Molecular Genetic Techniques Notes - Burman University
Document Details
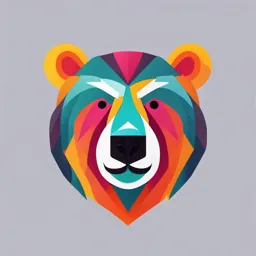
Uploaded by TenaciousNephrite186
Burman University
Tags
Summary
These notes provide an overview of molecular genetic techniques, including various types of mutations and their effects. They also discuss the use of recombinant DNA technology and various molecular biological techniques in different scientific fields. The notes are primarily for an undergraduate level biology course.
Full Transcript
Molecular Genetic Techniques Learning Objectives. After completing this chapter on Molecular Genetic Techniques, you will be able to: 1. Name the different types of mutations that occur in DNA, and how these nucleotide mutations can lead to alterations at the protein level. 2. Descri...
Molecular Genetic Techniques Learning Objectives. After completing this chapter on Molecular Genetic Techniques, you will be able to: 1. Name the different types of mutations that occur in DNA, and how these nucleotide mutations can lead to alterations at the protein level. 2. Describe how recombinant DNA technology is used to identify, clone, express and study (by the inactivation of) genes. 3. Describe the principles, methods, and applications of Northern, Southern, Western blot, microarray, PCR, DNA sequencing, and other molecular biological techniques, for biological, clinical, and forensic sciences. Textbook: Molecular Genetic Techniques, Chapter 6, Lodish et al. Verse: Genesis, Ch 1 and 2. The creation of life by God is described in Genesis 1 and 2. The natural biological laws of human reproduction ensures that human lives continue. Cloning is the transfer of genetic material, in some cases, to create life, by unnatural and artificial means. This chapter discusses the many molecular tools to achieve cloning. The subject of human cloning and issues that arise from this technology have prompted several Christian denominations, including the Seventh-day Adventist (SDA) Church, to outright condemn it - “Given our present state of knowledge and the current refinement of somatic cell nuclear transfer, the use of this technique for human cloning is deemed unacceptable by the Seventh-day Adventist Church. Given our responsibility to alleviate disease and to enhance the quality of human life, continued appropriate research with animals is deemed acceptable.” (the complete document is available at https://www.adventist.org/articles/ethical-considerations- regarding-human-cloning/) However, it has also left the door opened for “therapeutic cloning”. What do you think? That is a good question to ask yourself. To help you, I will direct you to an article written by two of my teachers at Loma Linda University, who have guided a lot of my thoughts on human cloning. Here I quote them “God endowed human beings with intelligence and creativity and gave us responsibility to cooperate with him in the care of the planet and all its creatures. He intends for us to grow in our understanding of the principles of life, including the function of our bodies. Ethical research and examination can only increase our appreciation of God's wisdom and goodness. ….. Within the medical realm, we are powerfully driven to control disease— conditions that disrupt the order and harmony that God intended. We are invited to use the knowledge he gives us. Consequently, gene therapy need not be an expression of human pride or arrogance. As long as the aim is to alleviate suffering, and we use our creativity with purpose, courage, caution, contingency, and compassion, keeping in mind the protection of the defenseless and helpless, genetic medicine has the same moral justification as traditional medicine. On the other hand, an attempt to redesign ourselves into creatures with new and LW2024 Burman University, BIOL 374 Cellular Biology, Genetic Molecular Techniques, Page 2 superlative powers would be perilous. A balanced view of our God-likeness should remind us that we tamper with fundamental human attributes at great risk.” (Anthony J. Zuccarelli and Gerald Winslow, 2002. The Test of Human Cloning. Spectrum, Vol. 30, pp. 25-40.) A lot to think about! 5.1 Genetic Analysis of Mutations to Identify and Study Genes § Widely accepted strategies are used to relate genes to their function, genomic location, and structure of gene products. All utilize common molecular biological/genetic techniques but have different starting points (F6-1): 1. In classical genetics, a mutant organism is first isolated to facilitate gene cloning from a genomic library, or from specific amplification of genomic DNA. Once the gene is cloned, it is used to produce the encoded protein for biochemical analysis. 2. Or, a purified protein with observable biochemical activity is first used to identify and isolate a gene. 3. Lastly, from a genomic sequence database (from completely sequenced genomes of organisms), sequence analysis leads to the identification of a protein-coding sequence. This putative gene can also be used to produce a purified protein. Additionally, if the gene sequence is confirmed to be a protein-coding sequence, it can be inactivated by various techniques and used to generate mutant cells or organisms. § An organism’s genotype is its entire set of genes and may denote whether an individual carries mutation(s) in one or more genes. An organism’s phenotype is its function and physical appearance and depends on that individual’s genotype. § Mutations are alterations in DNA sequences that result in changes in the structure of a gene. – Normal (wild type) organisms may develop mutations in their DNA sequence thereby altering their genotype and perhaps their phenotype. – Organisms that develop such mutations are termed “mutants”. – A mutation with a change in a single base generally affects the function of one gene. – At the DNA level, these single base changes result in: 1. Transitions – purine replaced by a different purine, or pyrimidine replaced by a LW2024 Burman University, BIOL 374 Cellular Biology, Genetic Molecular Techniques, Page 3 different pyrimidine. 2. Transversion – purine replaced by a pyrimidine, or pyrimidine replaced by a purine. 3. Indel – addition or deletion of one or more base pairs of DNA – At the protein level, point mutations lead to: 1. Synonymous or silent – no change in the amino acid sequence or activity of a gene’s encoded protein (i.e., alternate codon specifies the same amino acid). 2. Missense – conservative (codon specifies chemically similar amino acid) or nonconservative (codon specifies chemically dissimilar amino acids) substitutions. 3. Nonsense – codon signals chain termination. 4. Frameshifts – one-base-pair addition or one-base-pair deletion. § While most mutations in noncoding sequences may not be detrimental, it can affect gene expression if the mutated sequence is involved in gene control. § Spontaneous mutations (e.g., frameshifts) can occur during DNA replication. § Spontaneous mutations may also result from chemical instability of purine and pyrimidine bases, or from exposure to UV light or chemical carcinogens. For example, scientists often induce of point mutations by treatment of experimental organisms with EMS (ethyl methyl sulfoxide; a chemical mutagen), or exposure to ionizing radiation. § Chromosomal mutations or abnormalities are large scale changes in chromosomal structure that can affect several genes. These are inversions, deletions or insertions, and translocations. § Haploids and diploids – A haploid organism has a single copy of each chromosome, and its phenotype is a consequence of that one copy. – A diploid organism has two copies of each chromosome and thus two copies of each gene. § Alleles in a diploid organism – The two copies of each gene may be the same, or the copies may be different. Different forms of each gene are termed alleles. – Diploids that carry identical alleles are termed homozygous. – Diploids that carry different alleles are termed heterozygous. § The phenotypic expression of alleles in diploid organisms is dependent whether the alleles are recessive or dominant (F6-2). – Recessive mutations lead to a loss of function mutation, which is masked if a normal allele of the gene is present. – For mutant phenotype to occur, both alleles must carry the mutation. – Such mutations may remove part of the gene or the entire gene from the chromosome, disrupt expression of the gene, or alter the structure of the encoded protein, thereby altering its function. – Dominant mutations usually result in a gain of function mutation. LW2024 Burman University, BIOL 374 Cellular Biology, Genetic Molecular Techniques, Page 4 – Mutant phenotype in the presence of a normal copy of the gene is observed. – In a gain of function mutation, this mutation may increase the activity of the encoded protein, confer a new function on it, or lead to its inappropriate spatial or temporal pattern of expression. – A dominant mutation could also result in a loss of function mutation. § A haploinsufficient gene requires both alleles for normal function. – Removing or inactivating a single allele in such a gene leads to a mutant phenotype. – Some alleles can exhibit both recessive and dominant properties, e.g., Hbs. Homozygous Hbs/Hbs individuals suffer from sickle-cell anemia but heterozygous Hbs/Hba individuals do not, i.e., Hbs is recessive for the trait of sickle-cell anemia. However, heterozygous Hbs/Hba individuals are more resistant to malaria than homozygous individuals, i.e., Hbs is dominant for the trait of malaria resistance. § Segregation of Mutations – Dominant and recessive mutations exhibit characteristic segregation patterns in genetic crosses (F6-4). – Random segregation occurs during the first meiotic division yielding daughter cells with different mixes of paternal and maternal chromosomes. § Mitosis and Meiosis (F6-3). – Somatic cells divide by mitosis. – A diploid cell undergoes one DNA replication and two cell divisions. – The members of each pair of homologous chromosomes segregate independently during meiosis, leading to the random assortment of maternal and paternal alleles in the gametes. § Genetic Screens – The procedures used to identify and isolate mutants are termed “genetic screens”. – The screen used depends on whether the organism is haploid or diploid. – If diploid, the screen used also depends on whether the mutation is dominant or recessive. – Crosses between haploid cells can determine whether a mutant allele is dominant or recessive (e.g., segregation of alleles in yeast, F6-5). § Conditional Mutations – A mutation that has the wild-type phenotype under certain (permissive) environmental conditions and a mutant phenotype under restrictive, or non- permissive, conditions. – Screens with haploid organisms can involve temperature-sensitive (ts; or conditional) mutants, LW2024 Burman University, BIOL 374 Cellular Biology, Genetic Molecular Techniques, Page 5 e.g., in haploid yeast, Drosophila and C. elegans, temperature-sensitive mutants are particularly useful for identifying and studying genes essential for survival. – When ts mutants are maintained at permissive temperature, the mutant phenotype is not observed. Then for analysis, a subculture is incubated at the nonpermissive temperature where the mutant phenotype is observed. For example, ts genetic screen used to identify cell cycle mutants in yeast [F6-6a]. – ts mutants cannot be isolated in warm-blooded animals § Complementation Analysis – Used to determine whether different recessive mutations, associated with the same phenotype, are on the same gene. – Complementation is the restoration of wild-type phenotype by mating of two different mutations. – For example, in F6-7, if recessive mutations X and Y are in the same gene, then a diploid heterozygous for both mutations (i.e., carrying one X allele and one Y allele) will exhibit the mutant phenotype because neither allele provides a functional copy of the gene. – However, if recessive mutations X and Y are in separate genes, then heterozygotes carrying a single copy of each mutant allele will exhibit the wild- type phenotype because a wild-type allele of each gene will also be present (i.e., mutations are said to complement each other). § Ordering of Genes involved in Biosynthetic and Signaling Pathways – The order in which genes function in either a biosynthetic or signaling pathway can be deduced from the phenotype of double mutants defective in two steps of the affected process. – i.e., in the genetic dissection of pathways, a double mutant, with successive enzymes mutated, will clearly accumulate the intermediate immediately preceding the earlier step catalyzed by the wild- type proteins (F6-8a). – Double-mutant analysis is possible if two mutations, affecting the same process, have opposite effects on expression of a reporter gene. The observed phenotype of the double mutant provides LW2024 Burman University, BIOL 374 Cellular Biology, Genetic Molecular Techniques, Page 6 information about the order in which the proteins act and whether they are positive or negative regulators (F6-8bc). § Suppressor Mutation – A suppressor mutation is one that reverses the phenotypic effect of a second mutation, i.e., a “compensatory” mutation. – Can be used to identify genes encoding interacting proteins, e.g., observation that double mutants with two defective proteins (A and B) have a wild-type phenotype but that single mutants give a mutant phenotype indicates that the function of each protein depends on interaction with each other (F6-9a). § Synthetic Lethal Mutations – Produces a phenotype opposite that of suppression, i.e., the deleterious effect of one mutation is greatly exacerbated (rather than suppressed) by a second mutation in the same or related gene. – Can be used to reveal whether proteins interact with each other or if they are redundant (i.e., proteins with the same function). – Observation that double mutants have a more severe phenotypic defect than single mutants also is evidence that two proteins (e.g., subunits of a heterodimer) must interact to function normally (F6-9b). – Observation that a double mutant is nonviable, but the corresponding single mutants have wild-type phenotype indicates that two proteins function in redundant pathways to produce an essential product (F6-9c). LW2024 Burman University, BIOL 374 Cellular Biology, Genetic Molecular Techniques, Page 7 5.2 DNA Cloning and Characterization § DNA cloning permits scientists to prepare large numbers of identical DNA molecules. § A variety of techniques, often referred to recombinant DNA technology, are used in DNA cloning. § Recombinant DNA is simply any DNA molecule composed of sequences derived from different sources. § Two important discoveries in the 1970’s ushered in the era of DNA cloning, allowing one to "cut and splice" or recombine DNA, (hence, recombinant DNA technology): 1. Restriction endonucleases – “scissors” that cut DNA at specific sites. 2. DNA ligase – enzymatically joins 2 DNA fragments together. § In a typical DNA Cloning Scheme, steps: 1. Recombinant DNA molecules are formed in vitro by inserting DNA fragments of interest into vector DNA molecules. 2. “Recombined” DNA is introduced into host cells, where they replicate, producing large numbers of recombinant DNA molecules including the original DNA fragment. 3. Recombinant DNA molecules are isolated, sequenced and manipulated after it is purified in large quantities. 4. Cloned DNA is used in experiments, e.g., structure-function studies. A. Restriction endonucleases (RE) Bacterial enzymes that recognize specific 4- to 8-bp sequences, called restriction sites, cleaving both DNA strands at this site. Endonucleases cleave DNA within the molecule, exonucleases digest nucleic acids from an end. RE sites are inverted repeats (palindromic), i.e., restriction-site sequence is the same on each DNA strand when read in the 5’ à 3’ direction. e.g., for the EcoRI site (F6-11) 5’-GAATTC-3’ 3’-CTTAAG-5’ Most leave “sticky ends” or self-complementary single-stranded tails with 5’ overhangs or 3’ overhangs (e.g., EcoR1 digestion leaves 5’- overhangs). Some result in blunt (or flush) ends in which all the nucleotides at the fragment ends are base-paired, e.g., SmaI. RE's were discovered first in bacteria where they functioned to destroy (or restrict) incoming foreign DNA. RE Sites and Cut Frequency § The frequency a RE cuts DNA depends on the length of the restriction recognition site. § If the RE recognizes a 4-bp site, then it will cleave DNA an average of once every 44, or 256, base pairs. One that recognizes an 8-bp sequence will cut DNA once every 48 base pairs, or ~ 65 kb (4n, where n is length of recognition sequence, and 4 for the four possible bases - A, T, G and C). LW2024 Burman University, BIOL 374 Cellular Biology, Genetic Molecular Techniques, Page 8 § Often, these RE enzymes are named after the microorganism source (e.g., EcoRI form E. coli; HindIII from Haemophilus Influenzae strain D). How do bacteria prevent their own REs from destroying their DNA? § Host DNA is protected by a modification of its own DNA. § Modification enzymes, recognizing the same sites as the REs, methylate some of the nucleotides (A or G) inside that restriction site (right fig, old text). § This prevents the RE from cutting at a modified RE site. This is called the restriction- modification system. – Because DNA isolated from an individual organism has a specific sequence, REs cut the DNA into a reproducible set of fragments called restriction fragments. For example, in the fig (old text) below, the AD2 viral genomic DNA is digested with two different enzymes (EcoR1 and HindIII) that creates different restriction fragments. B. DNA Ligase – Catalyzes formation of 3’à5’ phosphodiester bonds between Okazaki fragments (in DNA replication). – Used to covalently link restriction fragments, DNA ligase requires a 3’-OH group at one end and a 5’- phosphate, along with ATP to fuel the enzyme. – Fragments that are “cut” with the same enzymes (compatible ends) are easily ligated. Blunt-end ligations require more DNA (100-1000 × higher). – To use DNA ligase to join incompatible ends, overhangs are first “filled-in”, or removed, to get blunt ends. – e.g., "filling-in" a 5' overhang can be done with Klenow (DNA polymerase lacking 3'à5' proofreading) to make a blunt end. The two blunt ends are then ligated. LW2024 Burman University, BIOL 374 Cellular Biology, Genetic Molecular Techniques, Page 9 C. Plasmid vectors – Plasmids are circular, extra-chromosomal, self- replicating dsDNA molecules (F6-13). – Plasmids occur naturally in bacteria and lower eukaryotes (e.g., yeast), existing in a parasitic or symbiotic relationship with their host cells. – E. coli plasmids are commonly engineered for use as cloning vectors (usually small, ~3 kb, with all nonessential genes removed). – Important components of a plasmid: 1. Replication origin (ORI) Specific region of 50-100 bp that must be present for plasmid DNA to replicate. Once DNA replication has begun, any foreign DNA in the plasmid will be replicated. 2. Drug resistance gene(s) Selectable gene/marker placed into a plasmid to select for bacteria harboring this plasmid. Most used is b-lactamase, which inactivates ampicillin (ampr). Others: tetr (tetracyclin) or kanr (Kanamycin) resistances. Some plasmids may contain more than one drug resistance gene. 3. Polylinker or multiple cloning site (MCS) Made from synthetic oligonucleotides composting of one copy each of several different restriction sites (not normally found in the vector). Many RE sites permits cloning of restriction fragments generated by cleavage of DNA with different REs. REs and DNA ligase allow the splicing of DNA fragments into cloning vectors (right top fig, old text). D. Transformation Genetic alteration of a cell caused by the uptake and expression of foreign DNA. After pretreatment of bacteria with CaCl2 or RbCl2, cells become competent (i.e., able to take up DNA). Typically, 1 out of 10,000 cells become competent, incorporating a single plasmid and thus becomes transformed. Finally, select for transformed bacteria, carrying the antibiotic-resistance gene, on an agar plate with antibiotics (F6-14). LW2024 Burman University, BIOL 374 Cellular Biology, Genetic Molecular Techniques, Page 10 E. Other Cloning Vectors – Plasmid vectors are relatively simple to use but limited by insert fragment size (20 kb maximum) and transformation efficiency. – Transformation efficiency also decreases as plasmid size increases. l Phage Structure – l Phage Vectors Bacteriophage l vectors can take inserts up to – Contains DNA 25 kb, permitting the efficient construction of – Infection large DNA libraries. of host cell Infection of E. coli host cells by l-phage occurs at ~1000-fold greater frequency than transformation (theoretically, need only one virus to infect a cell, and thus transform a cell). The genes for lysogenic growth are removed, leaving space for the inserted genes (right fig, old text). – Cosmids A cosmid (cos sites + plasmid = cosmids) is a type of hybrid plasmid that contains a l phage cos sequence required for the packing of l DNA. Basically, a circularized version of a l cloning vector. Cosmids vectors are designed to clone large fragments of DNA (~ 40-50 kb). The vector can be carried in a l phage (thus, all the advantages of a l phage) or grown in a bacterial host as a circularized plasmid. – BACs/YACs/HACs Acronyms mean bacterial artificial chromosome, yeast artificial chromosome and human artificial chromosome, respectively. These vectors contain selectable markers and ori (as in regular plasmids) but additionally contain CEN (centromeric; required for mitotic segregation) and TEL (telomeric; required for the replication and protection of the ends in linear DNA sequences) sequences, e.g., pYAC3 (Pearson Publishing, 2012). A restriction site(s) is used to insert the DNA fragment for cloning. These vectors can carry large, megabase- sized DNA fragments. These mini-chromosomes behave as stable chromosomes that are independent from the chromosomes of host cells (e.g., HAC from https://igtrcn.org/tool-for-making- synthetic-chromosomes/). LW2024 Burman University, BIOL 374 Cellular Biology, Genetic Molecular Techniques, Page 11 – Shuttle Vectors Most vectors are propagated in one host type. Shuttle vectors are those capable of propagation in two different hosts. e.g., Shuttle vectors that replicate in both yeast and E. coli can be used to construct a yeast genomic library (F6-15). The vector contains the basic elements (ORI, ampr and polylinker)that permit the cloning of DNA fragments in E. coli. The presence of a yeast origin of replication (ARS) and a yeast centromere (CEN) allows stable replication and segregation in yeast. A yeast selectable marker, URA3 (enzyme for uracil synthesis), which allows a ura3- mutant host harboring the vector to grow on medium lacking uracil. F. DNA Libraries 1. Genomic Libraries A set of l, or plasmid, clones that collectively contain every DNA sequence in the genome of a particular organism. Nearly complete genomic libraries of higher organisms can be prepared by l cloning (right fig, old text). A haploid human genome (3 x 109 bp) requires ~150,000 l phages. 2. cDNA Libraries cDNA libraries are made from complimentary DNA (hence, cDNA), reverse transcribed from mRNA (i.e., reverse transcriptase makes a DNA copy using an mRNA template; F6-17). A cDNA library represents all the mRNA expressed in a cell type. cDNA libraries, unlike the genomic library, lack introns. cDNA library should contain 106 – 107 individual recombinant clones to ensure slowly transcribed genes are included in these clones. LW2024 Burman University, BIOL 374 Cellular Biology, Genetic Molecular Techniques, Page 12 G. Screening DNA Libraries Two approaches are taken to isolate (or screen for) a DNA clone encoding a protein of interest from a collection of different clones, such as a genomic library or a cDNA library: 1. Detection by amplification of a specific DNA sequence with the polymerase chain reaction (PCR; see section H). 2. Detection based on functional complementation of the encoded proteins. § Specific genes can be isolated by their ability to complement the corresponding mutant genes in yeast cells. § i.e., only yeast colonies that carry recombinant plasmids expressing a copy of the wild-type copy of a mutated gene will be able to grow. This vector-borne wild-type gene complements, or reverses, the mutant phenotype (F6-16). Screening relies on several chemicals and background concepts: 1. Use of Oligonucleotides in molecular biology. a) Primers (~20 n’tides) are DNA sequences used for sequencing, PCR, and site-directed mutagenesis. b) Probes (> 20 n’tides) are short DNA/RNA sequences for probing/screening cDNA libraries, Southern Blots, etc. c) Synthesis of completely synthetic DNA (e.g., poly linker or MCS has the sequences of many restriction enzymes and are usually around 50-150 n’tides long). § The synthesized ssDNA (i.e., single-stranded DNA) is chemically identical to, and can be substituted for native DNA. § DNA is synthesized in the 3’à 5’direction (in vivo DNA replication is 5’ à 3’). § Automated machines called DNA synthesizers are used at a cost of < $1/base. § Oligonucleotide probes, to be synthesized, can be designed from information provided by protein sequencing or homology searches (i.e., sequence alignments) of genetic databases. § Since the genetic code is redundant, an amino acid may be coded by several codons, thus, to obtain a probe for with all the possible sequence iterations, a degenerate probe is created that is a mixture of all possible DNA sequences that encode the determined amino acid LW2024 Burman University, BIOL 374 Cellular Biology, Genetic Molecular Techniques, Page 13 sequence. This ensures that the probe mixture contains the one sequence exactly complementary to the gene of interest (fig above, old text). § For some procedures, the oligonucleotide is labelled with a radioactive (e.g., 32P-labeled phosphate), or fluorescent (e.g., fluorescein, rhodamine, etc), molecule for detection of the probe. 2. Nucleic Acid Hybridization § Hybridization is the ability of single-stranded DNA or RNA molecules to associate (or hybridize) specifically with complimentary DNA/RNA via base pairing (i.e., A=T and Gº C). § Denaturation of two strands of complementary nucleic acids can occur due to: 1. a temperature increase, which breaks the H-bonds), or 2. a pH increase (adding NaOH), which disrupts the H-bonding (OH- competes with bases for H-bonding sites). § If these conditions are reversed slowly, the individual H-bonding bases on the separated ssDNAs have time to find its complimentary sequence partner and renature (or reassociate or anneal) properly. § If reversed quickly, the ssDNA will form “self complementary tangles” (between complementary bases in the ssDNA). § Temperature is the preferred method. § e.g., Membrane-hybridization assay detects nuclei acids complementary to an oligonucleotide probe [F5-16, old text]. 3. Gel Electrophoresis § Resolves/separate DNA and RNA molecules in a gel matrix based on size (F5-19a, old text). § The mobility of either a ds- or ss-nucleic acid molecule during gel electrophoresis is inversely proportional to the logarithm of its length in nucleotides (i.e., the larger the molecule, the slower the mobility). § The size of a DNA molecule of unknown length can be determined by comparison to the electrophoretic migration of molecules of known length. § Different gels: a) Polyacrylamide gels are used for separation of nuclei acids up to 2000 n’tides. b) Most traditional method, Agarose gels, for fragments 500 n’tides to 20 kb. c) Pulsed-field gel electrophoresis separates large DNA molecules – 20 to 10,000 kb. LW2024 Burman University, BIOL 374 Cellular Biology, Genetic Molecular Techniques, Page 14 § Fragments separated by gel electrophoresis are visualized using Ethidium bromide + UV light. The fluorescent dye ethidium bromide intercalates between DNA bases, increasing intrinsic fluorescence when illuminated with UV light. H. PCR – The polymerase chain reaction (PCR) permits exponential amplification of a specific segment of DNA from just a single initial template DNA molecule if the sequence flanking the DNA region to be amplified is known (F6-18). – PCR can amplify a specific DNA sequence from a complex mixture of DNA because the reaction is based on the highly specific complementary base-pairing of template and primers. – The two flanking primers, 18-20 nucleotides long, are complementary to the 3’-end of the target DNA. – Primers are annealed to the target DNA by a cycle of heating (95 °C) and cooling (50-60 °C, close to the tm of the primer-template hybrid). – Uses a heat-stable DNA polymerase (Taq polymerase from Thermus aquaticus) that can survive up to 95 °C and can extend the primers at optimal temperatures of 72 °C. – The products undergo repeated doublings in an exponential fashion (amplification of 2n, where n is the number of PCR cycles). – Procedure is a highly sensitive method to detect nucleic acids, e.g., HIV virus, forensics, etc. – However, prone to false-positives due to contamination of DNA sample from exogenous sources. – PCR is commonly used as a rapid cloning tool for the direct isolation of specific segments of DNA, either from genomic or cDNAs libraries (F6-19). – Alternately, it can be used to generate longer probes for hybridization-based experiments, tagging of genes by insertion mutagenesis and mRNA expression profiles (RT-PCR). Short primers are first synthesized to flank the target sequence from which a probe is going to be generated. PCR probes are usually more efficient for hybridization because they are usually longer - 100 bp. Radioactive probes can also be generated when 32P- labeled dNTPs are included in the PCR reaction. I. The DNA sequence of cloned DNA fragments can be determined by various DNA sequencing protocols discussed in the laboratory exercise (see DNA sequencing, L3; note, you will still be responsible for that content). LW2024 Burman University, BIOL 374 Cellular Biology, Genetic Molecular Techniques, Page 15 5.3 Using Cloned DNA Fragments to Study Gene Expression Once you have a cloned piece of DNA fragment, it can be used for several experiments that can reveal the identity, function, temporal and spatial expression of its gene or gene products. Here are some common molecular biological techniques to facilitate that. A. Blotting Techniques § Southern blotting can detect a single, specific DNA fragment within a complex mixture by combining gel electrophoresis, transfer (blotting) of the separated bands to a membrane, and hybridization with a complementary labeled DNA/RNA probe (F6-24). § The similar technique of Northern blotting detects a specific mRNA within a mixture. § Expression of a particular gene can be followed by assaying the corresponding [mRNA] by Northern blotting. The temporal expression of a gene can also be performed if one is interested in the timing of gene expression in an experimental cell, or tissue. B. In Situ Hybridization § Northern blotting requires extracting the mRNA from a cell/tissue, which means that positional information (i.e., the location of cells relation to its neighbors) is lost. § In precise studies of gene expression, a whole or sectioned tissue, or even a whole organism (e.g., embryo) may be subjected to in situ hybridization to detect the (spatial) location of an expressed mRNA (F6-30). § If the detection of minor mRNA species is required, it may be coupled to a PCR step that would amplify single or low copy mRNA in the tissue section. This procedure is sometimes referred to in situ PCR. C. DNA Microarrays § DNA microarray analysis simultaneously detects the relative expression of thousands of genes in different types of cells or in the same cells under different conditions (i.e., complete transcriptional program). § A DNA microarray consist of thousands of individual gene LW2024 Burman University, BIOL 374 Cellular Biology, Genetic Molecular Techniques, Page 16 sequences (~ 1 kb) bound to closely spaced regions on the surface of a glass microscope slide. Some DNA microarray slides from different experimental organisms are available commercially (F6-31b). § Typically, total mRNA is isolated from experimental cultures, converted to cDNA before labelled with a fluorescent dye. If the experiment requires the differentiation of mRNA expression in two different conditions (or developmental stages), the cDNA probes created from the two different conditions are labelled with different coloured fluorescent dyes – allowing for differentiation of the signal (F6-26a). § A hybridized cDNA probe is captured by a complimentary gene sequence on the DNA microarray, identified by a fluorescent spot – which indicates the mRNA expression of that corresponding gene sequence. § The intensity of the spot can also indicate the level of mRNA expression. § Several, usually 2 different, coloured probes can be used simultaneously for the different conditions/developmental stages. A “housekeeping” gene is one that is expressed at all developmental stages of the cell, thus, would result in probes (of different colour) hybridizing to the same spot in the DNA microarray. § Cluster analysis of the data from multiple microarray expression experiments can identify genes that are similarly regulated under various conditions (i.e., regions A, B, C, etc; F6-32). § Such co-regulated genes commonly encode proteins that have biologically related functions. D. PCR-based Assays for Gene Expression 1. Quantitative PCR Quantitative PCR, or qPCR, or real-time PCR, can be adapted to estimate copy numbers of a particular sequence in a sample. PCR is carried out in the presence of a probe (i.e., primer) that emits a fluorescent signal when the PCR product is present (F9-13a, Lehninger). LW2024 Burman University, BIOL 374 Cellular Biology, Genetic Molecular Techniques, Page 17 If the sequence of interest is present at higher levels than other sequences in the sample, the PCR signal will reach a predetermined threshold faster (F9- 13b, Lehninger). Reverse transcriptase PCR (that converts mRNA sequences first to DNA; see below) and qPCR can be combined to determine the relative concentration of a particular mRNA concentration in a cell, hence, monitor gene expression. 2. Reverse Transcriptase PCR (or RT-PCR) The enzyme reverse transcriptase first converts mRNA to cDNA, which is then used as a template to synthesize dsDNA (double- stranded DNA). PCR primers are then used to amplify the DNA. Accumulation of a PCR product indicates the presence of mRNA in the original sample, hence, the expression of the gene for the mRNA. The amount of PCR product can also be used to estimate the level of gene expression. 3. in situ PCR Mentioned in section B, an RT-PCR is performed directly on a tissue slice mounted on a microscope. The presence of a PCR product in a specific location in the tissue indicate the spatial expression of mRNA at that location. E. Expression Vectors § Expression vectors derived from plasmids allow the production of abundant quantities of a protein of interest once a cDNA encoding it has been cloned. § This unique feature of the vectors is the presence of a promoter fused (i.e., joined) to the cDNA that allows high- level transcription in host cells (F6-33). § Even larger quantities of a desired protein can be produced in more complicated E.coli expression systems where high transcription is coupled to high translation systems. § Eukaryotic Expression Vectors – Eukaryotic expression vectors can be used to express cloned genes in yeast or mammalian cells. – Most can also function as shuttle vectors for cloning procedures in E. coli and expression purposes in mammalian cells, e.g., Dual Prokaryotic/Eukaryotic Expression Vector (many commercially available but here is one example from Vickers et al.). LW2024 Burman University, BIOL 374 Cellular Biology, Genetic Molecular Techniques, Page 18 F. Transfection § Transfection is a process to introduce cloned genes into cultured animal cells. § Cells can be transfected by: 1. Liposome-mediated – DNA is delivered in liposomes that fused with the cell membranes of animal cells. 2. Electroporation – cells are subjected to a brief electric shock of several thousand volts that makes them transiently permeable to DNA. § Transient Transfection (F6-34a) – Plasmid vectors (with viral ORI, polylinker, selectable marker and mammalian promoter adjacent to the cloned DNA) are not incorporated into the genome of the animal cell. – Production of the cDNA-encoded proteins occur if the animal cell has the plasmid. – Over time plasmids are lost from the cells because the plasmids are not faithfully segregated into daughter cells (hence, transient transfection). § Stable Transfection (F6-34b) – If an introduced vector integrates into the genome of the host cell, a stable transfection occurred. – Since the genome is permanently altered, the cell is said to be transformed. – A selectable marker, neor (which provides resistance to G-418, a chemical related to neomycin), is used to select for neor after several passages of cell culture when plasmids are lost from host cells. G. Retroviral Expression Systems § Retroviruses can integrate into host genomes with high efficiencies. § An example of such an expression system uses a class of retroviruses called the lentiviruses. Three different plasmids are introduced into cells by transient transfection to produce recombinant lentivirus particles that can efficiently introduce a cloned gene into target animal cells (F6-35). 1. Vector plasmid – The vector plasmid contains the cloned gene of interest next to a selectable marker such as neor flanked by lentiviral LTR sequences. – LTRs are required for integration into host chromosomes of the target cell. – LTRs direct the synthesis of recombinant viral RNA (i.e., sequence between the LTRs). LW2024 Burman University, BIOL 374 Cellular Biology, Genetic Molecular Techniques, Page 19 2. Packaging plasmid – Carries all the viral genes, except for the major viral envelope protein, necessary for the packaging of the LTR-containing viral RNA into a functional lentiviral particle. – Since it is lacking the viral envelop, this plasmid by itself cannot form an infectious viral particle. 3. Envelope plasmid – Plasmid controls the expression of a viral envelope protein which when incorporated into the recombinant lentivirus results in an infectious hybrid viral particle. – Often the envelope protein from vesicular stomatitis virus is used to replace the lentiviral envelope protein because it would allow the hybrid virus to infect a wide variety of mammalian cells, including hematopoietic stem cells, neurons, muscle and liver cells. H. Fusion Proteins as Tags, Epitopes and Protein Reporting § An important application is the tagging of proteins with a reporter protein (F6-36), or epitope for antibody detection, or protein purifications (bottom fig). § These tags are protein domains fused to the protein of interest. Some of these tags are removed after the fusion proteins are made (e.g., to facilitate the purification of a fusion protein). Purification of Fusion Proteins LW2024 Burman University, BIOL 374 Cellular Biology, Genetic Molecular Techniques, Page 20 5.4 Identifying and Locating Human Disease Genes § Inherited diseases and other traits in humans show three major patterns of inheritance - autosomal dominant, autosomal recessive, and X-linked recessive (T6-1; F6-26). § Autosome is one of the chromosomes that is not a sex chromosome. § Genes located on the same chromosome can be separated by crossing over during meiosis, thus producing new recombinant genotypes in the next generation (F6-10a). § Genes for human diseases and other traits can be mapped by determining their co-segregation with markers whose locations in the genomes are known. § The closer the gene is to a particular marker, the more likely they are to co-segregate (i.e., they are linked; F6- 10b). § Mapping of human genes with great precision requires thousands of molecular markers distributed along the chromosomes. § The most useful markers are differences in the DNA sequences (polymorphisms) among individuals in noncoding regions of the genome. § DNA polymorphisms useful in mapping human genes include restriction fragment length polymorphisms (RFLPs), single- nucleotide polymorphisms (SNPs), and simple tandem repeats (STRs; 2-4 base repeats). § Restriction fragment length polymorphism (RFLP) is a technique that exploits variations in homologous DNA sequences to distinguish individuals, populations, or species LW2024 Burman University, BIOL 374 Cellular Biology, Genetic Molecular Techniques, Page 21 or to pinpoint the locations of genes within a sequence (F5-36, old text). § Single-Nucleotide Polymorphisms (SNPs) can be followed like genetic markers (Old text; F6-33). § SNP and STR polymorphisms can be detected by PCR amplification and DNA sequencing. § Linkage mapping often can locate a human disease gene to a chromosomal region that includes as many as 10 genes (F6-28). § To identify the gene of interest within this candidate region typically requires expression analysis and comparison of DNA sequences between wild-type and disease-affected individuals. § Some inherited diseases can result from mutations in different genes in different individuals (genetic heterogeneity). § The occurrence and severity of other diseases depend on the presence of the mutant alleles of multiple genes in the same individual (polygenic traits). § Mapping of the genes associated with these diseases is particularly difficult because the occurrence of the disease cannot readily be correlated to a single chromosomal locus. 5.5 Inactivating the Function of Specific Genes in Eukaryotes § Once a gene has been cloned, important clues about its normal function in vivo can be deduced from the observed phenotypic effects of mutating the gene. § Basic strategies are utilized: 1. Replacing a normal gene with other sequence. 2. Introducing an allele whose encoded protein inhibits functioning of the expressed normal protein. 3. Destruction of the mRNA expressed from a gene. 4. Precise gene editing with CRISPR- Cas9 system. A. Disrupting Genes in Yeast (F6-37) – Genes can be disrupted in yeast by inserting a selectable marker gene LW2024 Burman University, BIOL 374 Cellular Biology, Genetic Molecular Techniques, Page 22 into one allele of a wild-type gene via homologous recombination, producing a heterozygous mutant. – When such a heterozygote is sporulated, disruption of an essential gene will produce two nonviable haploid spores. B. “Knockout” Mice – In mice, modified genes can be incorporated into the germ line at their original genomic location by homologous recombination, producing “knockouts” (or gene-targeted knockout). – Normal in vivo functions of genes can be deduced from the observed effects of disrupting it. – Mouse knockouts can provide models for human genetic disease. – Step 1: Production of “knockout” mice. Modified (or mutant) alleles are introduced by homologous recombination into embryonic stem (ES) cells. ES cells appear as the inner cell mass at the blastocyst stage of development. Preparation of ES cells carrying a knockout mutation (F6-37a; Old text). Isolation of ES cells with a gene-targeted disruption by positive and negative selection (F6-37b; Old text). – Step 2: Selection of “knockout” mice (F6-38; Old text). LW2024 Burman University, BIOL 374 Cellular Biology, Genetic Molecular Techniques, Page 23 The selected ES cells are injected into blastocoel cavity of early embryo of a mouse with different coat colour. Offsprings with chimeric coat colours are crossed to obtain ES-cell- derived progeny. – The loxP-Cre recombination system can knock out genes in one specific cell type (F6-39; Old text). C. Transgenic Animals – In the production of transgenic cells or organisms, exogenous DNA is integrated into the host genome by nonhomologous recombination (F6-40; Old text). – Introduction of dominant-negative allele in this way can functionally inactivate a gene without altering its sequence (F6-41; Old text). D. “Knockin” Mouse – To avoid the problems associated with a standard transgenic mice (i.e., transgene interrupting a gene), many researchers now rely on knockin mice to study the exogenous expression of a protein. – A knockin mouse is generated by targeted insertion of the transgene at a selected locus. The insert is flanked by DNA from a non-critical locus, and homologous recombination allows the transgene to be targeted to that specific, non-critical integration site. – In this way, a researcher has complete control of the genetic environment surrounding the overexpression cassette and it is likely that the DNA does not incorporate itself into multiple locations. – Site-specific knockins result in a more consistent level of expression of the transgene from generation to generation because it is known that the overexpression cassette is present as a single copy. LW2024 Burman University, BIOL 374 Cellular Biology, Genetic Molecular Techniques, Page 24 – Also, because a targeted transgene is not interfering with a critical locus, the researcher can be more certain that any resulting phenotype is due to the exogenous expression of the protein. – Disadvantage compared to transgenic mice, this procedure requires more time to assemble the vector and to identify ES cells that have undergone homologous recombination. E. RNA interference (RNAi) – In some organisms, double stranded RNA triggers the destruction of all mRNA molecules with the same sequence, but not that of mRNAs with a different sequence. – This phenomenon, known as RNAi, provides a specific and potent means of functionally inactivating genes without altering their structure. – First, double stranded RNA is created by using sense and antisense transcripts (F6-42a; Old text), or with a hairpin construct (F6-42c; Old text). – The dsRNA is microinjected into a cell to disrupt the production of the gene in question (F6-42b; Old text). – A specialized RNA-processing enzymes, called Dicer, cleave the injected dsRNA into short fragments, known as small inhibitory RNAs (siRNAs), which can base-pair with endogenous mRNA. – The resulting hybrid molecules are cleaved by another enzyme complex, called the RISC complex, thus destroying any mRNA that is complementary to the siRNA. F. Genome Editing – Site/oligonuleotide-directed Mutagenesis – In site-directed mutagenesis, a synthetic DNA segment replaces a fragment removed by REs. – In oligonucleotide-directed mutagenesis, a complimentary oligonucleotide with a specific sequence change is used to alter the cloned gene to be altered (F9- 10, Lehninger). LW2024 Burman University, BIOL 374 Cellular Biology, Genetic Molecular Techniques, Page 25 G. Genome Editing – CRISPR – The CRISPR (clustered regularly interspersed short palindromic repeats) mechanism is used by bacterial cells to protect against foreign DNA such as phage DNA. – Adapted to be used in many organisms, cleavage by CRISPR-Cas9 results in a short deletion at the cleavage site. – Cas (CRISPR-associated) 9 is the nuclease that performs the cleavage. – A chimeric guide RNA (gRNA) is expressed to provide the tracrRNA scaffold for binding to Cas proteins to initiate the cleavage (F6-38). – The chimeric gRNA includes a target sequence that guides the Cas nuclease complex to the specific target through base pairing. – Cas creates a double strand break at the target sequence in the genome; when repaired via a nonhomologous end joining process, the removal of some nucleotide bases inactivates the gene (due to a frameshift mutation). – If an appropriately designed DNA segment (HDR template – which spans the cleavage site) is introduced by transfection, specific DNA changes, such as point mutations, can be introduced at the cleavage site. LW2024