin (one cell layer) to allow easy diffusion of gases. These cells line the alveoli (air sacs) and are moist to help dissolve gases before diffusion into the bloodstream. 6. Gut (Absorb Nutrients) Type of Epithelium: Simple columnar epithelium (with microvilli). Function: Absorbs nutrients and water from food. Characteristics: The epithelial cells in the intestines have microvilli (finger-like projections) that increase the surface area for absorption. They are specialized for nutrient absorption and secretion, and many contain goblet cells that secrete mucus to protect the lining of the gut. 7. Kidneys (Absorb and Filter) Type of Epithelium: Simple cuboidal or columnar epithelium (in the renal tubules). Function: Filters blood, absorbs nutrients, and regulates water balance. Characteristics: The epithelial cells in the kidneys' nephron (the functional unit) are specialized for absorption and filtration. They line the renal tubules and help filter blood, reabsorb water and ions, and secrete waste products into urine. 8. Blood Vessels (Endothelium) Type of Epithelium: Simple squamous epithelium (endothelium). Function: Lines blood vessels, facilitating smooth blood flow and exchange of gases, nutrients, and waste between the blood and tissues. Characteristics: Endothelial cells are thin and smooth, minimizing friction and facilitating the efficient exchange of substances across the vessel wall. These examples highlight the diversity of epithelial cells in the body. The structure of the epithelial tissue varies based on location and function, demonstrating the body’s ability to adapt to different physiological needs, whether for protection, absorption, secretion, or gas exchange. Epithelial cells form membranes Epithelial cells form membranes that define the boundaries between different body compartments. These membranes can be categorized into two main types based on their structure: stratified and monolayers. 1. Types of Epithelial Membranes: Stratified Epithelium: Composed of multiple layers of cells stacked on top of each other. Provides additional protection, especially in areas subject to abrasion or mechanical stress. Examples: Skin (epidermis), esophagus, and vagina. Monolayer (Simple Epithelium): Composed of a single layer of cells, allowing for efficient absorption, secretion, and filtration. Examples: Lungs (alveoli), kidneys (nephrons), and blood vessels (endothelium). 2. Key Surface Characteristics: Apical Surface: Exposed to the outside environment or to an internal open space (lumen). Faces air, fluid, or other substances, depending on the organ system. In certain epithelial cells, this surface may have cilia or microvilli to aid in movement (cilia in the respiratory tract) or absorption (microvilli in the intestines). Basolateral Surface: Attached to the underlying connective tissue by the basement membrane. The basement membrane helps anchor the epithelial tissue and separates it from underlying tissues, providing structural support and filtering functions. The basolateral surface interacts with connective tissue, which provides nutrients and regulates waste removal. 3. Specialized Junctions: Tight Junctions: These are protein complexes that seal the gaps between adjacent epithelial cells, preventing leakage of extracellular fluid and ensuring the selective permeability of the membrane. 4. Keratinized vs. Non-Keratinized Epithelia: Keratinized Epithelium: Dead and dry: In keratinized epithelium, cells on the surface become filled with keratin (a tough, fibrous protein) and die, forming a dry, protective barrier. Found in areas exposed to friction and mechanical stress. Example: Skin (epidermis). Non-Keratinized Epithelium: Alive and moist: The surface cells are not filled with keratin, keeping them moist and functional. Found in areas that require flexibility and moisture, typically in areas exposed to less friction. Example: Inside the mouth, lining of the lungs, and esophagus. Summary of Epithelial Cell Functions: Protection: Stratified and keratinized epithelia protect against abrasion, dehydration, and pathogen invasion (e.g., skin). Absorption: Simple epithelium in the intestines and kidneys is specialized for nutrient and ion absorption. Secretion: Epithelial cells also line glands that secrete substances like enzymes, mucus, and hormones (e.g., sweat glands, mucosal lining). Excretion: Certain epithelia facilitate the excretion of waste products, as seen in the renal system. These epithelial membranes play a critical role in maintaining the structural integrity and function of organs and body systems, facilitating the selective exchange of substances, and providing barriers to protect the body from harmful environmental factors. ★ The glands (which secrete into or out of the body) The pancreas and gonads (testes and ovaries) have both endocrine and exocrine functions, playing crucial roles in the body’s regulation and reproductive systems. Here's a breakdown of their dual roles: Pancreas: Endocrine Function: o The pancreas releases insulin and other hormones like glucagon into the bloodstream. These hormones regulate blood glucose levels and play key roles in metabolism. o Insulin helps lower blood sugar by promoting the uptake of glucose into cells. o Glucagon raises blood sugar by stimulating the liver to release glucose. Exocrine Function: o The pancreas also secretes digestive enzymes into the gastrointestinal tract (GIT) via the pancreatic duct. These enzymes (such as amylase, lipase, and proteases) aid in the breakdown of carbohydrates, fats, and proteins, respectively, facilitating digestion. Testes and Ovaries (Gonads): Endocrine Function: o The testes secrete testosterone, which is responsible for the development of male secondary sexual characteristics and plays a role in sperm production. o The ovaries secrete estrogen and progesterone, hormones that regulate the menstrual cycle, support pregnancy, and contribute to the development of female secondary sexual characteristics. Exocrine Function: o Testes produce sperm through spermatogenesis, releasing it into the male reproductive tract (via the seminiferous tubules, epididymis, and vas deferens). o Ovaries release ova (eggs) during ovulation, which then travel through the fallopian tubes for potential fertilization. Summary: Both the pancreas and gonads serve dual functions, as they secrete both hormones into the blood (endocrine) and produce substances that are released into ducts or external areas (exocrine). This integration of both functions is essential for maintaining metabolic balance, reproductive health, and overall homeostasis. Inside versus Outside The distinction between the inside and outside of the body refers to how substances are transported or processed in the body. Here's a more detailed breakdown: Inside the Body: Blood or Lymph System The blood and lymph systems are part of the internal circulatory system that transport nutrients, gases, hormones, waste products, and immune cells throughout the body. The blood circulates through blood vessels (arteries, veins, and capillaries) and carries oxygen, nutrients, and waste products to and from cells. The lymphatic system transports lymph (a fluid containing white blood cells and waste products) and is crucial for immune function and the removal of excess fluid from tissues. The inside of the body is essentially the spaces and organs that are perfused by blood or lymph, such as tissues, organs, and blood vessels. Outside the Body: Skin, Lung, Kidney Tubules, Digestive Tract The outside refers to the external environment of the body and includes systems that interface directly with the outside world: o Skin: The body's protective barrier that prevents pathogens from entering and regulates water loss. o Lungs: Facilitate the exchange of gases (oxygen and carbon dioxide) with the external environment through the respiratory system. o Kidney Tubules: Involved in filtering blood and excreting waste as urine. While blood enters the kidney, the waste products are processed and excreted from the body, effectively moving from the internal system to the external. o Digestive Tract: The gastrointestinal system, which absorbs nutrients from food into the bloodstream and excretes waste products (in the form of feces) out of the body. Interfaces between Inside and Outside: Epithelial tissues form the barrier between the inside and outside. For example: o Skin epithelium separates the external environment from internal body systems. o Mucosal linings in the lungs, digestive tract, and kidneys serve as interfaces between external materials and internal systems. These systems allow for the intake of essential substances (like oxygen and nutrients) and the elimination of waste (like CO2 and urea), helping the body maintain homeostasis. Body water ★ Body water volume and composition The total body water (TBW) of a 70 kg human is approximately 42 liters (L), which makes up about 55-60% of body mass. This volume is equivalent to 74 pints of water! This percentage can vary depending on factors such as age, gender, and body composition. Typically: Adult males have a higher percentage of body water (around 60%) due to more lean muscle mass, which holds more water. Adult females tend to have slightly less body water (around 55%) because they generally have a higher body fat percentage, which contains less water. Body Water Distribution: 1. Intracellular Fluid (ICF): The majority of body water (about 2/3) is inside cells, where it plays crucial roles in cellular function. 2. Extracellular Fluid (ECF): The remaining 1/3 is outside the cells and includes: o Interstitial Fluid: The fluid between cells. o Plasma: The liquid part of blood. o Other body fluids: Lymph, synovial fluid, etc. Importance of Body Water: Water is essential for chemical reactions, nutrient transport, temperature regulation, and waste elimination. Maintaining the balance of water in the body is vital for proper homeostasis and organ function. If you're conducting an experiment to calculate body water (as mentioned in your notes), it likely involves measurements such as body weight, fluid compartments, and possibly bioimpedance or other techniques to estimate total body water. In a 70 kg human, the total body water (TBW) is approximately 42 liters, which constitutes 55-60% of body mass. Here's how this water is distributed: 1. Intracellular Fluid (ICF): About 2/3 of the total body water is located inside cells. So, for a 42 L total body water, approximately 28 L is within cells. o There are ~50 trillion cells (or 50 million million cells) in the body. o On average, each cell contains water, which is vital for its metabolic processes. 2. Extracellular Fluid (ECF): The remaining 1/3 of body water is found outside cells, which includes: o Interstitial Fluid: The fluid between cells. o Plasma: The liquid part of blood, which accounts for about 20% of extracellular fluid. o Other Fluids: These include lymph, synovial fluid, and fluids in various body cavities and organs. So, about 14 L (1/3 of 42 L) is outside the cells, mainly in the extracellular space. This distribution is crucial for maintaining homeostasis, as both intracellular and extracellular fluids play key roles in nutrient delivery, waste removal, and maintaining cellular function and fluid balance. ★ Composition of Body Fluids and Terminology 1. Total Extracellular Fluid: o Total volume: 14 L o Refers to all the fluid present outside of cells. 2. Major Components of Extracellular Fluid: o Interstitial Fluid: ▪ Definition: The fluid that bathes and surrounds cells, distinct from intracellular fluid. ▪ Volume: 11 L o Plasma: ▪ Definition: The liquid component of blood that circulates within blood vessels, transporting substances to cells and tissues. ▪ Volume: 3 L 3. Distribution of Body Water: o 33% of the total body water surrounds the approximately 50 trillion cells in the body as extracellular fluid. Terminology Breakdown Extra: Outside Inter: Between Intra: Within This foundational understanding of body fluid distribution is essential for grasping key physiological concepts. ★ Body Water Composition: Intracellular Fluid (ICF) vs. Extracellular Fluid (ECF) Total Body Water is divided into: o Intracellular Fluid (ICF): 28 L o Extracellular Fluid (ECF): 14 L Key Characteristics: While ICF and ECF have different solutes and concentrations, they share the same osmolarity (~280 mOsm). This equal osmolarity prevents net water movement across cell membranes, maintaining fluid balance. Summary ECF (e.g., plasma) is high in Na⁺ and Ca²⁺, while ICF (e.g., muscle cells) is high in K ⁺. The osmolarity remains similar between both fluid compartments despite the differences in solute concentrations, ensuring that no net water movement occurs between ICF and ECF under normal conditions. Daily Balance of Water in the Body Daily Water Loss (on a typical day): Kidneys (urine): 1.5 L Lungs (via breathing): 0.3 L Skin/Sweat: 0.6 L Feces: 0.1 L Total: 2.5 L Key Points: Water Intake = Water Loss: To maintain balance and prevent dehydration, daily water intake must match daily loss. Mathematical Estimation: Theoretically, if the body loses 2.5 L of water per day, one could survive for almost 17 days without water intake (42.5 L total body water ÷ 2.5 L/day). Physiological Reality: In practice, surviving more than 3 days without water is unlikely, as severe dehydration can be fatal. Factors Affecting Water Loss Exercise External temperature Intake of coffee, alcohol, or salt Medical conditions (diseases) These factors can significantly increase the rate of water loss, making it crucial to adjust water intake accordingly. How Does the Body Maintain Water Balance? The human body needs to regulate its water levels to sustain approximately 42 liters of total body water. This involves: 1. Water Intake: o Through drinking fluids and consuming food that contains water. 2. Water Excretion: o Primarily through kidneys (urine), but also via lungs, skin, and feces. 3. Monitoring and Regulation: o The body uses mechanisms such as thirst and hormonal regulation (e.g., ADH - antidiuretic hormone) to control water intake and adjust urine output to maintain a stable internal environment. Understanding this balance is crucial for maintaining optimal hydration, especially under varying conditions like physical activity, temperature changes, and dietary factors. Maintaining the body’s water balance is vital for health and survival, especially since factors like exercise, external temperature, and dietary intake (coffee, alcohol, salt) can significantly affect water loss. Here’s how the body manages this balance: How the Body Takes In Water: 1. Ingestion (Primary Source): o Drinking Fluids: Water, juices, and other beverages are the main source of water intake. o Foods: Many foods, especially fruits and vegetables (e.g., watermelon, cucumbers), contain high water content. 2. Metabolic Water Production: o Water is produced as a byproduct of metabolism, especially during the breakdown of carbohydrates, fats, and proteins. However, this source contributes only a small amount to total intake. How the Body Gets Rid of Excess Water: 1. Kidneys (Urine): o The kidneys are the primary regulators of water balance. They adjust the volume and concentration of urine based on hydration status. o When excess water is present, the kidneys increase urine output to prevent fluid overload. 2. Lungs (Breath): o Water is lost through respiration as water vapor when we exhale. o The rate of water loss via breathing increases during exercise or in cold, dry environments. 3. Skin (Sweating): o Sweat helps cool the body, especially during physical activity or high temperatures. o Sweat rates can increase dramatically during exercise, hot weather, or with fever, leading to significant water loss. 4. Feces: o A small amount of water is excreted with stool. However, conditions like diarrhea can cause a dramatic increase in water loss, leading to dehydration. How the Body Monitors and Regulates Water Balance: 1. Thirst Mechanism: o The brain’s hypothalamus detects changes in blood osmolarity (concentration of solutes). o When osmolarity increases (indicating dehydration), it triggers thirst, prompting fluid intake. 2. Hormonal Regulation: o Antidiuretic Hormone (ADH): Released by the pituitary gland, ADH signals the kidneys to reabsorb water, reducing urine output. o Aldosterone: This hormone helps regulate sodium and water balance, particularly in response to low blood volume or blood pressure. o Natriuretic Peptides: These hormones promote water and sodium excretion when blood volume is too high. 3. Sweating and Thermoregulation: o The body adjusts sweat production in response to changes in body temperature and hydration levels to prevent overheating and dehydration. Summary To maintain the body’s total water content of approximately 42 liters, the body relies on a dynamic balance between intake and excretion, regulated by a complex system involving the kidneys, hormones, and brain. Understanding these processes helps us manage hydration, especially under varying conditions like intense exercise, hot climates, or dietary changes. ★ How does H2O cross epithelia and enter body? Water (H₂O) crosses epithelial barriers and enters the body through two primary processes: 1. Absorption in the Gut When you drink water, it passes through the digestive tract and is absorbed in the small intestine (primarily) and, to a lesser extent, in the large intestine. Epithelial cells lining the intestines allow water to move into the bloodstream via osmosis, driven by differences in solute concentrations between the intestinal lumen and blood vessels. This absorption process ensures that water enters the circulatory system, where it can be distributed to cells and tissues throughout the body. 2. Reabsorption in the Kidneys Once water is in the bloodstream, it is filtered by the kidneys to remove waste products. During this filtration process, most of the water is reabsorbed into the blood to maintain fluid balance. The nephrons (functional units of the kidneys) adjust water reabsorption based on the body's needs, controlled by hormones such as antidiuretic hormone (ADH). This allows the body to retain water when dehydrated or excrete excess water when hydrated. Key Points on Water Transport Across Epithelia: Water moves primarily through osmosis, following the movement of solutes like sodium and glucose. Aquaporins, specialized water channels in cell membranes, facilitate rapid water transport across epithelial cells in both the intestines and kidneys. Through these mechanisms, the body efficiently regulates water intake, absorption, and reabsorption to maintain proper hydration and electrolyte balance. ★ How does H2O cross epithelia and enter body? Water (H₂O) crosses epithelial barriers and leaves the body through several mechanisms, which are crucial for regulating fluid balance and maintaining homeostasis: 1. Secretion & Evaporation: Lungs & Skin Lungs: Water is lost as water vapor during exhalation. This process is part of normal breathing but can increase with heavy breathing during exercise or in cold, dry environments. Skin: o Sweating: The sweat glands secrete water onto the skin's surface, which evaporates to cool the body. This process can be increased by heat, exercise, or fever. o Insensible Water Loss: Even without visible sweating, a small amount of water is lost continuously through the skin via trans-epidermal evaporation. 2. Secretion and/or Lack of Absorption in the Gut Diarrhea: If the intestines fail to properly absorb water, or if there is excessive secretion due to infection (bacterial, viral, or parasitic), large volumes of water are lost in the form of diarrhea. Microbial Pathogenesis: Certain pathogens (e.g., cholera toxin) can increase secretion of water and electrolytes into the intestinal lumen, leading to severe dehydration. 3. Filtration/Secretion and/or Lack of Reabsorption in the Kidneys Kidneys are responsible for filtering blood and excreting excess water and waste as urine. Water leaves the body if not reabsorbed by the nephrons: o Filtration: Water is filtered out of the blood in the glomeruli. o Secretion: In some cases, additional water is secreted into the renal tubules. o Lack of Reabsorption: When the body needs to eliminate excess water, the kidneys reduce water reabsorption, increasing urine output. Conditions like diabetes (leading to osmotic diuresis) or kidney dysfunction can result in increased water loss due to impaired reabsorption. Summary of Water Loss Mechanisms These processes ensure that excess water is removed from the body, but they can also lead to dehydration if not properly balanced with adequate water intake. ★ Water can cross epithelia… but it cannot cross a lipid bilayer (so how does it get in and out?? Water (H₂O) can cross epithelial barriers efficiently despite being unable to pass directly through the lipid bilayer due to its polar nature. Here’s how water manages to enter and exit cells and tissues: How Water Crosses Epithelial Barriers: 1. Filtration of Blood in the Kidneys o Glomerulus: In the kidneys, blood is filtered through the glomerulus, a network of tiny capillaries with gaps (fenestrations) between endothelial cells. o These gaps allow water and small molecules to pass freely into the Bowman's capsule, while larger molecules (like proteins) remain in the bloodstream. o This filtration process enables the kidneys to rapidly filter large volumes of water and waste products. 2. Reabsorption and Secretion in Other Epithelial Cells o In other parts of the kidney (like the renal tubules) and the intestines, water is reabsorbed or secreted through specialized epithelial cells. o These cells utilize transport proteins to move water in and out of cells efficiently. 3. Transport Proteins and Water Channels o Aquaporins: To bypass the lipid bilayer, water relies on aquaporins, which are specialized transport proteins that form pores in cell membranes. o Aquaporins facilitate the rapid movement of water molecules across cell membranes, allowing cells to adjust water balance quickly. o These proteins are especially important in tissues where water movement is crucial, such as the kidneys, lungs, and intestines. Key Points: Lipid Bilayer Barrier: The lipid bilayer of cell membranes is hydrophobic and does not allow water to pass directly due to water's polarity. Aquaporins: These water channels are essential for allowing water to cross the membrane efficiently. Fenestrations in the Glomerulus: Water can also cross epithelia in the kidneys through physical gaps between cells, enabling filtration. By utilizing a combination of physical gaps (glomerulus) and protein channels (aquaporins), the body ensures that water can be efficiently filtered, reabsorbed, and secreted as needed to maintain fluid balance and homeostasis. Filtration in the Kidneys: Filtration is one of the key processes by which the kidneys maintain fluid and electrolyte balance. It is crucial for removing waste products from the blood while retaining essential substances, like water and useful solutes, for the body's needs. Key Points on Filtration in the Kidneys: 1. Continual Filtration of Plasma: o The kidneys continuously filter plasma (the liquid part of blood) as it passes through the glomerulus, a network of capillaries in the renal corpuscle. o Blood enters the glomerulus via the afferent arteriole and exits via the efferent arteriole. As blood flows through the glomerulus, water and small solutes are filtered out into the Bowman’s capsule (the initial part of the nephron). 2. What is Filtered? o Water and small solutes (less than 1000 Da, such as glucose, ions, and waste products) pass through the filtration barrier into the Bowman's capsule. o This filtration allows the kidneys to rapidly remove waste, excess salts, and metabolic byproducts from the bloodstream. 3. The Filtration Barrier: o The basement membrane of the glomerulus serves as the primary filter, allowing small molecules to pass while preventing larger molecules, such as proteins (e.g., albumin), from exiting the bloodstream. o The basement membrane has a selective permeability, which is essential for maintaining blood composition and preventing protein loss in the urine. 4. Reabsorption and Excretion: o After filtration, the filtrate (the fluid that has entered the Bowman's capsule) travels through the renal tubules (proximal convoluted tubule, loop of Henle, distal convoluted tubule). o Reabsorption: Water, glucose, and other essential solutes are reabsorbed from the filtrate back into the blood, mainly in the proximal convoluted tubule and loop of Henle. o Excretion: Waste products that are not reabsorbed are excreted as urine. This includes urea, creatinine, and excess ions. This filtration process ensures that waste products are efficiently removed from the bloodstream while maintaining the balance of water and electrolytes necessary for the body's functions. ★ Filtration in the Kidneys: Filtration is one of the two primary functions of the kidneys, along with reabsorption. Filtration accounts for about half of what the kidneys do in maintaining homeostasis and regulating body fluids. The Two Main Functions of the Kidneys: 1. Filtration: o Filtration occurs in the glomerulus, where blood is filtered into the Bowman’s capsule. o The kidneys filter plasma continuously, removing waste products, excess ions, and water, while retaining larger molecules like proteins. o This process allows the kidneys to regulate fluid and electrolyte balance by determining which substances are excreted as urine and which are reabsorbed. 2. Reabsorption: o After the filtrate enters the renal tubules, the kidneys selectively reabsorb useful solutes (such as water, glucose, and salts) back into the bloodstream. o This process ensures that important substances are not lost in urine and maintains the body’s fluid and electrolyte balance. While filtration removes waste and excess substances, reabsorption helps conserve vital components, maintaining homeostasis and fluid balance. Together, these two processes account for the kidneys' essential role in regulating the body's internal environment. The protein mesh in the kidneys refers to the basement membrane and the podocytes in the glomerulus, which form a crucial part of the filtration barrier in the kidney. Key Features of the Protein Mesh in Kidney Filtration: 1. Basement Membrane: o The basement membrane is a gel-like extracellular matrix composed of collagen and glycoproteins. o It forms the primary filtration barrier in the glomerulus, preventing the passage of large molecules (such as proteins) from the blood into the filtrate (urine). o The membrane is negatively charged, which helps repel negatively charged molecules, such as proteins like albumin. 2. Podocytes: o Podocytes are specialized epithelial cells that cover the capillaries in the glomerulus. o These cells have foot-like projections (called pedicels) that interdigitate to form filtration slits. o The slit diaphragm between the foot processes acts as a secondary filtration barrier, preventing large molecules and proteins from being filtered into the Bowman's capsule. 3. Filtration Slits: o These slits between the podocyte foot processes are lined with a protein mesh (slit diaphragm) made of nephrin and other proteins. o The slit diaphragm controls the passage of water, small solutes, and ions, allowing only molecules of a certain size to pass through while blocking larger molecules like proteins. Function of the Protein Mesh in Filtration: This protein mesh ensures that while small solutes (such as water, glucose, and salts) pass through into the filtrate, proteins and blood cells are retained in the bloodstream. This process is essential for maintaining protein homeostasis and preventing proteinuria (excess proteins in the urine), which can be a sign of kidney damage. In summary, the protein mesh formed by the basement membrane and podocytes in the glomerulus plays a critical role in maintaining selective filtration and preventing the loss of important proteins into the urine. ★ Reabsorb most of this water Reabsorption of Water in the Kidneys Reabsorption is the other half of the kidneys' critical role in maintaining homeostasis, following filtration. After blood is filtered in the glomerulus, the kidneys reabsorb most of the water from the filtrate back into the bloodstream, thus conserving water and regulating fluid balance. Key Mechanisms of Reabsorption: 1. Anti-Diuretic Hormone (ADH): o ADH, also known as vasopressin, plays a crucial role in regulating the reabsorption of water in the kidneys. o ADH is secreted by the posterior pituitary gland in response to high blood osmolality (high concentration of solutes in blood) or low blood volume. o It acts on the distal convoluted tubule and collecting ducts in the kidney, increasing their permeability to water. This allows more water to be reabsorbed into the bloodstream, reducing urine volume and concentrating the urine. o ADH works by promoting the insertion of aquaporins (AQPs), which are water channels in the membranes of kidney cells. 2. Aquaporins (AQPs): o Aquaporins are transport proteins that form pores in the cell membranes of epithelial cells in the kidneys (especially in the collecting ducts). o These proteins allow water to pass through the cell membrane via osmosis. This process is essential for the kidneys to reabsorb water efficiently and maintain proper hydration. o Aquaporins are regulated by ADH, which stimulates their insertion into the membrane when the body needs to conserve water. o In 2003, the discovery of aquaporins and their importance in water transport earned Peter Agre the Nobel Prize in Chemistry. Summary of Kidney Function (Filtration & Reabsorption): The kidneys filter blood and reabsorb most of the water through a finely regulated process involving ADH and aquaporins. This helps maintain the body's fluid balance, ensuring proper hydration and the excretion of waste products in the urine. ★ Reabsorption in the Collecting Duct: Role of ADH and Aquaporins (AQPs) The collecting duct is a critical site in the kidney for the final reabsorption of water, and this process is highly regulated by Anti-Diuretic Hormone (ADH) and aquaporins (AQPs). Here's how these mechanisms work together: 1. Anti-Diuretic Hormone (ADH) ADH, also known as vasopressin, is a hormone that regulates water balance in the body. It is secreted by the posterior pituitary gland in response to changes in blood osmolality (the concentration of solutes in the blood) or blood volume. When blood becomes too concentrated (hypertonic) or when there is a drop in blood volume, ADH is released into the bloodstream. ADH acts specifically on the collecting ducts in the kidneys, where it promotes water reabsorption. 2. Mechanism of ADH Action in the Collecting Duct When ADH binds to receptors on the cells of the collecting duct, it activates a signaling pathway that leads to the insertion of aquaporins (AQPs) into the cell membranes of these tubule cells. ADH also stimulates the production of cyclic AMP (cAMP) inside the cells, which activates protein kinases that ultimately trigger the translocation of aquaporin water channels to the membrane. 3. Aquaporins (AQPs) Aquaporins are membrane proteins that form water channels, allowing water to pass through the cell membrane by osmosis. In the presence of ADH, aquaporins are inserted into the apical membrane of the collecting duct cells (the side facing the filtrate), allowing water to be reabsorbed from the filtrate back into the bloodstream. The insertion of AQPs increases the permeability of the collecting duct to water, enabling the kidneys to conserve water when necessary. 4. Water Reabsorption in the Collecting Duct As the filtrate moves through the collecting duct, water is reabsorbed into the surrounding interstitial space (fluid between the cells) and then into the bloodstream. The high concentration of sodium and other solutes in the medullary interstitial space (in the inner region of the kidney) creates an osmotic gradient that draws water out of the collecting duct through AQPs. This process is essential for producing concentrated urine when the body needs to conserve water. Without ADH, the collecting duct is much less permeable to water, and most of the water remains in the urine, resulting in dilute urine. 5. Summary of ADH and Aquaporins in the Collecting Duct ADH regulates the reabsorption of water in the collecting duct by promoting the insertion of aquaporins into the cell membranes. The presence of aquaporins increases the permeability of the collecting duct to water, allowing water to be reabsorbed from the filtrate and returned to the bloodstream. This process helps the body conserve water and maintain fluid balance, especially when dehydrated or during conditions requiring water conservation. Impact of ADH and Aquaporins on Urine Concentration: With ADH: The kidneys conserve water, producing concentrated urine. Without ADH: The kidneys excrete more water, resulting in dilute urine. Thus, ADH and aquaporins are essential for regulating the body's water balance and ensuring that the kidneys can adjust urine concentration based on the body's hydration status. ★ Reabsorption in collecting duct – ADH & AQPs In the collecting duct of the kidneys, the process of water reabsorption is regulated by antidiuretic hormone (ADH) and aquaporins (AQPs), particularly AQP-2. Here's how it works: 1. AQP-1: This aquaporin is always present in the basolateral membrane (the side of the epithelial cell facing the bloodstream) of the cells in the collecting duct. AQP-1 facilitates the passive movement of water from the tubular lumen (inside of the duct) into the interstitial space, allowing water to be reabsorbed into the blood. 2. AQP-2: Unlike AQP-1, AQP-2 is not always present on the apical (luminal) membrane of the collecting duct cells. In the absence of ADH, AQP-2 remains stored in secretory vesicles within the cytoplasm of the epithelial cells lining the collecting duct. This prevents water from being reabsorbed into the bloodstream, resulting in a large volume of dilute urine. 3. Role of ADH: When ADH is present, it binds to receptors on the cells of the collecting duct, triggering a signaling pathway that causes AQP-2 to be inserted into the apical membrane. This allows water to move from the urine in the collecting duct back into the bloodstream, concentrating the urine and reducing the volume of water excreted. 4. Absence of ADH: When ADH is absent (or in insufficient amounts), AQP-2 remains sequestered in the cytoplasm, and water cannot be reabsorbed from the urine. As a result, the urine becomes dilute, and a large volume of water is excreted from the body. Summary: With ADH present: AQP-2 moves to the apical membrane, water is reabsorbed, and urine becomes concentrated. Without ADH: AQP-2 stays in vesicles, water cannot be reabsorbed, and urine is dilute with a large volume. This mechanism is crucial for maintaining water balance in the body. When ADH (antidiuretic hormone) is present in the body, it triggers a series of events in the collecting duct of the kidneys that enable water reabsorption, leading to concentrated urine. Here's the process in more detail: 1. ADH Presence: ADH is released from the pituitary gland in response to factors like dehydration or increased blood osmolality. It travels through the bloodstream to the kidneys, where it acts on the collecting duct cells. 2. Insertion of AQP-2: ADH binds to specific receptors (V2 receptors) on the basolateral membrane of the epithelial cells in the collecting duct. This activates a signaling cascade, resulting in the insertion of AQP-2 (aquaporin-2) water channels into the apical membrane (the membrane facing the tubular lumen) of these cells. 3. Water Reabsorption: With AQP-2 now in the apical membrane, water can move from the urine in the collecting duct through the cells and into the interstitial fluid. From there, it is reabsorbed into the bloodstream via the peritubular capillaries. 4. Concentrated Urine: As water is reabsorbed back into the body, the urine becomes more concentrated. The volume of urine produced is reduced, and the body retains water to prevent dehydration. Summary of the process: With ADH: AQP-2 is inserted into the apical membrane, allowing water to be reabsorbed, and urine becomes concentrated. The body retains water, reducing urine volume. When ADH (antidiuretic hormone) is present, it plays a crucial role in the reabsorption of water in the collecting duct of the kidneys, resulting in concentrated urine and water retention by the body. Here's the step-by-step process: 1. ADH Binding: ADH is released from the pituitary gland in response to signals such as increased blood osmolarity or dehydration. It travels through the bloodstream to the kidneys. 2. Receptor Activation: ADH binds to specific receptors (V2 receptors) located on the basolateral membrane of the epithelial cells in the collecting duct. 3. Signaling Cascade: The binding of ADH activates a signaling pathway inside the cells. This results in the insertion of AQP-2 (aquaporin-2) water channels into the apical membrane (the membrane facing the tubular lumen) of the collecting duct cells. 4. Water Reabsorption: Once AQP-2 is present on the apical membrane, it allows water to pass through the epithelial cells from the urine in the collecting duct into the interstitial space. From there, water moves into the bloodstream, where it is retained by the body. 5. Concentrated Urine: As a result of water being reabsorbed, the urine becomes concentrated, and the volume of urine produced decreases. This helps the body retain water and maintain proper hydration levels. Key Point: With ADH: AQP-2 is inserted into the apical membrane, allowing water reabsorption, leading to concentrated urine and water retention. When ADH (antidiuretic hormone) is present, the following occurs in the collecting duct of the kidneys: 1. ADH Action: ADH is released from the pituitary gland and travels to the kidneys, where it binds to specific receptors (V2 receptors) on the basolateral membrane of the epithelial cells lining the collecting duct. 2. Insertion of AQP-2: Upon binding, ADH triggers a signaling pathway that causes AQP-2 (aquaporin-2) water channels to be inserted into the apical membrane (the side of the cell facing the urine) of the epithelial cells. 3. Water Reabsorption: The presence of AQP-2 on the apical membrane allows water to move from the collecting duct into the cells and then into the interstitial fluid. From there, water is reabsorbed into the bloodstream. 4. Concentrated Urine: As a result of this water reabsorption, the volume of urine decreases, and the urine becomes more concentrated. This process helps the body retain water and prevent dehydration. Key Outcome: With ADH: AQP-2 is inserted into the apical membrane, allowing water to be reabsorbed from the collecting duct, resulting in concentrated urine and water retention. If a person inherits two defective AQP-2 alleles, they would develop a genetic disorder known as Congenital Nephrogenic Diabetes Insipidus (NDI). Here's how it affects the body: 1. AQP-2 Deficiency: AQP-2 (aquaporin-2) is a water channel that allows water to be reabsorbed from the collecting duct into the bloodstream under the influence of ADH. If the AQP-2 gene is defective, the AQP-2 protein may be absent or malfunctioning, which means the water channels cannot be inserted into the apical membrane of the epithelial cells in the collecting duct. 2. Impact on Water Reabsorption: In the absence of functional AQP-2, even when ADH is present, water cannot be reabsorbed from the urine back into the body. This results in inability to concentrate urine. 3. Symptoms: o Polyuria (increased urine output) because the kidneys cannot concentrate the urine. o Polydipsia (excessive thirst) as the body attempts to compensate for the loss of water by stimulating the person to drink large amounts of water. o Dilute Urine: The urine remains very dilute and is excreted in large volumes, leading to the risk of dehydration if fluid intake is not adequately increased. Summary: If a person inherits two defective AQP-2 alleles, they would have Congenital Nephrogenic Diabetes Insipidus, leading to impaired water reabsorption in the kidneys, causing large volumes of dilute urine and the potential for dehydration. This condition typically requires management with increased fluid intake to prevent dehydration. If you inherit two defective AQP-2 alleles, you would develop Congenital Nephrogenic Diabetes Insipidus (NDI). This genetic disorder results in a significant impairment in the kidney's ability to concentrate urine due to defective aquaporin-2 (AQP-2) water channels in the collecting duct. Here's how it works: 1. Role of AQP-2: AQP-2 is a water channel that is inserted into the apical membrane of the collecting duct cells in response to ADH (antidiuretic hormone). ADH normally triggers the insertion of AQP-2 into the membrane, allowing water to be reabsorbed from the urine back into the bloodstream. 2. Defective AQP-2: When both alleles for AQP-2 are defective (inherited in an autosomal recessive manner), the AQP-2 water channels are either absent or nonfunctional. Even in the presence of ADH, the cells cannot reabsorb water effectively from the urine. 3. Impaired Water Reabsorption: Without functional AQP-2 channels, water cannot be reabsorbed from the collecting duct into the bloodstream, leading to: o Polyuria (large volumes of urine), o Polydipsia (excessive thirst), as the body tries to compensate for the water loss by increasing fluid intake. 4. Dilute Urine: The urine produced remains very dilute because the kidneys cannot concentrate it, resulting in large volumes of water being lost. Symptoms of Congenital Nephrogenic Diabetes Insipidus: Excessive urination (polyuria), Excessive thirst (polydipsia), Dilute urine. Management: Increased fluid intake is essential to prevent dehydration since the kidneys cannot concentrate urine properly. Medication (e.g., thiazide diuretics) may be used to help reduce urine output and manage symptoms. In summary, inheriting two defective AQP-2 alleles leads to Congenital Nephrogenic Diabetes Insipidus, a condition where the kidneys cannot reabsorb water properly, resulting in large volumes of dilute urine and excessive thirst. Overall regulation of body water The regulation of body water is a dynamic process involving several mechanisms to maintain homeostasis—the balance of water intake and output to ensure the body remains hydrated. This process involves various factors like ADH (antidiuretic hormone), thirst, and the effects of substances like caffeine and alcohol. Here's an overview of how body water intake and output are regulated: 1. Daily Water Loss: Daily loss: On average, the body loses around 2.5 L of water per day through urine, sweat, respiration, and feces. Daily intake: To maintain balance, the body needs to replace this 2.5 L of water through food and beverages. 2. Regulation of Intake/Output: Thirst Mechanism: Thirst is triggered when the body becomes dehydrated, stimulating the brain's thirst centers (mainly in the hypothalamus) to create the impulse to drink. o Dehydration increases blood osmolality (concentration of solutes like salts), and this stimulates the release of ADH. If the body is over-hydrated, thirst is suppressed, and the body signals to stop drinking. o Over-hydration reduces blood osmolality, and the body works to eliminate excess water through urine. Urine Production: The kidneys play a central role in maintaining water balance by regulating urine production in response to hydration status. Dehydration: When the body is dehydrated, there is an increase in blood osmolality. This triggers the release of ADH from the pituitary gland, which acts on the kidneys, specifically the collecting ducts. o ADH stimulates the insertion of aquaporin-2 (AQP-2) water channels into the apical membrane of the collecting duct cells, allowing more water to be reabsorbed into the bloodstream. This process results in the production of small amounts of concentrated urine to conserve water. Over-hydration: When the body is over-hydrated, blood osmolality decreases. ADH secretion is suppressed, and as a result, water channels (AQP-2) are not inserted into the collecting ducts, preventing water reabsorption. This leads to the production of large amounts of dilute urine to expel the excess water from the body. 3. Effects of Caffeine and Alcohol: Caffeine and alcohol both have a diuretic effect, which means they increase urine production by inhibiting the release of ADH: o Caffeine inhibits ADH release and thus reduces water reabsorption in the kidneys, leading to increased urine output. o Alcohol also inhibits ADH secretion, resulting in more water being excreted as urine and potentially causing dehydration. 4. Experiment on Water Regulation: In an experiment studying water regulation, you could examine the effects of dehydration, over-hydration, or the consumption of caffeine/alcohol on urine output and concentration. For example: After dehydration, you might observe increased ADH secretion, smaller volumes of concentrated urine. After over-hydration, you would observe reduced ADH secretion, larger volumes of dilute urine. Following the consumption of caffeine or alcohol, you would likely see increased urine production due to the inhibition of ADH release. Summary: Water Intake: Regulated by thirst signals, which are triggered by dehydration or suppressed by over-hydration. Urine Production: o Dehydration leads to ADH secretion, promoting water reabsorption and producing concentrated urine. o Over-hydration leads to no ADH secretion, producing dilute urine. Caffeine and Alcohol inhibit ADH secretion, causing increased urine output and potential dehydration. This regulation of body water ensures homeostasis by balancing intake and output based on hydration status. How is ADH regulated? Regulation of ADH (Antidiuretic Hormone) ADH is regulated by the plasma osmolarity, which is the concentration of osmotically active ions (like sodium) and molecules in the blood. Osmoreceptors in the hypothalamus constantly monitor the osmolarity of plasma, which is typically in the range of 280-295 mOsmol/kg. When osmolarity rises (indicating dehydration or an increased concentration of solutes in the blood), the hypothalamus signals the posterior pituitary to release ADH. ADH then acts on the kidneys to increase water reabsorption, thereby concentrating the urine and helping to restore normal osmolarity. Conversely, if osmolarity falls (indicating overhydration), ADH secretion is suppressed, leading to more dilute urine production and reduced water reabsorption. Do We Need to Calculate Water Loss Every Day? While the body does lose around 2.5 L of water per day (through urine, sweat, and other mechanisms), we do not need to consciously calculate or drink exactly 2.5 L daily. The body has a built-in system to manage this fluid balance. The thirst mechanism and the regulation of ADH ensure hydration without the need for constant monitoring of water intake. If you are dehydrated, the hypothalamus triggers the sensation of thirst, prompting you to drink. This system adjusts water loss based on your hydration status. In summary, the body's ability to regulate water intake and output is automatic, with the hypothalamus acting as a sensor to maintain plasma osmolarity and control ADH secretion, ensuring hydration without conscious effort. Hypothalamus senses osmolarity of plasma (fluid component of blood) The hypothalamus plays a central role in regulating the body’s water balance by monitoring the osmolarity of plasma (the fluid component of blood). Osmolarity refers to the concentration of osmotically active substances, primarily ions such as sodium and molecules like glucose, in the plasma. These substances influence the movement of water in and out of cells. Mechanism of Osmolarity Sensing: Osmoreceptors in the hypothalamus detect changes in plasma osmolarity. o When osmolarity increases (indicating dehydration or a higher concentration of solutes), the hypothalamus stimulates the release of ADH (antidiuretic hormone) from the posterior pituitary gland. o ADH then acts on the kidneys, increasing water reabsorption from the urine into the bloodstream, which helps restore normal osmolarity levels and conserves water in the body. o Conversely, when osmolarity decreases (indicating overhydration), ADH secretion is reduced, and less water is reabsorbed by the kidneys, resulting in the production of more dilute urine to expel the excess water. This osmolarity feedback system helps maintain homeostasis by regulating the volume and concentration of body fluids without requiring conscious input or effort from the individual. ★ Osmolarity In terms of body water regulation, osmolarity plays a key role in maintaining fluid balance and proper cellular function. Here's how it works: When osmolarity increases, the body detects higher concentrations of osmotically active substances (like salts or sugars) in the plasma. This can happen if: o The amount of substance in plasma increases (e.g., more solutes like sodium or glucose are present). o Volume decreases (e.g., dehydration, where water is lost, but solutes remain, leading to a higher concentration of substances in the blood). The body responds by triggering mechanisms to retain water to dilute the concentration of solutes and restore normal osmolarity. This might involve: o Thirst, prompting you to drink more water. o Release of antidiuretic hormone (ADH), which signals the kidneys to conserve water and reduce urine output. When osmolarity decreases, the body detects a lower concentration of solutes in the plasma. This can happen if: o The amount of substance in plasma decreases (e.g., low sodium levels due to excessive water intake or loss of solutes). o Volume increases (e.g., excess water intake dilutes the solutes in the blood). In response, the body tries to excrete excess water to maintain normal osmolarity. This could involve: o Decreased thirst to prevent further water intake. o Reduced release of ADH, causing the kidneys to excrete more water and produce more urine. In summary, the body uses osmolarity as a signal to regulate water intake and excretion to maintain fluid balance, ensuring that cells and tissues have the appropriate environment for function. Hypothalamus senses osmolarity of plasma (fluid component of blood) When osmolarity becomes too high, indicating dehydration, the body activates these two key mechanisms to restore fluid balance: 1. Increase water intake: o The thirst mechanism is triggered by osmoreceptors in the hypothalamus, which detect high osmolarity in the blood. o This leads to the sensation of thirst, prompting the individual to drink more fluids, which dilutes the solute concentration in the body and helps lower osmolarity. 2. Decrease water excretion: o The hypothalamus also stimulates the release of antidiuretic hormone (ADH), also known as vasopressin, from the pituitary gland. o ADH acts on the kidneys, specifically on the collecting ducts. It binds to receptors on the cells, leading to the insertion of aquaporin-2 (AQP-2) channels in the membranes of kidney cells. These channels allow water to be reabsorbed back into the bloodstream, reducing water loss in urine. o As a result, more water is retained, concentrating the urine and reducing the volume of water excreted. Both of these actions (drinking water and reducing water excretion) work together to restore osmolarity to normal levels by increasing the body's water content and decreasing water loss, thereby correcting dehydration. What happens if you exercise then drink water? Osmolarity and Water Loss: Osmolarity is the concentration of osmotically active substances (e.g., ions, glucose) in the plasma, which can be described by the equation: When the amount of water in the plasma decreases (due to sweating or lung evaporation), but the amount of solutes stays the same, the plasma becomes more concentrated—thus, osmolarity increases. Hypothalamus Response: The hypothalamus detects the rise in osmolarity (due to dehydration) and recognizes that the body is in a state of water deficiency. o Thirst response: The hypothalamus triggers the sensation of thirst, prompting you to drink water to restore fluid balance. o ADH release: The hypothalamus also signals the pituitary gland to release antidiuretic hormone (ADH) into the bloodstream. ▪ ADH acts on the kidneys, causing them to reabsorb more water from the filtrate back into the bloodstream. This is achieved through the insertion of aquaporin-2 (AQP-2) channels into the cells of the kidney's collecting ducts, allowing more water to be reabsorbed. Net Result - Restoring Homeostasis: By drinking water (to increase fluid intake) and by reabsorbing water through ADH (to reduce water loss via urine), the body effectively restores normal osmolarity. This process helps maintain homeostasis, ensuring that the fluid and electrolyte balance is preserved. Conclusion: In this way, the body uses both behavioral (thirst) and physiological (ADH release and water reabsorption) mechanisms to counter dehydration and keep osmolarity within a normal range. What happens if you exercise then drink alcohol? Osmolarity and Dehydration: Osmolarity is determined by the ratio of the amount of osmotically active substances (like salts or glucose) to the volume of water in the plasma. When water is lost (e.g., through sweating or lung evaporation), but the solutes remain in the plasma, osmolarity increases (because there’s less water to dilute the solutes). Hypothalamus Response: The hypothalamus detects this increase in osmolarity (indicating dehydration) and activates the following responses: o Thirst response: It triggers the sensation of thirst, encouraging you to drink more water. o ADH release: The hypothalamus signals the pituitary gland to release antidiuretic hormone (ADH). ADH acts on the kidneys, specifically on the collecting ducts, to increase water reabsorption into the bloodstream. This is achieved by inserting aquaporin-2 (AQP-2) channels into the cell membranes, allowing water to be reabsorbed. Alcohol’s Impact: Alcohol interferes with this response by blocking the release of ADH from the pituitary gland. Without ADH: o AQP-2 channels are not inserted into the kidney cells. o Water is not reabsorbed, so it is lost in urine instead. o This prevents the body from correcting the increased osmolarity caused by dehydration, leading to continued water loss and failure to restore homeostasis. Net Result: Since alcohol blocks ADH, water continues to be lost in urine, even though the body is in a dehydrated state. The plasma osmolarity remains elevated, and the body is unable to rehydrate properly, disrupting homeostasis. Conclusion: While the body would typically respond to increased osmolarity by reabsorbing water through ADH, alcohol blocks this mechanism, leading to further dehydration and a failure to restore normal osmolarity. This is why alcohol can contribute to dehydration and worsen the body's ability to maintain fluid balance. Concept of homeostasis ★ Maintenance of internal body environment (including body water) Homeostasis: Claude Bernard (1813-1878) is credited with the foundational concept that the internal environment of the body remains stable, despite changes in the external environment. He recognized that the body’s ability to maintain a relatively constant internal state is essential for proper function. Walter Cannon (1871-1945) further developed this idea and coined the term "homeostasis" to describe the body's ability to maintain internal stability (such as temperature, pH, and fluid balance) through regulatory mechanisms, even when faced with external changes. Examples of Homeostasis: 1. Body Temperature: o The body must maintain a core temperature around 37°C (98.6°F). If external temperature changes (e.g., heat or cold), the body adjusts through mechanisms like sweating or shivering to restore normal temperature. 2. Blood Pressure: o Blood pressure needs to remain within a certain range to ensure proper circulation of oxygen and nutrients. If blood pressure drops too low or rises too high, the body adjusts through mechanisms like vasoconstriction or vasodilation, or altering heart rate. 3. Heart Rate: o The heart rate adjusts in response to activity levels, stress, or other factors to ensure that sufficient blood is delivered to tissues. The autonomic nervous system regulates the heart rate to maintain homeostasis. 4. Respiration Rate: o The respiratory rate adjusts to maintain proper oxygen levels in the blood and to regulate carbon dioxide levels. If oxygen levels drop or carbon dioxide rises, the body increases the rate of breathing to correct the imbalance. Other Examples of Homeostasis: Blood Glucose Levels: Regulated by insulin and glucagon, maintaining a stable concentration of glucose in the blood. Electrolyte Balance: The body regulates the levels of ions like sodium, potassium, and calcium to ensure normal cellular function. Homeostasis in Action: These processes are typically controlled by feedback mechanisms, such as negative feedback, where the body works to counteract deviations from a set point (e.g., lowering blood pressure if it rises too high) or positive feedback, where a change is amplified (e.g., during childbirth, where contractions lead to the release of oxytocin, further intensifying contractions). In summary, homeostasis is the body’s vital ability to maintain internal stability, ensuring that critical functions remain within a range that supports life, despite external environmental changes. Feedback loops – role in Homeostats 1. Sensor (Receptor): The sensor detects changes in the internal or external environment, such as changes in temperature, blood pressure, or pH levels. These sensors are specialized cells or organs that can detect specific variables. o Example: Temperature receptors in the skin or hypothalamus detect whether the body is too hot or too cold. 2. Integrating Centre (Control Centre): The integrating centre receives the signal from the sensor and processes the information. It then determines the appropriate response to correct any imbalance. The integrating centre is typically located in the brain or spinal cord. o The hypothalamus, for example, is an integrating centre for temperature regulation, where it compares the actual temperature to the body’s set point (e.g., 37°C). o Example: When the body temperature is too high, the hypothalamus receives signals from temperature receptors and processes this information to activate cooling mechanisms. 3. Effectors: The effectors are the organs or systems that carry out the response to bring the variable back to normal. They receive signals from the integrating centre and perform actions to restore balance. o Example: If the body is too hot, sweat glands act as effectors to produce sweat, which cools the body through evaporation. o Example: If the body is too cold, muscles act as effectors to initiate shivering, generating heat to raise the body temperature. 4. Feedback Control: Feedback is the mechanism by which the response of the effectors is regulated, ensuring the system works to maintain homeostasis. o Negative Feedback: The most common type of feedback in homeostasis. The activity of the effectors is opposed or reversed when the condition goes beyond the set point, bringing the system back to equilibrium. ▪ Example: If body temperature increases, sweat glands activate to cool the body down. As temperature normalizes, the sweating process decreases, preventing overheating. ▪ Example: If blood sugar levels rise, insulin is released to lower the sugar levels. Once blood sugar reaches normal levels, insulin secretion stops. o Positive Feedback (less common, but still important): ▪ In positive feedback, the response amplifies the initial change rather than reversing it. ▪ Example: During childbirth, the release of oxytocin causes uterine contractions, which stimulate more oxytocin release, intensifying the contractions until delivery occurs. This cycle continues until the baby is born. ▪ Positive feedback is usually self-limiting and ends once a specific event is achieved. Summary: In homeostatic regulation, the sensor detects a change, the integrating centre processes it, and the effectors carry out the necessary action to return the system to normal. Feedback control, primarily negative feedback, ensures that this process works to stabilize the internal environment. ★ Control of body temperature by negative feedback 1. When the Body Gets Too Hot: Sensor Activation: o The temperature receptors (sensors) in the skin or hypothalamus detect an increase in body temperature (e.g., from exercise, heat exposure, etc.). Effect: o The integrating center (hypothalamus) activates effectors like sweat glands, triggering sweating. Lag Period: o After a short lag period, as sweat evaporates from the skin, it cools the body, gradually decreasing the body temperature. Sensor Deactivation: o Once the body temperature returns to the normal range (e.g., around 37°C), the sensors are deactivated. Stabilization: o After the temperature normalizes, the body stabilizes its temperature, and sweating gradually stops. This completes the negative feedback loop, where the response (sweating) decreases as the body returns to homeostasis. 2. When the Body Gets Too Cold: Sensor Activation: o When the body temperature drops (e.g., due to cold weather), temperature receptors detect the decrease. Effect: o The integrating center (hypothalamus) activates shivering as an effector mechanism to generate heat. Lag Period: o After a short lag period, shivering produces heat through muscle contractions, gradually increasing the body temperature. Sensor Deactivation: o Once the body temperature returns to the normal range, the temperature sensors stop detecting the cold. Stabilization: o After the temperature normalizes, shivering stops, stabilizing the body temperature. Summary: Sweating is the body’s response to overheating (high temperatures), and shivering is the response to cold (low temperatures). Both mechanisms work in a feedback loop, where the sensor detects the temperature change, the effectors (sweat glands or muscles) correct the temperature, and the sensor deactivates once the temperature is back to normal. The lag periods reflect the time it takes for the body to respond and stabilize. These processes ensure homeostasis, keeping the body's internal environment stable despite changes in external conditions. 1. Sensor Failure to Detect a Drop in Temperature: Normal Function: The body's temperature sensors in the skin and hypothalamus detect when the internal temperature drops below the normal range (typically around 37°C). If the sensor fails: If the sensors don't detect the drop in temperature or there's a problem with the hypothalamus, the body doesn't initiate the appropriate response to counter the cold. 2. No Activation of Effectors: When the temperature receptors fail to activate, the body won’t trigger the shivering response or constrict blood vessels (vasoconstriction) to conserve heat. This means the body cannot generate enough heat or retain the heat needed to stabilize the temperature. 3. Consequences - Hypothermia: Without the body’s ability to regulate temperature properly, the body continues to lose heat to the environment without replenishing it. As the body temperature falls below 35°C, it enters hypothermia, a dangerous condition where the body becomes too cold to function properly. Symptoms of hypothermia include shivering (initially), confusion, slurred speech, numbness, and eventually loss of consciousness. 4. Severe Hypothermia and Death: If the temperature continues to drop without intervention, severe hypothermia can set in, leading to organ failure, unconsciousness, and ultimately death. The failure to activate the appropriate sensors and effectors can result in the body’s inability to restore normal temperature, putting survival at risk. Summary: Proper sensor function is crucial for detecting temperature changes, and if this system fails to activate when the body temperature drops too low, the body cannot respond to prevent hypothermia. Without shivering, vasoconstriction, or other heat-generating mechanisms, the body would continue to lose heat, potentially leading to life-threatening hypothermia and death. Why is it called negative feedback? You're on the right track with the concept of negative feedback! Here's how the mathematical analogy works and why it's called negative feedback in biological systems: 1. The Concept of Feedback: In the context of homeostasis, feedback refers to the body's response to a change in a certain variable (e.g., temperature, blood pressure, glucose levels). The body tries to counteract the change in the variable and bring it back toward a set point or normal range. 2. Why "Negative" Feedback? The term negative feedback comes from the mathematical concept of a negative change or opposite direction: If something (like body temperature) deviates from the set point (e.g., 37°C), the system will respond in the opposite direction to bring it back toward normal. Negative means that the response opposes the original change. For example: o If the body is too hot, the body activates cooling mechanisms (like sweating) to bring the temperature down. o If the body is too cold, the body activates warming mechanisms (like shivering) to bring the temperature up. 3. Mathematical Analogy: Now, applying your analogy with gradients: The gradient represents the rate of change of a variable. In your case, the temperature change (or the difference from the normal temperature) is represented by the gradient. Gradient of +1: This could represent a positive increase in temperature (e.g., temperature rises above 37°C). Gradient of -1: This represents a negative change, where temperature falls back down toward the set point (e.g., cooling mechanisms working to lower temperature). To convert a positive gradient (increasing temperature) to a negative gradient (decreasing temperature), you multiply by -1: Positive gradient (+1) becomes negative gradient (-1). The system reacts to the change by trying to reverse the trend, bringing the temperature back to normal. 4. Set Point and Normal Range: Set Point (e.g., 37°C) is the normal, target temperature the body aims to maintain. The range around the set point is the normal fluctuation (e.g., 36.5°C to 37.5°C). If the temperature moves outside this range (either above or below), the feedback system works in the opposite direction (negative feedback) to return it to the normal range. 5. Negative Feedback in Action: If temperature increases (e.g., +1 gradient), the body activates cooling mechanisms (like sweating), causing the temperature to decrease (e.g., -1 gradient). If temperature decreases (e.g., -1 gradient), the body activates warming mechanisms (like shivering), causing the temperature to increase (e.g., +1 gradient). Summary: Negative feedback is a system where the response opposes the initial change (the "gradient"). It’s called "negative" because the body's response works in the opposite direction of the deviation from the set point, trying to bring the variable back to normal. It’s like multiplying by -1 to reverse the direction of change, bringing things back to homeostasis. Disruption in the negative feedback pathways You're absolutely right! Illnesses often occur when there is a disruption in the negative feedback pathways that normally maintain homeostasis. When these regulatory systems fail, the set point of a given physiological parameter is disrupted, leading to an imbalance. Here's a more detailed explanation of the examples you've provided: 1. Cancer - Disregulation of Cell Division: Normal Function: Cell division is tightly controlled by a variety of feedback mechanisms to maintain normal growth and repair. Disruption: In cancer, negative feedback mechanisms that regulate cell division fail. This can be due to mutations in genes controlling the cell cycle, like tumor suppressor genes (e.g., p53) or oncogenes. Result: The normal set point for cell growth is disregulated, leading to uncontrolled cell division and tumor formation. 2. Cholera - Disregulation of Water Balance: Normal Function: The body regulates water balance through mechanisms like the release of antidiuretic hormone (ADH), which helps control water reabsorption in the kidneys. Disruption: In cholera, the bacterium produces toxins that disrupt the normal function of the intestinal cells, causing an overproduction of cAMP. This leads to excessive secretion of chloride ions into the intestines, which pulls water with it. Result: The body loses large amounts of water, leading to dehydration and electrolyte imbalance. The negative feedback systems controlling water balance are overwhelmed. 3. Fever - Disregulation of Body Temperature: Normal Function: The body maintains a normal body temperature around the set point (about 37°C) through negative feedback mechanisms such as sweating and shivering. Disruption: In response to infection, the immune system releases pyrogens that signal the hypothalamus to raise the set point for body temperature. This causes a fever, an intentional increase in body temperature as part of the immune response. Result: The body's normal temperature regulation is disrupted, but this is usually a temporary, beneficial adaptation. However, if the fever becomes too high or prolonged, it can become harmful. 4. Cystic Fibrosis - Disregulation of Airway Surface Liquid Composition: Normal Function: The composition of airway surface liquid is carefully balanced to keep mucus thin and allow for proper airway clearance. This balance is maintained by feedback regulation of ion transport across the epithelial cells. Disruption: In cystic fibrosis, mutations in the CFTR gene lead to defective chloride ion transport in epithelial cells, disrupting the normal balance of salt and water on the airway surface. Result: The disrupted ion transport leads to thick, sticky mucus buildup in the airways, which impairs lung function and makes the body more susceptible to infections. Summary: Illness occurs when negative feedback mechanisms fail or become disregulated, leading to a disruption of the normal set point of a physiological parameter. Whether it’s cell division in cancer, water balance in cholera, body temperature in fever, or airway surface liquid composition in cystic fibrosis, the result is a failure to maintain homeostasis, causing health problems. The key feature of these diseases is that the body’s ability to regulate important physiological processes is impaired, often due to genetic mutations, infections, or external factors. Positive feedback Yes, positive feedback does exist, and blood clot formation is a great example of it! Positive Feedback in Blood Clot Formation: In positive feedback, the initial stimulus is amplified rather than reversed, like in negative feedback. Here's how it works in blood clotting: 1. Initial Injury: o When a blood vessel is injured, the body activates a series of proteins (clotting factors) to form a clot and stop the bleeding. 2. Clotting Cascade (Positive Feedback): o The activation of the first clotting factor triggers the activation of subsequent clotting factors in a sequential cascade. o This amplifies the clotting process, meaning that each activated factor speeds up the activation of the next one, resulting in a rapid and strong response. o Positive feedback happens here because each step in the clotting process leads to more activation of the next factor, making the clotting response stronger and faster. 3. Formation of the Clot: o The final step is the conversion of fibrinogen into fibrin, which forms the mesh that creates the clot and stops the bleeding. 4. Transition to Negative Feedback (Homeostasis): o Once the clot has formed and the bleeding has stopped, the process transitions to negative feedback. o Negative feedback mechanisms, such as the release of anticoagulants, are activated to stop further clotting, preventing excessive clot formation that could lead to a blockage in the blood vessels. Key Points: Positive feedback amplifies the process (increasing clotting factor activity). The clot formation process ultimately helps maintain homeostasis by stopping blood loss. Once the clot is formed, negative feedback mechanisms take over to limit clotting and restore the system to normal. Summary: Positive feedback in blood clot formation is an example of how the body uses amplification to rapidly respond to an injury. However, once the clot forms, the system shifts to negative feedback to ensure the process doesn’t continue unchecked, maintaining overall homeostasis.