Gastrointestinal Motility PDF
Document Details
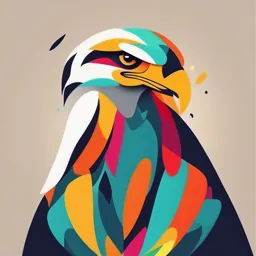
Uploaded by UnrealEinsteinium
Tags
Summary
This document discusses the gastrointestinal motility. It covers the general patterns of motility, including peristalsis and segmentation. It also goes into basic electrical activity and regulation of motility.
Full Transcript
Chapter 27 Gastrointestinal Motility The digestive and absorptive functions of the gastrointestinal system outlined in the previous chapter depend on a variety of mechanisms that soften the food, propel it through the length of the gastrointestinal tract (Table 27–1), and mix it with bile from the g...
Chapter 27 Gastrointestinal Motility The digestive and absorptive functions of the gastrointestinal system outlined in the previous chapter depend on a variety of mechanisms that soften the food, propel it through the length of the gastrointestinal tract (Table 27–1), and mix it with bile from the gallbladder and digestive enzymes secreted by the salivary glands and pancreas. Some of these mechanisms depend on intrinsic properties of the intestinal smooth muscle. Others involve the operation of reflexes involving the neurons intrinsic to the gut, reflexes involving the central nervous system (CNS), paracrine effects of chemical messengers, and gastrointestinal hormones. GENERAL PATTERNS OF MOTILITY PERISTALSIS Peristalsis is a reflex response that is initiated when the gut wall is stretched by the contents of the lumen, and it occurs in all parts of the gastrointestinal tract from the esophagus to the rectum. The stretch initiates a circular contraction behind the stimulus and an area of relaxation in front of it (Figure 27–1). The wave of contraction then moves in an oral-to-caudal direction, propelling the contents of the lumen forward at rates that vary from 2 to 25 cm/s. Peristaltic activity can be increased or decreased by the autonomic input to the gut, but its occurrence is independent of extrinsic innervation. Indeed, progression of the contents is not blocked by removal and resuture of a segment of intestine in its original position and is blocked only if the segment is reversed before it is sewn back into place. Peristalsis is an excellent example of the integrated activity of the enteric nervous system. It appears that local stretch releases serotonin, which activates sensory neurons that activate the myenteric plexus. Cholinergic neurons passing in a retrograde direction in this plexus activate neurons that release substance P and acetylcholine, causing smooth muscle contraction behind the bolus. At the same time, cholinergic neurons passing in an anterograde direction activate neurons that secrete NO and vasoactive intestinal polypeptide (VIP), producing the relaxation ahead of the stimulus. SEGMENTATION & MIXING When the meal is present, the enteric nervous system promotes a motility pattern that is related to peristalsis but is designed to retard the movement of the intestinal contents along the length of the intestinal tract to provide time for digestion and absorption (Figure 27–1). This motility pattern is known as segmentation, and it provides for ample mixing of the intestinal contents (known as chyme) with the digestive juices. A segment of bowel contracts at both ends, and then a second contraction occurs in the center of the segment to force the chyme both backward and forward. Unlike peristalsis, therefore, retrograde movement of the chyme occurs routinely in the setting of segmentation. This mixing pattern persists for as long as nutrients remain in the lumen to be absorbed. It presumably reflects programmed activity of the bowel dictated by the enteric nervous system, and can occur independent of central input, although the latter can modulate it. BASIC ELECTRICAL ACTIVITY & REGULATION OF MOTILITY Except in the esophagus and the proximal portion of the stomach, the smooth muscle of the gastrointestinal tract has spontaneous rhythmic fluctuations in membrane potential between about −65 and −45 mV. This basic electrical rhythm (BER) is initiated by the interstitial cells of Cajal, stellate mesenchymal pacemaker cells with smooth muscle-like features that send long multiply branched processes into the intestinal smooth muscle. In the stomach and the small intestine, these cells are located in the outer circular muscle layer near the myenteric plexus; in the colon, they are at the submucosal border of the circular muscle layer. In the stomach and small intestine, there is a descending gradient in pacemaker frequency, and as in the heart, the pacemaker with the highest frequency usually dominates. The BER itself rarely causes muscle contraction, but spike potentials superimposed on the most depolarizing portions of the BER waves do increase muscle tension (Figure 27–2). The depolarizing portion of each spike is due to Ca2+ influx, and the repolarizing portion is due to K+ efflux. Many polypeptides and neurotransmitters affect the BER. For example, acetylcholine increases the number of spikes and the tension of the smooth muscle, whereas epinephrine decreases the number of spikes and the tension. The rate of the BER is about 4/min in the stomach. It is about 12/min in the duodenum and falls to about 8/min in the distal ileum. In the colon, the BER rate rises from about 2/min at the cecum to about 6/min at the sigmoid. The function of the BER is to coordinate peristaltic and other motor activity, such as setting the rhythm of segmentation; contractions can occur only during the depolarizing part of the waves. After vagotomy or transection of the stomach wall, for example, peristalsis in the stomach becomes irregular and chaotic. MIGRATING MOTOR COMPLEX During fasting between periods of digestion, the pattern of electrical and motor activity in gastrointestinal smooth muscle becomes modified so that cycles of motor activity migrate from the stomach to the distal ileum. Each cycle, or migrating motor complex (MMC), starts with a quiescent period (phase I), continues with a period of irregular electrical and mechanical activity (phase II), and ends with a burst of regular activity (phase III) (Figure 27–3). The MMCs are initiated by motilin. The circulating level of this hormone increases at intervals of approximately 100 min in the inter-digestive state, coordinated with the contractile phases of the MMC. The contractions migrate aborally at a rate of about 5 cm/min, and also occur at intervals of approximately 100 min. Gastric secretion, bile flow, and pancreatic secretion increase during each MMC. They likely serve to clear the stomach and small intestine of luminal contents in preparation for the next meal. Conversely, when a meal is ingested, secretion of motilin is suppressed (ingestion of food suppresses motilin release via mechanisms that have not yet been elucidated), and the MMC is abolished, until digestion and absorption are complete. Instead, there is a return to peristalsis and the other forms of BER and spike potentials during this time. The antibiotic erythromycin binds to motilin receptors, and derivatives of this compound may be of value in treating patients in whom gastrointestinal motility is decreased. STOMACH Food is stored in the stomach; mixed with acid, mucus, and pepsin; and released at a controlled, steady rate into the duodenum. GASTRIC MOTILITY & EMPTYING When food enters the stomach, the fundus and upper portion of the body relax and accommodate the food with little if any increase in pressure (receptive relaxation). Peristalsis then begins in the lower portion of the body, mixing and grinding the food and permitting small, semiliquid portions of it to pass through the pylorus and enter the duodenum. Receptive relaxation is, in part, vagally mediated and triggered by movement of the pharynx and esophagus. Intrinsic reflexes also lead to relaxation as the stomach wall is stretched. Peristaltic waves controlled by the gastric BER begin soon thereafter and sweep toward the pylorus. The contraction of the distal stomach caused by each wave is sometimes called antral systole and can last up to 10 s. Waves occur 3–4 times per minute. In the regulation of gastric emptying, the antrum, pylorus, and upper duodenum apparently function as a unit. Contraction of the antrum is followed by sequential contraction of the pyloric region and the duodenum. In the antrum, partial contraction ahead of the advancing gastric contents prevents solid masses from entering the duodenum, and they are mixed and crushed instead. The more liquid gastric contents are squirted a bit at a time into the small intestine. Normally, regurgitation from the duodenum does not occur, because the contraction of the pyloric segment tends to persist slightly longer than that of the duodenum. The prevention of regurgitation may also be due to the stimulating action of cholecystokinin (CCK) and secretin on the pyloric sphincter. REGULATION OF GASTRIC MOTILITY & EMPTYING The rate at which the stomach empties into the duodenum depends on the type of food ingested. Food rich in carbohydrate leaves the stomach in a few hours. Proteinrich food leaves more slowly, and emptying is slowest after a meal containing fat (Figure 27–6). The rate of emptying also depends on the osmotic pressure of the material entering the duodenum. Hyperosmolality of the duodenal contents is sensed by “duodenal osmoreceptors” that initiate a decrease in gastric emptying, which is probably neural in origin. Fats, carbohydrates, and acid in the duodenum inhibit gastric acid and pepsin secretion and gastric motility via neural and hormonal mechanisms. The messenger involved is probably peptide YY. CCK has also been implicated as an inhibitor of gastric emptying (Clinical Box 27–2). VOMITING Vomiting is an example of central regulation of gut motility functions. Vomiting starts with salivation and the sensation of nausea. Reverse peristalsis empties material from the upper part of the small intestine into the stomach. The glottis closes, preventing aspiration of vomitus into the trachea. The breath is held in mid inspiration. The muscles of the abdominal wall contract, and because the chest is held in a fixed position, the contraction increases intra-abdominal pressure. The lower esophageal sphincter and the esophagus relax, and the gastric contents are ejected. The “vomiting center” in the reticular formation of the medulla (Figure 27– 7) consists of various scattered groups of neurons in this region that control the different components of the vomiting act. Irritation of the mucosa of the upper gastrointestinal tract is one trigger for vomiting. Impulses are relayed from the mucosa to the medulla over visceral afferent pathways in the sympathetic nerves and vagi. Other causes of vomiting can arise centrally. For example, afferents from the vestibular nuclei mediate the nausea and vomiting of motion sickness. Other afferents presumably reach the vomiting control areas from the diencephalon and limbic system, because emetic responses to emotionally charged stimuli also occur. Thus, we speak of “nauseating smells” and “sickening sights.” Chemoreceptor cells in the medulla can also initiate vomiting when they are stimulated by certain circulating chemical agents. The chemoreceptor trigger zone in which these cells are located (Figure 27–7) is in the area postrema, a V-shaped band of tissue on the lateral walls of the fourth ventricle near the obex. Lesions of the area postrema have little effect on the vomiting response to gastrointestinal irritation or motion sickness, but abolish the vomiting that follows injection of apomorphine and a number of other emetic drugs. Such lesions also decrease vomiting in uremia and radiation sickness, both of which may be associated with endogenous production of circulating emetic substances. Serotonin (5-HT) released from enterochromaffin cells in the small intestine appears to initiate impulses via 5-HT3 receptors that trigger vomiting. In addition, there are dopamine D2 receptors and 5-HT3 receptors in the area postrema and adjacent nucleus of the solitary tract. 5-HT3 antagonists such as ondansetron and D2 antagonists such as chlorpromazine and haloperidol are effective antiemetic agents. Corticosteroids, cannabinoids, and benzodiazepines, alone or in combination with 5-HT3 and D2 antagonists, are also useful in treatment of the vomiting produced by chemotherapy. The mechanisms of action of corticosteroids and cannabinoids are unknown, whereas the benzodiazepines probably reduce the anxiety associated with chemotherapy. SMALL INTESTINE In the small intestine, the intestinal contents are mixed with the secretions of the mucosal cells and with pancreatic juice and bile. INTESTINAL MOTILITY The MMCs that pass along the intestine at regular intervals in the fasting state and their replacement by peristaltic and other contractions controlled by the BER are described above. In the small intestine, there are an average of 12 BER cycles/min in the proximal jejunum, declining to 8/min in the distal ileum. There are three types of smooth muscle contractions: peristaltic waves, segmentation contractions, and tonic contractions. Peristalsis is described above. It propels the intestinal contents (chyme) toward the large intestines. Segmentation contractions (Figure 27–1), move the chyme to and from and increase its exposure to the mucosal surface. These contractions are initiated by focal increases in Ca2+ influx with waves of increased Ca2+ concentration spreading from each focus. Tonic contractions are relatively prolonged contractions that in effect isolate one segment of the intestine from another. Note that these last two types of contractions slow transit in the small intestine to the point that the transit time is actually longer in the fed than in the fasted state. This permits longer contact of the chyme with the enterocytes and fosters absorption COLON The colon serves as a reservoir for the residues of meals that cannot be digested or absorbed. Motility in this segment is likewise slowed to allow the colon to absorb water, Na+, and other minerals. By removing about 90% of the fluid, it converts the 1000–2000 mL of isotonic chyme that enters it each day from the ileum to about 200–250 mL of semisolid feces. MOTILITY OF THE COLON The ileum is linked to the colon by a structure known as the ileocecal valve, which restricts reflux of colonic contents, and particularly the large numbers of commensal bacteria, into the relatively sterile ileum. The portion of the ileum containing the ileocecal valve projects slightly into the cecum, so that increases in colonic pressure squeeze it shut, whereas increases in ileal pressure open it. It is normally closed. Each time a peristaltic wave reaches it, it opens briefly, permitting some of the ileal chyme to squirt into the cecum. When food leaves the stomach, the cecum relaxes and the passage of chyme through the ileocecal valve increases (gastroileal reflex). This is presumably a vago-vagal reflex. The movements of the colon include segmentation contractions and peristaltic waves like those occurring in the small intestine. Segmentation contractions mix the contents of the colon and, by exposing more of the contents to the mucosa, facilitate absorption. Peristaltic waves propel the contents toward the rectum, although weak antiperistalsis is sometimes seen. A third type of contraction that occurs only in the colon is the mass action contraction, occurring about 10 times per day, in which there is simultaneous contraction of the smooth muscle over large confluent areas. These contractions move material from one portion of the colon to another. They also move material into the rectum, and rectal distension initiates the defecation reflex (see below). The movements of the colon are coordinated by the BER of the colon. The frequency of this wave, unlike the wave in the small intestine, increases along the colon, from about 2/min at the ileocecal valve to 6/min at the sigmoid. TRANSIT TIME IN THE SMALL INTESTINE & COLON The first part of a test meal reaches the cecum in about 4 h in most individuals, and all the undigested portions have entered the colon in 8 or 9 h. On average, the first remnants of the meal traverse the first third of the colon in 6 h, the second third in 9 h, and reach the terminal part of the colon (the sigmoid colon) in 12 h. From the sigmoid colon to the anus, transport is much slower (Clinical Box 27–5). When small colored beads are fed with a meal, an average of 70% of them are recovered in the stool in 72 h, but total recovery requires more than a week. Transit time, pressure fluctuations, and changes in pH in the gastrointestinal tract can be observed by monitoring the progress of a small pill that contains sensors and a miniature radio transmitter.