Amino Acids - Chapter 3 PDF
Document Details
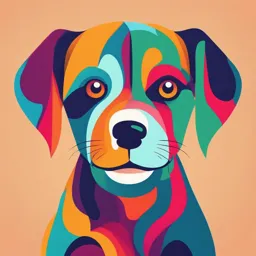
Uploaded by EarnestLake
Tags
Summary
This document offers a detailed overview of amino acids, including their biological functions, structures, and classifications. Topics covered include biochemical reactions, transport mechanisms, and structural roles in proteins.
Full Transcript
Amino Acids Proteins: Biological Functions - Biochemical Catalysis (Enzymes): Speed up chemical reactions in cells. - Binding Molecules for Storage & Transport: Transport molecules like oxygen (e.g., hemoglobin) or store nutrients. - Membrane Pores and Channels: Regulate the passage of molecules in...
Amino Acids Proteins: Biological Functions - Biochemical Catalysis (Enzymes): Speed up chemical reactions in cells. - Binding Molecules for Storage & Transport: Transport molecules like oxygen (e.g., hemoglobin) or store nutrients. - Membrane Pores and Channels: Regulate the passage of molecules in and out of cells. - Cell Structural Support and Shape: Provide structural integrity and maintain cell shape. - Mechanical Work: Contribute to muscle contraction and movement. - Regulation During Transcription & Translation: Control gene expression and protein synthesis. - Hormone and Signaling Molecules: Transmit signals for various cellular processes. - Receptors: Bind to specific molecules (ligands) and initiate cellular responses. - Specialized Functions (e.g., Antibodies, Toxins): Defend against pathogens or exert specific effects on cells. Proteins: Structure Informs Function - Polypeptide Chains Composed of Amino Acids: Long chains of amino acids linked by peptide bonds. - 20 Standard Amino Acids: Basic building blocks of proteins, each with unique properties. - Amino Acid Structure: Amino group (-NH2), carboxyl group (-COOH), and side chain (R group). - Systematic Names vs. Trivial Names: Each amino acid has a systematic name (e.g., 2-aminopropanoic acid for alanine) and a trivial name (alanine). Conformation vs. Configuration: - Conformation: Spatial arrangement due to rotation around single bonds, not requiring bond breakage. - Configuration: Spatial arrangement requiring bond breakage and reformation, as seen in stereoisomers. L-Amino Acid: - L-Amino acids are a type of amino acid commonly found in proteins. - The "L" designation indicates the configuration of the amino acid molecule. - L- and D- Comparison: - L-Amino acids have the amino group (-NH2) on the left side when written in Fischer projection. - D-Amino acids have the amino group on the right side in Fischer projection. Racemization: - Racemization is the conversion of one stereoisomer to its mirror image. - In the case of L-amino acids, racemization would convert them to D-amino acids or vice versa. - This process typically occurs under conditions that allow the rotation of bonds without breaking them, leading to a mixture of L- and D-forms. Amino Acid Categorization: - Amino acids are categorized based on their side chain composition and properties. - Side chain composition categories include aliphatic, aromatic, sulfur-containing, alcohols, positively charged, negatively charged, and amides. - Side chain properties categories include non-polar (hydrophobic), polar (hydrophilic), and charged. Aliphatic Amino Acids: - Aliphatic amino acids have side chains composed of aliphatic hydrocarbons. - They are nonpolar and hydrophobic, meaning they do not interact with water molecules. - Examples of aliphatic amino acids include glycine, alanine, valine, leucine, and isoleucine. - These amino acids are typically found in the interior of proteins, away from water-exposed surfaces. UV Protein Absorbance - UV (Ultraviolet) absorbance is a technique used to analyze the concentration and purity of proteins in a sample. - Proteins contain aromatic amino acids such as tryptophan, tyrosine, and phenylalanine, which absorb UV light at specific wavelengths. - The absorbance of UV light by proteins is primarily due to the electronic transitions within these aromatic amino acids. Wavelength vs. Absorbance Graph: - In a wavelength vs. absorbance graph for proteins, the x-axis represents the wavelength of UV light (usually in nanometers, nm), and the y-axis represents the absorbance value. - The graph typically shows a peak or peaks at specific wavelengths where the protein absorbs UV light most strongly. - The wavelength at which proteins absorb UV light most significantly depends on the specific aromatic amino acids present in the protein sequence. - For example, tryptophan has a strong absorbance peak around 280 nm, while tyrosine and phenylalanine also contribute to absorbance but at slightly higher wavelengths. - The height of the peak or the intensity of absorbance correlates with the concentration of proteins in the sample. Higher concentrations of proteins result in higher absorbance values at the characteristic wavelengths. Alcohol-Containing Amino Acids: - Amino acids with side chains containing alcohol (-OH) groups. - Examples include serine and threonine. Sulphur-Containing Amino Acids: - These amino acids contain sulfur in their side chains - Examples of sulfur-containing amino acids include cysteine and methionine. Cysteine: - Has a thiol (-SH) group in its side chain, which is highly reactive and forms disulfide bonds (S-S) with other cysteine residues. - Disulfide bonds play a crucial role in stabilizing protein structures, such as in the folding of proteins and the formation of protein complexes. - Cysteine is often involved in redox reactions, serving as a reducing agent by donating electrons. Methionine: - Contains a thioether group (-S-CH3) in its side chain. - Methionine is an essential amino acid, meaning it must be obtained from the diet as the body cannot synthesize it. - It plays a role in initiating protein synthesis as the start codon (AUG) codes for methionine in mRNA. - Methionine can also undergo post-translational modifications, such as methylation, which can influence protein function and regulation. - Positively Charged Amino Acids: - Amino acids with side chains containing positively charged amino groups (+NH3). - Examples include lysine, arginine, and histidine. - Negatively Charged Amino Acids: - Amino acids with side chains containing negatively charged carboxylate groups (-COO-). - Examples include aspartate and glutamate. Ionization of Side Chains: - The side chains of amino acids can undergo ionization, where they transition between protonated (acidic) and deprotonated (basic) forms depending on the pH of the environment. - Positively charged side chains gain a proton in acidic conditions, becoming neutral. - Negatively charged side chains lose a proton in basic conditions, becoming neutral. 1) Glutamate Side Chain: - Glutamate contains a carboxylic acid group in its side chain. When protonated (pKa = 4.1), it exists as the protonated form, which means it has a hydrogen ion (H+) attached to the oxygen of the carboxyl group. - At a lower pH below its pKa, the carboxylic acid group remains protonated. - As the pH increases above its pKa, the carboxylic acid group starts to deprotonate, forming the carboxylate ion. In this deprotonated form, the hydrogen ion dissociates, leaving behind a negatively charged carboxylate group (-COO-). 2) Arginine Side Chain: - Arginine contains a guanidinium group in its side chain. When protonated (pKa = 12.5), it exists as the protonated form, which means it has a hydrogen ion (H+) attached to the nitrogen atoms of the guanidinium group. - At a higher pH above its pKa, the guanidinium group starts to deprotonate, forming the guanidine group. In this deprotonated form, the hydrogen ions dissociate from the nitrogen atoms, resulting in a neutral guanidine group. - Amide Amino Acids: - Amino acids with side chains containing amide (-CONH2) groups. - Examples include asparagine and glutamine. - Other Amino Acids: - **Homoserine:** Derivative of serine. - **Homocysteine:** Derivative of cysteine. - **N-Formylmethionine:** Used as the initial amino acid in the synthesis of proteins in prokaryotes and in mitochondria of eukaryotes. It has a formyl group (-CHO) attached to the amino group. - **Selenocysteine:** An amino acid analogue of cysteine where the sulfur atom is replaced by selenium (-SeH). It is involved in certain enzymes, particularly those related to antioxidant functions. - **Pyrrolysine:** An uncommon amino acid found in certain archaea and bacteria, used as a building block in specific proteins. It contains a pyrroline ring as part of its side chain. Amino Acid Ionization: Amino acids undergo ionization due to their acidic (carboxyl) and basic (amino) groups, leading to different forms depending on the pH of the solution. - Amino acids typically have at least two pK a values, corresponding to the ionization of their acidic and basic groups. - The pK a of the carboxyl group (COOH) is around 2, while the pK a of the amino group (NH 2 ) is around 9. - At physiological pH (around 7.4), most amino acids have a net charge close to zero because both the carboxyl and amino groups are partially ionized but roughly balanced in their protonation states. - The isoelectric point (pI) of an amino acid is the pH at which it carries no net charge. It occurs when the acidic and basic groups are equally protonated and deprotonated, resulting in a zwitterionic form with no net charge. - Ionization of Alanine: - Alanine has a carboxyl group (COOH) with a pKa around 2 and an amino group (NH 2 ) with a pKa around 9. - At low pH (acidic conditions), the carboxyl group is protonated (-COOH), and the amino group is unprotonated (-NH 2 ). Alanine has a net positive charge in this form. - At high pH (basic conditions), the carboxyl group loses a proton and becomes deprotonated (-COO - ), while the amino group remains protonated (-NH 3 + ). Alanine has a net negative charge in this form. - At its isoelectric point (pI), which is the pH where it carries no net charge, alanine exists as a zwitterion (neutral form) with a protonated amino group (-NH 3 + ) and a deprotonated carboxyl group (-COO - ). - Ionization of Histidine: - Histidine has an additional ionizable side chain with a pK a around 6. - At low pH, the side chain is protonated (+NH 3 ), contributing to the overall positive charge of histidine. - At high pH, the side chain loses a proton (-NH 2 ), contributing to the overall negative charge of histidine. - At its isoelectric point (pI), histidine exists as a zwitterion with a protonated amino group (+NH 3 ) and a deprotonated carboxyl group (-COO - ), while the side chain may or may not be protonated, depending on its pK a and the specific pH. Isoelectric Point (pI) Calculation: - The pI is determined by the average of the pKa values of the acidic (carboxyl) and basic (amino) groups in the amino acid. The formula for calculating pI is pI = (pK 1 + pK 2 ) / 2, where pK 1 is the pK a of the carboxyl group and pK 2 is the pK a of the amino group. - For amino acids with ionizable side chains (e.g., histidine, lysine, arginine), there may be a third pK a value corresponding to the ionization of their side chains, leading to more complex pI calculations. - The Henderson-Hasselbalch equation is used to determine the ratio of ionized and non-ionized forms of an amino acid at a given pH. It is given by pH = pK a + log [A - ] / [HA], where [A - ] is the concentration of the conjugate base (deprotonated form) and [HA] is the concentration of the acidic form (protonated form) of the amino acid. Polypeptides: - Chains of linked amino acids, known as residues, where each residue can be identified by either its 3-letter code (e.g., Ala for alanine, Glu for glutamate) or its 1-letter code (e.g., A for alanine, E for glutamate). - Amino acids in a polypeptide chain lose most of their charges from their carboxyl and amino groups due to polymerization. The resulting charges mainly come from the side chains of the amino acids. - Polypeptides can be classified based on their length: - Di- and tri-peptides consist of 2 or 3 amino acids ex) Aspartame (dipeptide): A methyl ester composed of aspartate and phenylalanine - Commonly used as a sugar substitute due to its intense sweetness, being approximately 200 times sweeter than sucrose. - Oligo-peptides refer to chains with several amino acids - Poly-peptides are longer chains composed of many amino acids Protein Purification: 1. Accessing the Proteins: - This step involves breaking up cells through a process called lysis. Lysis releases the cell contents, including proteins, into a solution. 2. Separation from Other Molecules: - Once the proteins are released, they need to be separated from other cellular components like nucleic acids and membranes. - One common method for this separation is the addition of ammonium sulfate (a salt). This can cause proteins to precipitate out of the solution based on their solubility characteristics. 3. Individual Protein Separation Using Chromatography: - Chromatography is a powerful technique for separating individual proteins based on their chemical and physical properties. - Types of chromatography can be used depending on the specific properties of the target protein: - Ion exchange chromatography separates proteins based on their charge. - Size exclusion chromatography separates proteins based on their size. - Affinity chromatography uses specific interactions, such as antigen-antibody binding, to isolate proteins. 1. Types of Chromatography for Protein Purification: - Ion-Exchange Chromatography: Separates proteins based on their charge interactions with a charged stationary phase. - Reverse-Phase or Hydrophobic Interaction Chromatography: Separates proteins based on their hydrophobicity, with non-polar proteins binding to a hydrophobic stationary phase. - Gel Filtration Chromatography: Separates proteins based on their size, allowing smaller proteins to enter the pores of the gel while larger proteins pass through quickly. - Affinity Chromatography: Separates proteins based on specific interactions, such as antigen-antibody binding or receptor-ligand interactions. 2. Mechanism of Column Chromatography: - Column chromatography typically involves two phases: a static phase (stationary phase) and a mobile phase. - The static phase is a solid medium, such as beads or gel, that is packed into a column and remains stationary during the process. - The mobile phase is a liquid or gas that passes through the stationary phase, carrying the sample mixture. - Separation occurs as components in the sample interact differently with the stationary phase. For example, in ion-exchange chromatography, proteins with different charges interact differently with the charged stationary phase. 3. Methods of Mediating Separation: - Chromatography separations can be driven by different forces, including: - Gravity: Allows the mobile phase to flow through the column due to gravitational force. - Low Pressure (Peristaltic Pump, FPLC): Uses a pump to push the mobile phase through the column at a controlled rate. - High Pressure (HPLC): Employs high-pressure pumps to increase the flow rate and separation efficiency, commonly used in high-performance liquid chromatography (HPLC). HPLC (High-Performance Liquid Chromatography) system: 1. Solvent Reservoirs: Containers holding the mobile phase solvents, usually at high purity levels. 2. Solvent Degasser: Removes any dissolved gases from the mobile phase to ensure consistent flow and performance. 3. Gradient Valve: Controls the composition of the mobile phase, allowing for gradient elution where the solvent composition changes over time. 4. Mixing Vessel for Mobile Phase Delivery: Mixes the solvents according to the gradient program before delivering them to the column. 5. High-Pressure Pump: Generates the high pressure required to push the mobile phase through the column at a controlled rate. 6. Switching Valve: - (6) in "Inject Position": Directs the sample from the injection loop to the column for separation. - (6') in "Load Position": Allows for loading of the sample into the injection loop without flowing into the column. 7. Sample Injection Loop: Holds the sample to be analyzed or separated. Different loop sizes can be used for varying sample volumes. 8. Pre-Column (Guard Column): Protects the analytical column from contaminants and extends its lifespan by trapping impurities. 9. Analytical Column: The main column where separation of sample components occurs based on their interaction with the stationary phase. 10. Detector (e.g., IR, UV): Analyzes the eluent from the column to detect and quantify the separated components. 11. Data Acquisition: Collects and processes data from the detector, typically showing chromatograms with peaks corresponding to separated compounds. 12. Waste or Fraction Collector: Collects waste from the system or fractions of interest for further analysis or storage. = Simplified steps for HPLC: 1. Prepare pure solvents. 2. Mix solvents to create the mobile phase. 3. Pump the mobile phase through the column at high pressure. 4. Inject the sample into the column. 5. Separate components based on interactions with the column. 6. Detecting separated components. 7. Collect and analyze data. Ion Exchange Chromatography: - Principle: Ion exchange chromatography relies on the attraction between charged molecules in a sample and charged groups on the chromatography matrix. It separates molecules based on their charge properties. - Two types of ion exchange chromatography: - *Cation Exchange Chromatography:* Positively charged molecules (cations) are attracted to a negatively charged solid support (resin or beads). - *Anion Exchange Chromatography:* Negatively charged molecules (anions) are attracted to a positively charged solid support. - Mechanism: - *Mobile Phase:* Typically, a concentrated salt solution is used as the mobile phase. This salt solution helps optimize the binding of charged molecules to the chromatography matrix. - *Adsorption:* Charged molecules in the sample bind to the functional groups on the chromatography matrix (resin or beads). This binding is driven by ionic interactions between oppositely charged groups. - *Interaction Strength:* The strength of the interaction depends on factors like the number and location of charges on the molecule and the functional group on the matrix. - *Salt Gradient:* By increasing the salt concentration in a linear gradient, molecules with weaker ionic interactions start to elute (come off) from the column first. Molecules with stronger ionic interactions require a higher salt concentration and elute later in the gradient. - Elution: After binding, the molecules can be eluted from the column by changing the salt concentration in the mobile phase. This elution process allows for the separation of different charged molecules based on their interaction strengths with the matrix. Ion Exchange Chromatography Simplified Steps: 1. Uses polymer beads with charged groups. 2. Cation exchange attracts positively charged molecules; anion exchange attracts negatively charged ones. 3. Mobile phase is a salt solution. 4. Molecules bind based on ionic interactions with the beads. 5. Elution order depends on charge strength and salt concentration. 6. Cation exchange: Proteins with more negative charge elute earlier; Mobile phase A is water, and Mobile phase B is 5M NaCl. Hydrophobic Interaction Chromatography (HIC): A technique that separates molecules based on their hydrophobicity. - Principle: HIC relies on the hydrophobic interactions between molecules and a hydrophobic stationary phase (beads or resin) in the column. - Mobile and Stationary Phases: - *Mobile Phase:* The mobile phase in HIC is polar, typically water (H2O). It is aqueous and contains salts or buffers. - *Stationary Phase:* The stationary phase in HIC is non-polar. It consists of hydrophobic beads or resin packed in the column. - Mechanism: - *Adsorption:* When the sample containing hydrophobic molecules is injected into the column, hydrophobic molecules tend to adsorb (stick) to the hydrophobic beads in the column due to their affinity for non-polar environments. - *Elution:* Hydrophilic molecules, which do not interact strongly with the hydrophobic beads, pass through the column first and are eluted. This initial elution is achieved using the polar mobile phase (water). - *Increasing Hydrophobicity:* To elute hydrophobic molecules, the polarity of the mobile phase is gradually reduced. This is done by adding an organic solvent like methanol to the mobile phase, creating a gradient where the concentration of methanol increases over time (Mobile Phase B: 100% methanol). - *Elution of Hydrophobic Molecules:* As the hydrophobicity of the mobile phase increases, it reduces the hydrophobic interactions between the molecules and the beads. This allows the hydrophobic molecules to be eluted from the column in order of increasing hydrophobicity. - Gradient Elution: HIC often employs gradient elution, where the composition of the mobile phase changes over time from polar (water) to non-polar (methanol), facilitating the elution of molecules based on their hydrophobic properties. Size Exclusion Chromatography (SEC) (= Gel Filtration Chromatography): - Used for separating proteins based on the size and shape of molecules. - Utilizes porous beads in the column that allow smaller molecules to enter the beads and take longer paths, while larger molecules pass through more quickly by following the shortest paths through the column. - Small molecules get trapped in the beads and elute later, while larger molecules elute earlier. = Large molecules elute first - Doesn't require a mobile phase, operates under conditions close to physiological conditions, and is gentle on biomolecules. - Useful for desalting and buffer exchange in addition to size-based separations. - Generally, larger molecules elute first, followed by smaller ones. Affinity Chromatography: 1. Antibody-Antigen (Ab-Ag) Interaction: - Desired Protein: Antibody (Ab) - Binding Material: Antigen (Ag) specific to the antibody - Non-Binding Protein: Other proteins in the sample - Explanation: - Antibodies specifically bind to antigens they recognize. - The column contains immobilized antigens that the antibody can bind to. - Only the desired antibody binds to the antigen in the column, while other proteins pass through. - Non-binding proteins are separated from the antibody. 2. Lectin-Sugar Interaction → Lectin Affinity Chromatography: - Desired Protein: Lectin - Binding Material: Sugar (specific to the lectin) - Non-Binding Protein: Other proteins in the sample - Explanation: - Lectins bind to specific sugars or carbohydrates. - The column contains immobilized sugars that the lectin binds to. - Only the desired lectin binds to the sugar ligands in the column, while other proteins pass through. - Non-binding proteins are separated from the lectin. 3. Recombinant Protein-Tag Interaction: - Desired Protein: Recombinant protein with a specific affinity tag (e.g., His-tag, FLAG-tag) - Binding Material: Affinity resin with complementary binding sites for the tag - Non-Binding Protein: Other proteins in the sample - Explanation: - Recombinant proteins can be engineered with tags for easy purification. - The column contains resin with binding sites specific to the tag (e.g., nickel-charged resin for His-tag). - Only the desired recombinant protein with the affinity tag binds to the resin in the column. - Non-binding proteins pass through, allowing isolation of the bound recombinant protein. Lectin Affinity Chromatography: Lectin Affinity Chromatography works by using special molecules called lectins that can bind to specific sugars, like glucose. Glucose binding proteins will bind to the lectins on the beads: - Lectins on the chromatography beads can grab onto glucose-binding proteins due to their affinity for glucose. Glucose binding proteins are released when glucose is added into the mobile phase: - When glucose is added, it competes with the glucose-binding proteins for binding sites on the lectins. This competition causes the proteins to detach and be released. Lectin Affinity Chromatography is utilized to isolate the glucose binding protein from the mixture: - This technique is specifically designed to isolate proteins that bind to lectins, like the glucose-binding proteins. It helps separate and collect these proteins from a mixture. Ion Affinity Chromatography: a) GST (Glutathione-S-Transferase) Affinity Chromatography with Glutathione: - Desired Protein: GST-fused protein - Binding Material: Glutathione (GSH)-bound resin or beads - Elution Method: Competitive elution using excess free glutathione - Additional Step: Dialysis or buffer exchange after elution - Explanation: - The column is packed with resin or beads conjugated with glutathione. - The GST-fused protein binds specifically to the glutathione on the resin. - Excess free glutathione is added to the column during elution, competing with the bound GST-fused protein and releasing it. - The eluted protein is then dialyzed or buffer-exchanged to remove excess glutathione and salts, ensuring purity. b) Polyhistidine Affinity Chromatography with Imidazole: - Desired Protein: Polyhistidine (His-tagged) protein - Binding Material: Nickel or cobalt-charged resin or beads - Elution Method: Gradient elution using imidazole - Additional Step: Column regeneration for reuse - Explanation: - The column is loaded with resin or beads charged with nickel or cobalt ions. - The polyhistidine-tagged protein binds specifically to the metal ions on the resin. - Imidazole is used in a gradient elution to compete with the polyhistidine tag and release the bound protein. - After elution, the column is regenerated by washing with high concentrations of salt or chelating agents to remove any remaining bound proteins and prepare it for reuse. Gel Electrophoresis: 1. PAGE (Polyacrylamide Gel Electrophoresis): - Also known as "native" gel electrophoresis. - Separates molecules based on their charge and mass. - Useful for studying native protein structures and complexes. 2. SDS-PAGE (Sodium Dodecyl Sulfate-Polyacrylamide Gel Electrophoresis): - Utilizes the detergent SDS (Sodium Dodecyl Sulfate). - Denatures proteins by binding to hydrophobic side chains and giving them an overall negative charge. - Separates proteins primarily based on their molecular mass. - Ideal for analyzing protein composition and estimating molecular weights. Molecular Mass of Proteins: - Proteins are quantified based on their molecular mass, not weight. - Measurement units are typically in Daltons (Da) or kiloDaltons (kDa). - The term "molecular weight" (MW) is commonly used in biochemical contexts to refer to protein mass. SDS-PAGE steps: 1. **Buffer Preparation:** - A buffer solution, such as Tris-Glycine, is prepared to maintain the pH and ionic strength required for protein separation. 2. **Sample Preparation:** - Proteins are denatured and treated with SDS (Sodium Dodecyl Sulfate), which coats them uniformly with negative charges based on their mass. 3. **Loading the Gel:** - The SDS-treated protein samples are loaded into wells on an SDS-polyacrylamide gel, typically made by pouring acrylamide and bis-acrylamide monomers between glass plates. 4. **Electrophoresis:** - An electric current is applied to the gel, causing proteins to migrate through the gel matrix based on their size and charge. The negatively charged SDS-bound proteins move towards the positive electrode. 5. **Migration Direction:** - Proteins migrate from the sample lanes (wells) towards the anode (positive electrode). - The migration rate depends on the molecular weight of the proteins; smaller proteins move faster than larger ones. 6. **Separation Based on Molecular Weight:** - As proteins migrate through the gel, they separate according to their molecular weights. - Larger proteins encounter more resistance and migrate slower, while smaller proteins move through the gel more quickly. 7. **Staining and Visualization:** - After electrophoresis, the gel is stained with a dye, such as Coomassie Blue or Silver Stain, to visualize the separated proteins. - Stained bands on the gel correspond to different proteins based on their molecular weights. Mass Spectrometry: 1. Determining Molecular Mass: - Mass spectrometry is a technique used to determine the mass of molecules. It involves ionizing molecules, separating them based on their mass-to-charge ratio (m/z), and detecting these ions. 2. Ionization and Vaporization: - In mass spectrometry, molecules are ionized, typically by techniques like electrospray ionization (ESI) or matrix-assisted laser desorption/ionization (MALDI). This process converts molecules into ions by adding or removing charged particles. - The ionized molecules are then vaporized and introduced into the mass spectrometer. 3. Mass-to-Charge Ratio (m/z): - Once ionized, molecules travel through the mass spectrometer, where they are separated based on their mass-to-charge ratio (m/z). This ratio is determined by dividing the mass of an ion by its charge. 4. Time of Flight (TOF): - In time-of-flight mass spectrometry (TOF-MS), ions are accelerated by an electric field and then allowed to travel a known distance. The time taken for ions to reach the detector is proportional to their m/z ratio, allowing for mass determination. 5. Methods for Protein Analysis: - Electrospray Mass Spectrometry (ESI-MS): This method involves creating charged droplets of a protein solution through an electrospray, which then evaporates, leaving behind gas-phase protein ions that can be analyzed. - Matrix-Assisted Laser Desorption/Ionization (MALDI-MS): In MALDI-MS, a laser is used to ionize proteins embedded in a matrix material. This produces ions that are then analyzed based on their m/z ratios. - Tryptic fingerprinting: 1. Protein Digestion: - The first step in tryptic fingerprinting is the digestion of proteins into smaller peptides. This is typically achieved using an enzyme called trypsin, which cleaves proteins at specific amino acid sequences, usually after lysine (K) or arginine (R) residues. 2. Peptide Separation: - After digestion, the resulting mixture contains numerous peptides of varying lengths and sequences. These peptides are then separated using techniques like liquid chromatography (LC) before entering the mass spectrometer. 3. Mass Spectrometry Analysis: - In the mass spectrometer, peptides are ionized and fragmented using techniques such as collision-induced dissociation (CID) or higher-energy collisional dissociation (HCD). - The resulting peptide fragments, or ions, are then analyzed based on their mass-to-charge ratio (m/z) and fragmentation patterns. 4. Tryptic Fingerprint: - The term "fingerprint" refers to the unique pattern of peptide fragments produced by a protein after trypsin digestion and MS analysis. - Each protein generates a distinct tryptic fingerprint based on the specific amino acid sequence of the protein and the resulting peptide fragments observed in the mass spectra. 5. Database Searching: - To identify proteins based on their tryptic fingerprints, the observed peptide masses and fragmentation patterns are compared to databases of known protein sequences. Protein sequence analysis Amino Acid Analysis: - Acid-Catalyzed Hydrolysis: This method involves breaking down proteins into their constituent amino acids through hydrolysis under acidic conditions. Typically, hydrochloric acid (HCl) is used to cleave peptide bonds and release individual amino acids. - This process is often carried out in a controlled environment, such as a high-pressure reactor, to ensure complete hydrolysis without damaging amino acids. - The resulting amino acids are then analyzed using chromatographic techniques to quantify their amounts. - PITC Treatment + HPLC: PITC (phenyl isothiocyanate) treatment is a chemical derivatization method used to label amino acids for analysis. - In this method, PITC reacts with the amino groups of amino acids to form stable phenylthiocarbamyl (PTC) derivatives. - After derivatization, the amino acids are separated and quantified using High-Performance Liquid Chromatography (HPLC). - HPLC separates the labeled amino acids based on their properties such as size, polarity, and hydrophobicity, allowing for accurate quantification and identification. Protein Sequence Analysis: 1. Edman Degradation: - Treatment of the protein at pH = 9 with PITC (Phenylisothiocyanate), also known as the Edman reagent. - The Edman reagent reacts specifically with the free N-terminus of the protein to form a PTC-peptide. - The treated peptide is then subjected to anhydrous acid, which cleaves the N-terminal amino acid from the peptide chain. - This process can be automated using a sequenator, which sequences the amino acids one by one. - Edman degradation is typically used for sequencing shorter peptides rather than full-length proteins due to its sequential nature. 2. Chemical & Enzymatic Cleavage: - Chemical cleavage methods involve specific chemicals that break peptide bonds at specific amino acids. For example, cyanogen bromide (CNBr) cleaves at methionine residues. - Enzymatic cleavage involves using proteolytic enzymes like trypsin, chymotrypsin, or pepsin to cleave proteins at specific amino acid residues. - These cleavage methods generate fragments that can be sequenced individually, helping to piece together the entire protein sequence. 3. Codon Analysis: - Codon analysis is used for DNA or mRNA sequences to infer the corresponding protein sequence based on the genetic code. - By translating the DNA or mRNA sequence using the genetic code, one can determine the amino acid sequence coded by the gene. - This method is particularly useful for predicting protein sequences from genomic or transcriptomic data.