Bioenergetics and Oxidative Metabolism 2024 PDF
Document Details
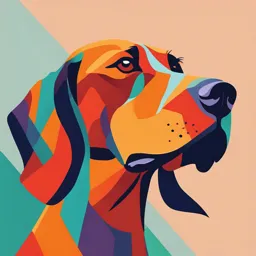
Uploaded by PromptAsteroid
USC
2024
Tags
Summary
This document provides a high-level overview of bioenergetics and oxidative metabolism. It contains the contents, learning objectives, and introduces the processes involved, including sections on the three stages of intermediate metabolism. It is a useful resource for students studying bioenergetics and related biological topics.
Full Transcript
3 BIOENERGETICS AND OXIDATIVE METABOLISM 1 BIOENERGETICS — CONTENTS — I. THE THREE STAGES OF METABOLISM V. OXIDATIVE PHOSPHORYLATION...
3 BIOENERGETICS AND OXIDATIVE METABOLISM 1 BIOENERGETICS — CONTENTS — I. THE THREE STAGES OF METABOLISM V. OXIDATIVE PHOSPHORYLATION Mechanism: chemiosmotic coupling II. THE PYRUVATE DEHYDROGENASE COMPLEX Inhibitors and uncouplers Reaction catalyzed by the PDH complex Sites of oxidative phosphorylation Regulation of the PDH complex Clinical correlations Hypoxic injury III. THE TRICARBOXYLIC ACID CYCLE Uncoupling proteins and obesity Reactions and stoichiometry of the TCA Regulation of the TCA VI. MITOCHONDRIAL TRANSPORT SYSTEMS The TCA and Inflammation Transport of reducing equivalents TCA and Macromolecule synthesis Transport of acetyl units Clinical correlations Transport of adenine nucleotides PDH deficiency Beri-beri IV. THE MITOCHONDRIAL RESPIRATORY CHAIN Complexes and mobile carriers Direction of electron transfer Where electrons enter the respiratory chain Inhibition of the respiratory chain Cyanide poisoning 2 3. BIOENERGETICS – LEARNING OBJECTIVES – Understand the principles of reversible phosphorylation in regulation of enzyme activity Analyze the central role of acetyl-CoA, a metabolite at the cross- roads of intermediate metabolism Discuss the principles that support a therapeutic approach to pyruvate dehydrogenase deficiency Apply knowledge on energy yield (ATP generated) depending on site of entry of reducing equivalents to the respiratory chain Discuss the mechanism of energy conservation in the respiratory chain and how can it be impaired by inhibitors or uncouplers Explain why transport systems are required for exchange of metabolites between mitochondria and cytosol? 3 I. THE THREE STAGES OF INTERMEDIATE METABOLISM PROTEINS CARBOHYDRATES LIPIDS Glycolysis Gluconeogenesis Stage I Glycogenolysis Glycogenesis amino acids hexoses glycerol pentoses + fatty acids deamination oxidation glycolysis b-oxidation Stage II ANABOLISM CATABOLISM pyruvate acetyl-CoA TCA Stage III respiratory chain ATP NH3 H2O CO2 4 II. THE PYRUVATE DEHYDROGENASE MULTIENZYME COMPLEX Reaction catalyzed by the pyruvate dehydrogenase complex (PDH) The oxidative decarboxylation of pyruvate to acetyl CoA is catalyzed by the pyruvate dehydrogenase complex (PDH), an organized assembly consisting of three different enzymes. The overall reaction catalyzed by the pyruvate dehydrogenase complex is: pyruvate + HS-CoA + NAD+ → acetyl-CoA + CO2 + NADH The reaction mechanism entails: Catalytic cofactors: thiamine pyrophosphate (TPP; vitamin B1), lipoamide, and FAD. These cofactors do not show in the overall equation because they are regenerated during the enzyme cycle. Stoichiometric cofactors, HS-CoA and NAD and they show in the overall equation 5 II. THE PYRUVATE DEHYDROGENASE MULTIENZYME COMPLEX Reaction catalyzed by the pyruvate dehydrogenase complex (PDH) Pyruvate dehydrogenase is a multienzyme complex containing three enzymes that catalyze four steps leading to the final formation of acetyl-CoA. Step Enzyme Component First Step Pyruvate dehydrogenase (E1) Second Step Dihydrolipoyl transacetylase (E2) Third Step Dihydrolipoyl dehydrogenase (E3) PYRUVATE STEP ENZYME COMPONENT E2 First step Lipoamide Pyruvate dehydrogenase (E1) Second step E1 TPP Third step Dihydrolipoyl transacetylase (E2) E3 Fourth step Dihydrolipoyl dehydrogenase (E3) FAD ACETYL-CoA 6 II. THE PYRUVATE DEHYDROGENASE MULTIENZYME COMPLEX Regulation of the pyruvate dehydrogenase complex activity a. Inhibition by products – The products of the oxidation of pyruvate –acetyl CoA and NADH– inhibit the enzyme complex. pyruvate + HS-CoA + NAD+ acetyl-CoA + CO2 + NADH PDH b. Feedback regulation by nucleotides – The energy status of the cell controls PDH activity: When the cell is rich in available energy (e.g., [GTP]), the activity of the PDH complex is reduced. Conversely, when the cell’s energy stores are low (e.g., [AMP]), PDH is activated. pyruvate + HS-CoA + NAD+ acetyl-CoA + CO2 + NADH PDH GTP AMP GTP AMP 7 II. THE PYRUVATE DEHYDROGENASE MULTIENZYME COMPLEX Regulation of the pyruvate dehydrogenase complex activity c. Regulation by reversible phosphorylation – The enzyme complex is active in the non-phosphorylated form and inactive when phosphorylated on the E1 subunit. Pyruvate dehydrogenase kinase (PDK) catalyzes the conversion of the active (non-phosphorylated) to the inactive ATP/ADP ATP/ADP Acetyl-CoA/CoA Acetyl-CoA/CoA (phosphorylated) forms of PDH. Pyruvate NADH/NAD NADH/NAD pyruvate pyruvate dehydrogenase kinase activity is stimulated PDKPDK by high ratios of ATP/ADP, acetyl CoA/CoA, and NADH/NAD+ and inhibited by pyruvate. The enzyme complex becomes active again E2 E2 E2 E2 E1 E1 P P E1 E1 when the phosphoryl group is removed by E3 E3 E3 E3 protein phosphatase 1 (PPase), which is stimulated by high levels of Ca2+ and insulin. Insulin activates the phosphatase in adipose PPase PPase tissue but not in liver. insulin insulin Ca Ca2+ 2+ 8 II. THE PYRUVATE DEHYDROGENASE MULTIENZYME COMPLEX – SUMMARY – ATP/ADP Acetyl-CoA/CoA NADH/NAD pyruvate HSCoA + NAD+ + PYRUVATE GTP AMP PDK E2 E2 E1 P E1 E3 E3 ACETYL-CoA acetyl-CoA PPase + NADH CO2 + NADH insulin Ca2+ 9 WHY METABOLISM OF CARBOHYDRATES AND LIPIDS? SIGNIFICANCE: STARVE-FEED CYCLE DIABETES OBESITY ATHEROSCLEROSIS CELL SIGNALING ALZHEIMER’S DISEASE [lactate] insulin [pyruvate] glucagon HYPOXIA b cells a cells Pancreas 10 II. THE PYRUVATE DEHYDROGENASE MULTIENZYME COMPLEX Pyruvate dehydrogenase generates acetyl-CoA from pyruvate (originating from glycolysis). Other sources of acetyl-CoA are fatty acid oxidation and certain amino acids. The fates of acetyl-CoA generated in the mitochondrial matrix include (a) complete oxidation of the acetyl group in the tricarboxylic acid cycle; (b) conversion of excess acetyl CoA into ketone bodies (in the liver), and (c) transfer of acetyl units to the cytosol for the synthesis of sterols and long-chain fatty acids. PYRUVATE SOURCES AMINO ACIDS FATTY ACIDS ACETYL-CoA FATES TRICARBOXYLIC FATTY ACIDS ACID CYCLE STEROLS KETONE BODIES 11 I. THE THREE STAGES OF INTERMEDIATE METABOLISM PROTEINS CARBOHYDRATES LIPIDS Glycolysis Gluconeogenesis Stage I Glycogenolysis Glycogenesis amino acids hexoses glycerol pentoses + fatty acids deamination oxidation glycolysis b-oxidation Stage II ANABOLISM CATABOLISM pyruvate acetyl-CoA TCA Stage III respiratory chain ATP NH3 H2O CO2 12 III. THE TRICARBOXYLIC ACID CYCLE PYRUVATE The primary metabolic fate of acetyl-CoA PDH CO2 produced is its complete oxidation in the ACETYL-CoA tricarboxylic acid cycle (or citric acid cycle or Krebs cycle) to CO2. A primary function of the IDH CO2 tricarboxylic acid cycle (TCA) is to generate TCA KGDH reducing equivalents (e.g., NADH and CO2 FADH2) that are utilized in the mitochondrial FPH2 electron-transport chain to generate ATP NADH RESPIRATORY CHAIN O2 through oxidative phosphorylation. ATP 13 III. THE TRICARBOXYLIC ACID CYCLE pyruvate NAD+ + CoA Reactions of the TCA PDH Two carbon atoms enter the cycle NADH + CO2 acetyl-CoA as acetyl-CoA condenses with citrate oxaloacetate citrate synthase oxalo- Two carbon atoms leave the cycle acetate cis-aconitate as CO2 malate DH aconitase Four pairs of reducing equi- malate isocitrate valents leave the cycle: three as NADH NAD+ isocitrate DH NADH and one as FADH2 fumarase CO2 One high-energy phosphate bond fumarate a-keto- glutarate (GTP) is generated from the succinate DH a-ketoglutarate DH (complex II) NAD+ + CoA energy-rich thioester linkage in FADH2 succinyl- FAD succinate CoA succinyl-CoA CO2 succinyl CoA synthase Pi + GDP GTP + CoA 14 III. THE TRICARBOXYLIC ACID CYCLE Regulation of the TCA is achieved at three control points: The first control point is determined by the synthesis of citrate from oxaloacetate and acetyl CoA. ATP inhibits citrate synthase. The second control point is isocitrate dehydrogenase, stimulated by ADP and inhibited by NADH and ATP. The third control point is a-ketoglutarate dehydrogenase, inhibited by the products of the reaction: succinyl CoA and NADH. CONTROL POINTS MODULATORS CITRATE SYNTHASE ATP TRICARBOXYLIC ACID ISOCITRATE ATP, NADH DEHYDROGENASE ADP CYCLE a-KETOGLUTARATE Succinyl-CoA, DEHYDROGENASE NADH 15 THE PYRUVATE DEHYDROGENASE COMPLEX AND THE TRICARBOXYLIC ACID CYCLE 16 THE TRICARBOXYLIC ACID CYCLE AND INFLAMMATION ROLES OF SUCCINATE AND ITACONATE AS SIGNAL TRANSDUCERS PRO-INFLAMMATORY ANTI-INFLAMMATORY COX2 ¯ ANTI-INFLAMMATORY PGE2→ IL1b HIF1a CYTOKINES PLASMA SUCNR1 MEMBRANE ¯OXIDATIVE STRESS succinate MITOCHONDRIA itaconate DIABETES citrate ISCHEMIA/REPERFUSION oxalo- O OXIDATIVE STRESS acetate cis-aconitate itaconate OH HO O malate isocitrate NADH fumarate a-keto- glutarate succinyl- succinate CoA Succinate-SUCNR1 signaling serves as a link between metabolic stress and inflammation 17 THE TRICARBOXYLIC ACID CYCLE METABOLITES SUPPORT MACROMOLECULE BIOMASS glucose glucose P-enol pyruvate pyruvate acetyl-CoA oxaloacetate fatty acids citrate sterols malate isocitrate succinyl-CoA fumarate aspartate succinate a-ketoglutarate asparagine succinyl-CoA glutamate proteins nucleotides porphyrins heme purines glutathione amino acids 17 glucose III. CLINICAL CORRELATIONS Pyruvate dehydrogenase deficiency in lactate pyruvate alanine children involves genetic deficiencies of the (lactic PDK dichloro- dichloroacetate acidemia) acetate phenylbutyrate catalytic or regulatory subunits of the P PDH PDH pyruvate dehydrogenase complex. Symptoms: Children exhibit elevated plasma ketone acetyl-CoA PPase bodies levels of lactate, pyruvate, and alanine, which produce chronic lactic acidosis and ketogenic diet serious neurological defects which, in most cases, results in death. TCA Diagnosis is made by assessing PDH activity in cultured skin fibroblasts from the patient. NADH NADH FPH2 Treatment: patients may respond to dietary management FPH2 consisting of a ketogenic diet (ketones as alternative source respiratory chain respiratory chain ATP of energy). Improvement with dichloroacetate, an inhibitor ATP of pyruvate dehydrogenase kinase, thus preventing inactivation of the enzyme complex. There are currently 2 NIH clinical trials on PDH deficiency: one advocates phenylbutyrate treatment and another dichloroacetate. 19 III. CLINICAL CORRELATIONS Beri-Beri Beri-beri is due to a deficiency of thiamine (vitamin B1) in the diet. This deficiency causes neurologic and cardiovascular symptoms, including edema and heart enlargement. Beri-beri is still a health problem in some parts of Asia and with malnourished populations in the Western world (especially with alcoholics). Vitamin B1 is TPP (thiamine pyrophosphate), a prostetic group of pyruvate dehydrogenase, a-ketoglutarate dehydrogenase (TCA cycle), and transketolase (pentose phosphate pathway). In beri-beri, the serum levels of pyruvate and a-ketoglutarate are high. Usually, the condition is diagnosed by the low activity of transketolase (which requires TPP) in erythrocytes. _______________________ mitochondria ________________________ _________ cytosol _________ _____ glycolysis _____ _______ TCA _______ _______ PPP _______ pyruvate DH TPP a-ketoglutarate DH TPP transketolase TPP 20 IV. THE MITOCHONDRIAL RESPIRATORY CHAIN The components of the mitochondrial respiratory chain are complexes (I to V) and two mobile carriers: coenzyme Q and cytochromes. These components of the mitochondrial respiratory chain are embedded in the inner membrane. 21 IV. THE MITOCHONDRIAL RESPIRATORY CHAIN RESPIRATORY CHAIN COMPLEXES Four large complexes linked by two mobile OMM IMM carriers arranged in a sequential pattern in the MATRIX OMMIMS IMM MATRIX IMM according to their reduction potentials. IMS MATRIX COMPLEXES I I Complex I (NADH-coenzyme Q reductase) Complex II (succinate dehydrogenase) (cont- II II QQ SDHSDH TCA TCA ains a flavoprotein: FP2). III Complex III (cytochromes b-c1) III Complex IV (cytochrome oxidase) (contains c c IV cytochromes a and a3) IV MOBILE CARRIERS Coenzyme Q Cytochrome c 22 IV. THE MITOCHONDRIAL RESPIRATORY CHAIN Direction of electron transfer NADH OMM IMM electrons Reducing equivalents enter at the level of IMS either complex I (electrons in the form of e– FPH2 NADH) or coenzyme Q (electrons from electrons I FPH2). direction of electron transfer Coenzyme Q donates the electrons to e– Q complex III and this to the mobile carrier cytochrome c. III Electrons from cytochrome c are channeled c to complex IV, where they are used to O2 IV reduce O2 to H2O. H2O 23 IV. THE MITOCHONDRIAL RESPIRATORY CHAIN Where electrons from various pathways enter the mitochondrial respiratory chain OMM IMM Pyruvate DH IMS a-Ketoglutarate DH Isocitrate DH TCA NADH Malate DH I b-Hydroxyacyl-CoA DH FP1 NAD+ b-Hydroxybutyrate DH Succinate DH (Complex II) Q FPH2 Fatty acyl-CoA DH Glyerol-P DH FP III c O2 IV H2O 24 IV. THE MITOCHONDRIAL RESPIRATORY CHAIN Inhibitors of the respiratory chain OMM IMM Inhibition of the mitochondrial electron IMS MATRIX transport results in impairment of normal energy-generated function and death of the I rotenone organism. amytal Rotenone (a fish poison) and amytal (a Q II barbiturate) inhibit at the level of Complex I. Antimycin A inhibits electron transfer at the III antimycin A Complex III site. Complex IV or cytochrome oxidase is c CN– inhibited by cyanide, azide, and carbon IV N3 – monoxide. CO 25 IV. THE MITOCHONDRIAL RESPIRATORY CHAIN Cyanide (CN–) poisoning CN– (as hydrogen cyanide gas or KCN) is one of the most potent acting poisons known; it causes rapid inhibition of the mitochondrial electron transport chain Complex IV. It binds to the Fe3+ in the heme of the cytochrome aa3 of Complex IV, thus preventing O2 from reacting with aa3: mitochondrial respiration and energy production cease and cell death occurs rapidly. Death to CN– poisoning occurs from tissue asphyxia, most notably of the central nervous system. OMM IMM OMM IMM IMS MATRIX IMS MATRIX I I Q II Q II III III c c IV IV aa3 -Fe3+-O 2 aa3-Fe3+-CN O2-binding site CN–-binding site 26 IV. THE MITOCHONDRIAL RESPIRATORY CHAIN Cyanide (CN–) poisoning HbO2 (Fe2+) NO2– If diagnosed rapidly, the patient can be OMM IMM OMM IMM OMM IMM given various nitrites (NO2–) that convert NO, N2O IMS MATRIX IMS MATRIX IMS MATRIX oxyhemoglobin to methemoglobin, (thus MetHb (Fe3+–CN) I I I facilitating the conversion of the Fe2+ of MetHb (Fe3+) Q II Q II Q II hemoglobin to Fe3+ in methemoglobin). III III III Methemoglobin (Fe3+) competes with c c c IV IV IV cytochrome aa3 aa(Fe 3+ 3+ 3-Fe -O) 2 for CN–, forming a aa3-Fe3+-CN aa3-Fe3+-O2 methemoglobin–CN– complex. O2-binding site CN–-binding site O2-bind 27 V. OXIDATIVE PHOSPHORYLATION Mitochondrial electron transfer is linked to the generation of large amount of free energy, which is conserved in the form of the phosphate-bond energy of ATP; this process is called oxidative phosphorylation. NADH electron flow electron O2 transfer ENERGY oxidative ADP phosphorylation + Pi ATP 28 VI. OXIDATIVE PHOSPHORYLATION OMM IMS IMM MATRIX CHEMIOSMOTIC COUPLING An electrochemical gradient (protons, NADH I H +) is established by pumping H+ from H+ FP1 H+ the mitochondrial matrix to the inter- Q FPH2 membrane space; the electrochemical Electron Transfer H+ III H+ Respiration gradient is dissipated by coupling it to c the synthesis of ATP by ATP synthase or O2 H+ IV H+ Complex V or mitochondrial F1F0- H2O COUPLING ATPase which catalyzes: ADP + Pi → ATP + HO– ATP synthase works together with a Pi ADP phosphate carrier to aid the synthesis of Oxidative H+ Phosphorylation ATPase ATP from ADP. ATP 29 V. OXIDATIVE PHOSPHORYLATION Complex V include two components: F1 or coupling factor 1 or ATP-synthesizing unit, that catalyzes the synthesis of ATP. This unit consists of five kinds of polypeptide chains (a b g d e). F0 or proton-conducting unit, whose H+ role is to conduct protons. F0 is a hydrophobic segment that spans the inner mitochondrial membrane. The stalk between the F0 and F1 unit F1 matrix contains several other proteins, one of them renders the complex inner stalk membrane sensitive to oligomycin, an antibiotic intermembrane intermembrane F0 that blocks ATP synthesis by spacespace interfering with the utilization of the H+ gradient. H+ Kühlbrandt W (2015) BMC Biol 13:89 30 V. OXIDATIVE PHOSPHORYLATION Inhibitors and Uncouplers Oligomycin, an inhibitor of F1F0-ATPase, stops ATP synthesis and, because the latter is coupled to electron flow (respiration), it also stops respiration. An uncoupler of respiration and phospho- rylation dissipates the H+ gradient by transporting H+ from the intermembrane space to the matrix, thus short-circuiting the normal flow of H+ through the F1F0- OH NO2 ATPase. 2,4-Dinitrophenol (DNP), causes NO2 rapid respiration, which is not linked to ATP formation. DNP is used as a weigh loss strategy. 31 V. OXIDATIVE PHOSPHORYLATION Sites of Oxidative Phosphorylation OMM IMS IMM MATRIX H+ are pumped at three sites as electrons I flow through the respiratory chain from H+ FP1 Site I NADH to O2: Q Site I is Complex I H+ III Site II Site II is Complex III c Site III is Complex IV O2 H+ IV Site III H2O Each of these oxidation-reduction sites Pi generates enough energy to drive the ADP H+ synthesis of ATP under standard conditions ATPase ATP (DG°’ = –7.3 kcal/mol). 32 IMM V. OXIDATIVE MATRIX PHOSPHORYLATION MATRIX IMM IMM Electron Transfer Electron Transfer Acetyl-CoA Electron Transfer Acetyl-CoA Chain Sites of Oxidative Phosphorylation Chain and Energy Yields Chain Tricarboxylic Acid Cycle Tricarboxylic Acid Cycle Oxidative Phosphorylation dative Phosphorylation Oxidative Phosphorylation Generation of Reducing Equivalents Generation of Reducing Equivalents Gene Pyruvate DH a-Ketoglutarate DH OMM (NADH - FPH2) OMM (NADH - FPH2) NADH NADH NADH Malate DH NADH Isocitrate DH IMS MS Glutamate DH IMS Pyruvate Ketone Fatty Pyruvate Ketone Fatty bodies ADP + Piacids ADP + Pi ADP + bodies Pi acids H+ I H+ I H+ I Site I Site I Site ISite I ISite I SiteFADH pyruvate DH FADH pyruvate DH FADH2 pyruvate D 2 succinyl-CoA 2 succinyl-CoA NADH transferase NADH transferase ATP ATP ATP Succinate DH Q Q Q acetyl-CoA FPH2 Fatty acyl-CoA DH acetyl-CoA Glycerol-P DH citrate citrate ADP + Pi ADP + Pi H+ ADP + Pi H+ III Site II H+ III Site II III Site aconitase II Site II aconitase Site II ! Site II citrate synthase citrate synthase ! oxaloacetate isocitrate oxaloacetate isocitrate ATP Ascorbic NADHacid ATP NADH c ATP NADH c Ascorbic c acid + ADP +DH isocitrate Pi ADP + Pi + ADP + Piisocitrate DH H malate DH IV Site III H+ IV Site III Hmalate DH IV Site III Site III Site III Site III tricarboxylic NADH tricarboxylic NADH acid acid malat malate malate cycle ATP ATP cycle ATP ! -ketoglutarate ! -ketoglutarate 3 ATP 2 ATP ! -ketoglu- tarate DH 1 !tarate ATP -ketoglu- DH NAD-linked dehydrogenases FP-linked dehydrogenases Pi yield Electrons to cytochrome Pi NADH c Pi NADH ADP yield 3 ATPADP molecules fumarate 2 ATP ADP succinate DH molecules succinyl-CoA fumarate succinate DH yield ATPase 1 ATP succinyl-CoA H+ ATPase H+ ATPase H+ succinate succinate ATP ATP ATP FADH2 FADH2 ATP ATP 33 V. CLINICAL CORRELATIONS Hypoxic injury Deprivation of O2 to a tissue inhibits the coupling of electron transfer and oxidative phosphorylation, thus resulting in a decrease of cellular ATP levels (e.g., in myocardial infarction). An increase of anaerobic glycolysis, as a compen- satory mechanism to yield energy (ATP) and to maintain cellular functions: glycogen is depleted and lactic acid increases in cytosol, thus decreasing cellular pH. As the pH drops, lysosomal membranes are damaged and lysosomes release their hydrolytic enzymes (proteases, lipases, etc), which start an autolytic digestion of cellular components. Recovery of cells upon reperfusion with an O2- containing medium is possible, but the exact point-of- no-return at which a hypoxic cell becomes irre- versibly damaged is not known. 34 V. CLINICAL CORRELATIONS Uncoupling Proteins Brown adipose tissue plays role in non-shivering thermogenesis in new- borns. The primary agent involved in cold-induced thermogenesis is the uncoupling protein-1, UCP-1, that carries H+ back across the inner mitochon- drial membrane, thereby uncoupling ATP synthesis from electron transport. Cold is sensed by Activation of sympathetic nerves in brain results in release of hypothalamus norepinephrine, which binds the b-adrenergic receptor and leads to the sympathetic release of cAMP and activation of PKA. PKA stimulates the degradation of nerve triglycerides (lipolysis). The resulting fatty acids activate UCP1 and H+ are e translocated from the inter- phrin pine — nore brown membrane space back to the b-adrenergic adipose cell receptor matrix, thereby antagoniz- ing the H+ gradient that H+ supports ATP synthesis H+ H+ cAMP (complex V). This is the I mechanism of action of UCP III IV PKA drugs, such as Phentermine V H+ Triglycerides Fatty acid H+ (noradrenergic activator), lipolysis used to treat obesity. 35 ELECTRON-TRANSFER AND OXIDATIVE PHOSPHORYLATION Effects of UCP Activation OMM IMS IMM MATRIX pyruvate DH oxoglutarate DH isocitrate DH I NADH malate DH H+ FP1 H+ b-hydroxyacyl DH b-hydroxybutyrate DH succinate DH Q FPH2 fatty acyl CoA DH Glycerol-P DH H+ III H+ c O2 H+ IV H+ H2O UPC H+ Pi ADP fatty acids ATPase ATP ATP ATP biosynthesis neurotransmission VDAC ANT muscle contraction ADP 36 VI. MITOCHONDRIAL TRANSPORT SYSTEMS The outer mitochondrial membrane is freely permeable to most solutes, but the inner membrane is not: the inner membrane is impermeable to NAD+, NADH, NADP+, and NADPH, as well as other nucleotides, such as AMP, CTP, GTP, CDP, GDP, and to HS—CoA and acyl—SCoA. Outer Mitochondrial Freely Permeable to Metabolites Membrane Inner Selective Permeability Mitochondrial Impermeable to most metabolites Membrane Specific transport / carrier systems Intermembrane Several metabolites pass the inner Space mitochondrial membrane by the action of specific membrane transport systems, known carriers or translocases. These transport systems are highly specific and will not transport even closely similar molecules. 37 VI. MITOCHONDRIAL TRANSPORT SYSTEMS Glycerol-P shuttle Transport of Reducing Equivalents malate-aspartate shuttle Transport of Acetyl Units citrate-malate exchange Transport of Adenine Nucleotides 38 VI. MITOCHONDRIAL TRANSPORT SYSTEMS Transport of Reducing Equivalents CYTOSOL CYTOSOL NAD+ a. The Glycerol-P Shuttle NADH The glycerol-P shuttle translocates reduc- glycerol-P DH ing equivalents from cytosol to mitochondria. dihydroxy- glycerol-P NADH is formed by glycolysis in the cytosol, acetone-P in the oxidation of glycer-aldehyde-3-P. For IMM glycolysis to continue, NAD+ should be IMM regenerated. Electrons from NADH, rather dihydroxy- glycerol-P acetone-P than NADH itself, are carried across the inner glycerol-P DH MITOCHONDRIA mitochondrial membrane by means of the MITOCHONDRIA FP4 glycerol- 3-P shuttle. FP4H2 39 VI. MITOCHONDRIAL TRANSPORT SYSTEMS Transport of Reducing Equivalents a. The Glycerol-P Shuttle acetyl-CoA oxalo- citrate glyceraldehyde-3P glycerol-P glycerol-P acetate synthase citrate NAD+ FP4 NAD+ NADH NADH dihydroxy- dihydroxy- FP4H2 malate isocitrate 3P-glycerate acetone-P acetone-P NAD+ fumarase NADH pyruvate I fumarate a-ketoglutarate NAD+ succinate DH NADH Q FP2H2 succinate succinyl- FP2 CoA III c IV NADH + FP4 → NAD+ + FP4H2 cytosol mitochondria cytosol mitochondria Question How many ATP are produced by the glycerol-P shuttle? 40 VI. MITOCHONDRIAL TRANSPORT SYSTEMS Transport of Reducing Equivalents b. The Malate-Aspartate Shuttle The malate-aspartate shuttle translocates reducing equivalents from cytosol to mitochondria. This shuttle is mediated by two membrane carriers and four enzymes aspartate aspartate a-ketoglutarate a-ketoglutarate glutamate glutamate CYTOSOL oxaloacetate oxaloacetate MITOCHONDRIA NADH NADH NAD+ NAD+ malate malate 41 VI. MITOCHONDRIAL TRANSPORT SYSTEMS Transport of Reducing Equivalents b. The Malate-Aspartate Shuttle acetyl-CoA IMM glutamate a-ketoglutarate acetyl-CoA oxalo- citrate glyceraldehyde-3P acetate citrate glutamate NAD+ a-ketoglutarate glycerol-P glycerol-P FP4 synthase oxalo- aspartate aspartate oxalo- citrate de-3P acetate glycerol-P glycerol-P acetate synthase citrate NAD+ FP4 NADH NAD+ NADH NADH dihydroxy- dihydroxy- FP4H2 malate isocitra 3P-glycerate acetone-P acetone-P NAD+ NAD+ NADH dihydroxy- NADH NAD+ NADH malate dihydroxy- FP4H2 malate isocitrate te acetone-P acetone-P fumarase NAD+ NADH fumarase pyruvate I fumarate NADH a-ketoglut NAD+ succinate DH NADH I fumarate f a-ketoglutarate Q FP2H2 NAD+ succinyl- succinate DH FP2 NADH succinate CoA Q III FP2H FP2H22 succinate succinyl- FP2 FP2 CoA III c IV c NADH + NAD+ → NAD+ + NADH cytosol mitochondria cytosol mitochondria IV Question How many ATP are produced by the malate-aspartate shuttle? 42 VI. MITOCHONDRIAL TRANSPORT SYSTEMS fatty acids acetyl-CoA cholesterol Transport of Acetyl Units sterols oxalo- Citrate-malate exchange acetate citrate lyase NADH citrate The transport of acetyl units NAD+ malate DH outside the mitochondria involve three systems: acetyl-CoA malate citrate synthase oxalo- citrate acetate synthase citrate the tricarboxylate transporter NADH malate DH citrate lyase malate NAD+ isocitrate Acetyl-CoA is exported to cytosol NAD+ as citrate and returns to mitochon- NADH fumarate a-ketoglutarate dria as malate, an intermediate of NAD+ NADH the tricarboxylic acid cycle FADH2 succinate succinyl- FAD CoA 43 VI. MITOCHONDRIAL TRANSPORT SYSTEMS Transport of Adenine Nucleotides ATP and ADP do not diffuse freely across the inner mitochondrial membrane: a specific trans-port protein, the adenine nucleotide translocator (ANT) allows these molecules to traverse the inner membrane. ANT is specific for ATP and ADP: it cannot transport other nucleotide species (such as guanine, uridine, etc). The overall catalysis involves exchange of ADP –which is generated during energy-consuming reactions in the cytosol– for ATP –which is generated during oxidative phosphorylation in the mitochondria: ADPc + ATPm → ADPm + ATPc IMM ADP ADENINE NUCLEOTIDE TRANSLOCATOR ATP atractyloside Pi 44 THE ELECTRON-TRANSPORT CHAIN, ATPASE, AND ANT Synergistic Effects that Support Energy Transduction OMM IMS IMM MATRIX pyruvate DH oxoglutarate DH isocitrate DH I NADH malate DH H+ FP1 H+ b-hydroxyacyl DH b-hydroxybutyrate DH succinate DH Q FPH2 fatty acyl CoA DH Glycerol-P DH H+ III H+ c O2 H+ IV H+ H2O Pi ADP H+ ATPase ATP ATP ATP biosynthesis neurotransmission VDAC ANT muscle contraction ADP 45