2024 Synaptic Transmission & Neurotransmitters PDF
Document Details
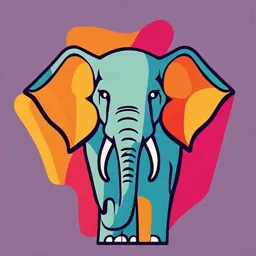
Uploaded by GratefulHyperbolic
Amerongen
2024
Todd Vanderah
Tags
Summary
This document is a set of lecture notes on synaptic transmission and neurotransmitters. Includes learning objectives covering topics like comparing and contrasting different synapses, and the synthesis and functions of neurotransmitters like acetylcholine, norepinephrine, dopamine, and serotonin. It also details the various types of receptors, and their actions.
Full Transcript
SYNAPTIC TRANSMISSION & NEUROTRANSMITTERS Block: Nervous System Block Director: Haley O'Brien, PhD Session Date: Monday, November 04, 2024 Time: 10:30 – 12:00pm Instructor: Todd Vanderah Department: Pharmacology Email: vanderah@email....
SYNAPTIC TRANSMISSION & NEUROTRANSMITTERS Block: Nervous System Block Director: Haley O'Brien, PhD Session Date: Monday, November 04, 2024 Time: 10:30 – 12:00pm Instructor: Todd Vanderah Department: Pharmacology Email: [email protected] INSTRUCTIONAL METHODS Primary Method: IM13: Lecture ☐ Flipped Session Resource Types: RE18: Written or Visual Media (or Digital Equivalent) INSTRUCTIONS Please read lecture notes and watch the podcast to prepare for an interactive session. READINGS N/A LEARNING OBJECTIVES 1. Compare and contrast the time course, and directionality of synaptic transmission at chemical and electrical synapses. 2. Determine the events that occur as the five basic steps of synaptic transmission at chemical synapses and understand the starting material as well as the rate-limiting enzyme in the synthesis of the major small-molecule transmitters in brain (acetylcholine, norepinephrine, dopamine, serotonin, GABA). 3. Outline the order of events associated with transmission at a chemical synapse, beginning with the arrival of an action potential at the presynaptic nerve terminal, and ending with the generation of a postsynaptic electrical event. 4. Decipher the mechanistic bases for formation of EPSPs and IPSPs, and discuss the concept of “integration” (summation) of these converging signals at the axon hillock; include a description of the differences between graded potentials and action potentials. 5. Compare and contrast the synthesis, processing, storage and termination of synaptic actions of the small molecule neurotransmitters and peptide neurotransmitters. 6. Compare and contrast neurotransmitter-gated ion channels and voltage-gated ion channels. 7. Evaluate the properties of G-protein-coupled receptors, kinase receptors, and nuclear receptors. 8. Summarize the basic mechanism by which commonly used local anesthetics inhibit neural transmission and their likely side effects. 9. Compare and contrast muscle relaxants (neuromuscular blockers) to spasmolytics (muscle relaxants) and be familiar with their side effects. 10. Determine the locations of brainstem neurons that give rise to diffuse modulatory projections using serotonin, dopamine, norepinephrine and acetylcholine. [Block: Nervous System | VANDERAH] [1 of 23] SYNAPTIC TRANSMISSION & NEUROTRANSMITTERS 11. Differentiate how changes in the levels of serotonin, dopamine, norepinephrine or acetylcholine might result in psychopathology, and describe the regions of the CNS in which there are correlations between psychopathology and changes in levels of these neurotransmitters. 12. Evaluate the type of receptors (GPCRs, ion channels, kinases, etc.) within the CNS that serotonin, dopamine, norepinephrine and acetylcholine interact with. 13. Summarize how medications alter the levels of serotonin, dopamine, norepinephrine and acetylcholine in ways that can be used to treat the psychopathology listed in #2. CURRICULAR CONNECTIONS Below are the competencies, educational program objectives (EPOs), disciplines and threads that most accurately describe the connection of this session to the curriculum. Related Related Competency\EPO Disciplines Threads COs LOs CO-01 LO-01 MK--03: The molecular, cellular and biochemical Neuroscience N/A mechanisms of homeostasis CO-01 LO-02 MK--03: The molecular, cellular and biochemical Neuroscience N/A mechanisms of homeostasis CO-01 LO-03 MK--03: The molecular, cellular and biochemical Neuroscience N/A mechanisms of homeostasis CO-01 LO-04 MK--03: The molecular, cellular and biochemical Neuroscience N/A mechanisms of homeostasis CO-01 LO-05 MK--03: The molecular, cellular and biochemical Neuroscience N/A mechanisms of homeostasis CO-01 LO-06 MK--03: The molecular, cellular and biochemical Neuroscience N/A mechanisms of homeostasis CO-01 LO-07 MK--03: The molecular, cellular and biochemical Neuroscience N/A mechanisms of homeostasis CO-06 LO-08 MK-06: The foundations of therapeutic intervention, Pharmacology N/A including concepts of outcomes, treatments, and prevention, and their relationships to specific disease processes CO-06 LO-09 MK-06: The foundations of therapeutic intervention, Pharmacology N/A including concepts of outcomes, treatments, and prevention, and their relationships to specific disease processes CO-01 LO-10 MK-02: The normal structure and function of the Neuroscience N/A body as a whole and of each of the major organ systems CO-02 LO-11 MK-05: The altered structure and function Pharmacology N/A (pathology & pathophysiology) of the body/organs in disease CO-01 LO-12 MK--03: The molecular, cellular and biochemical Neuroscience N/A mechanisms of homeostasis CO-06 LO-13 MK-06: The foundations of therapeutic intervention, Pharmacology N/A including concepts of outcomes, treatments, and prevention, and their relationships to specific disease processes [Block: Nervous System | VANDERAH] [2 of 23] SYNAPTIC TRANSMISSION & NEUROTRANSMITTERS Concepts within these notes (along the Review on Action Potential and Propagation lecture) are vital to understanding the function of the nervous system and how many medications and drugs of abuse affect the nervous system. Learning this will be very important in both medical practice and Step 1 of the USMLE board exam. The mechanism of action of many medications is dependent on learning neuronal synaptic communication since many medications will alter neurochemical interactions. [Block: Nervous System | VANDERAH] [3 of 23] SYNAPTIC TRANSMISSION & NEUROTRANSMITTERS INTRODUCTION The junction between two neurons is called a synapse. The synapse consists of a part of a presynaptic nerve terminal and a part of a postsynaptic cell neuron. Neurons communicate with each other through the process of synaptic transmission, in which Information is transferred from the presynaptic to the postsynaptic neuron. There are two general categories of synapses: electrical and chemical. ELECTRICAL SYNAPSES Figure 1, Electrical synapses formed by Electrical synapses involve a direct cytoplasmic connexon proteins allowing for continuity between adjacent cells at the point of bidirectional communication. Nolte’s The contact via specialized channels, called gap junctions Human Brain, 8th Ed. (Fig. 1). Electrical currents, generated by the activity of channels in the presynaptic cell are conducted to the postsynaptic cell by means of a direct flow of ions through the gap junction. The gap junction itself is a dodecameric complex of proteins, called connexins, which combine to form an aqueous pore that bridges the cytoplasmic compartments of the two cells. Electrical synapses allow for rapid and synchronous firing of interconnected cells. Information can flow either unidirectionally or bidirectionally and is typically excitatory. Electrical synapses are very important in coordinating the electrical activity of glial cells, Schwann cells, cardiac cells and some smooth muscle cells; however, they play a minor role in synaptic transmission in the mammalian central nervous system as compared to chemical synapse. The X-chromosome-linked genetic disease called Charcot-Marie-Tooth (CMT) delineates the importance of these gap junctions. CMT is a common hereditary peripheral neuropathy due to the demyelination of peripheral nerves with an incidence of 1 in 2000 individuals. Demyelination can result from a mutation in the connexin 32-protein hence forming a non-functional gap junction or a genetic mutation in the production of lipids that cause improper lipid-myelin formation. Patients often report a slow but usually progressive loss of both motor and sensory activity. Common clinical findings include muscle weakness, atrophy that starts more distal, high foot arches, “high stepping” to prevent tripping and palpable thickening of peripheral nerves. Table 1 CHEMICAL SYNAPSES Chemical synapses are the predominant class of synapses in the mammalian central nervous system. They are more complex than electrical synapses and offer more versatility for modifying synaptic transmission (Table 1). In chemical synapses, information is transferred from the presynaptic nerve terminal to the postsynaptic target cell through the release of neurotransmitters. A chemical synapse is [Block: Nervous System | VANDERAH] [4 of 23] SYNAPTIC TRANSMISSION & NEUROTRANSMITTERS separated by a small space called the synaptic cleft. A chemical synapse can mediate either excitatory or inhibitory actions and tend to be involved in behaviors that are more complex (e.g., learning and memory). Chemical synapses can amplify or modulate other neuronal signals. The synapse is the point of functional communication between two neurons or between neuron and target cell (i.e., gland, muscle, etc.). Unique synapses along an axon are referred to as varicosities (i.e., the neurons of the gastrointestinal tract), whereas a synapse at the end of an axon is referred to as a bouton (most prevalent in the CNS). Although there are approximately 100 billion nerves in the human central nervous system there are over 100 trillion synaptic connections. Over 100 chemical substances have been identified in the central nervous system and are thought to function as neurotransmitters. At one time, it was believed that each presynaptic nerve terminal released only one type of neurotransmitter molecule. This was referred to as “Dale’s Principle”. It is now known that a presynaptic nerve terminal in the central nervous system contains one predominant neurotransmitter, and typically several other minor neurotransmitters. The take home message is that more than one neurotransmitter may be released from a single neuron. Chemical neurotransmitters in the central nervous system fall into four broadly defined classes: 1. Typically, the major excitatory neurotransmitters—glutamate and aspartate. 2. Typically, the major inhibitory neurotransmitters - gamma-aminobutyric acid (GABA) and glycine. 3. Other small molecule neurotransmitters, including acetylcholine, and the “biogenic amines” including dopamine, norepinephrine, and serotonin. 4. Peptide neurotransmitters. The major excitatory and inhibitory neurotransmitters are involved in the rapid processing of information. Thus, synaptic actions of these substances often occur with a time course measured in milliseconds. In contrast, other small molecule neurotransmitters and peptide neurotransmitters often are more involved in modulatory actions. The synaptic actions of these substances may occur over a longer time course, measured from hundreds of milliseconds to seconds to minutes. Synapses can occur on different areas of the neuron (Fig. 2). 1. Axodendritic often excitatory 2. Axosomatic often inhibitory 3. Axoaxonic excitatory/inhibitory The proximity of a synapse to a trigger zone (axon hillock) of the postsynaptic cell is obviously important in determining its effectiveness. Hence, synaptic current generated at an axosomatic site has a stronger signal and therefore a greater influence on the outcome at the trigger zone than current from the more remote axodendritic contacts. There are five basic steps involved in the process of chemical Figure 2. Various forms of Synaptic Contact. synaptic transmission: Principles of Neuroscience, Kandel, et al. 4th ed. 2000. [Block: Nervous System | VANDERAH] [5 of 23] SYNAPTIC TRANSMISSION & NEUROTRANSMITTERS 1. Synthesis of the neurotransmitter within the presynaptic neuron. 2. Storage of the neurotransmitter within synaptic vesicles in the presynaptic nerve terminal. 3. Release upon depolarization, and diffusion across the synaptic cleft. 4. Interaction of the neurotransmitter with specific receptors located on the membrane of the postsynaptic cell. 5. Termination of the synaptic actions of the neurotransmitter. SYNTHESIS Small molecule neurotransmitters are often amino acids themselves (e.g., glutamate, aspartate, glycine), or are derived from amino acids. A neuron that uses a given molecule as a neurotransmitter must possess specific enzymes that synthesize the neurotransmitter from common precursors. Such enzymes may thus serve as specific “markers” for neurons that use the particular neurotransmitter. Acetylcholine is synthesized in cholinergic nerve terminals by the enzyme choline acetyltransferase (Fig.3). This enzyme uses the substrates acetyl coenzyme A (an activated acetate Figure 3. Acetylcholine Synthesis. group that is formed through a variety of common metabolic reactions) and choline (ubiquitous small molecule found in many foods we eat) to form acetylcholine (Fig. 3). The catecholamines dopamine and norepinephrine are synthesized from the common amino acid tyrosine. The first, and rate-limiting step, of this process is catalyzed by the enzyme tyrosine hydroxylase, which converts tyrosine to DOPA. DOPA is then converted to dopamine by the enzyme DOPA decarboxylase (Fig. 4). Both enzymes are present in neurons that use dopamine and norepinephrine as neurotransmitters. Administering DOPA, thus bypassing the rate limiting tyrosine hydroxylase step in catecholamine synthesis, can augment the synthesis of dopamine. This is one pharmacological strategy for the treatment of Parkinson’s disease, in which levels of dopamine in the target areas for dopamine-containing neurons are low (Parkinson’s Disease along with the drugs used to treat will be discussed in more detail later in the course). Norepinephrine- containing neurons have one additional enzyme, dopamine beta-hydroxylase, which converts Figure 4. Catecholamine Synthesis. dopamine to norepinephrine. [Block: Nervous System | VANDERAH] [6 of 23] SYNAPTIC TRANSMISSION & NEUROTRANSMITTERS The indolamine serotonin is synthesized from the common amino acid precursor tryptophan. The first and rate-limiting step of this process is catalyzed by the enzyme tryptophan hydroxylase, which converts tryptophan to 5-OH- tryptophan. This latter compound is converted to serotonin, by a second enzyme called L-aromatic acid amino acid decarboxylase (Fig. 5). The common inhibitory neurotransmitter GABA is synthesized from the amino acid glutamate, by the enzyme glutamic acid decarboxylase (Fig. 6). For all purposes there is Figure 5. Serotonin Synthesis. no need to memorize any chemical structures or enzymatic products, yet what you should know is the starting products of the neurotransmitters and the rate limiting or first enzyme in the synthesis of the above neurotransmitters. The numerous peptides that act as neurotransmitters (e.g., enkephalins, endorphins, cholecystokinin, GnRH, etc.) are synthesized, like all proteins/peptides, from the nucleus through the Figure 6. GABA Synthesis. common transcription and translation processes and imported into vesicles as they pass through the Golgi apparatus (Fig. 7). Figure 7. Peptide Neurotransmitter Synthesis occurs directly from the nucleus of the neuron where it goes through transcription and translation packaged into vesicles and transported to the end of the neuron. (Fundamental Neuroscience, MJ Zigmond et al., 1999) STORAGE AND RELEASE OF NEUROTRANSMITTERS [Block: Nervous System | VANDERAH] [7 of 23] SYNAPTIC TRANSMISSION & NEUROTRANSMITTERS Neurotransmitters are stored within numerous small membrane bound vesicles within the presynaptic nerve terminal and travel both anterograde (from cell body to the ends of the axon and dendrites) and retrograde (from ends of axon and dendrites to cell body) via the microtubule and special protein carriers (Fig. 8). Often small molecule neurotransmitters are re-synthesized in the cytoplasm of the presynaptic nerve terminal or within the vesicle themselves. Small neurotransmitter molecules can be transported into the synaptic vesicle by specific transporter proteins located in the membrane of the synaptic vesicle. Each synaptic vesicle contains 2000-5000 molecules of neurotransmitter. Inhibitors of this vesicle transport process result in a depletion in the amount of neurotransmitter. For example, the drug reserpine (Raudixin® used for hypertension) prevents the transport of catecholamines and indolamines into synaptic vesicles, and as a result, lowers the levels of dopamine, norepinephrine and serotonin in their respective presynaptic nerve terminals. The storage of neurotransmitter molecules in synaptic Figure 8. Life Cycle of Neurotransmitters and their vesicles protects them from degradation by transport to-and-from the cell body. catabolic enzymes, provides a mechanism of Neurotransmitters use special proteins and the transport to the neuronal end and provides a microtubule system to move to the ends of the mechanism that enables the transmitter to be nerve and back rapidly. Special transporters at the released upon depolarization of the presynaptic end of the axon also recycle small molecule nerve terminal. neurotransmitters. (Fundamental Neuroscience, MJ Zigmond et al., 1999) In addition to the transporter proteins, the presynaptic vesicles contain several additional proteins. There are proteins that; 1) sense the concentration of Ca2+, 2) hold the vesicle in place for release and 3) proteins that are involved in the docking, release and uptake of the vesicle within the presynaptic nerve terminal. Voltage-dependent Na+ and K+ channels are present in the axon, where they mediate the ion fluxes required for action potential propagation. However, these channels are not present in the presynaptic nerve terminal membrane. When an action potential reaches the presynaptic terminal, the resulting wave of depolarization spreads throughout the terminal membrane by 2+ electrotonic conduction. The resulting depolarization causes activation of voltage-gated Ca 2+ channels (VGCC) located in the presynaptic terminal membrane. Thus, Ca ions flow down 2+ their electrochemical gradient into the presynaptic terminal, raising intra-cytoplasmic Ca concentrations (Fig. 9). Many of the synaptic vesicles are present in a zone very close to the presynaptic terminal 2+ membrane, called the active zone. When intra-cytoplasmic Ca concentrations increase, the 2+ 2+ Ca binds to special Ca sensing proteins on the synaptic vesicles resulting in the synaptic vesicles in the active zone to fuse with the membrane of the presynaptic nerve terminal, leading to the exocytotic release of neurotransmitter into the synaptic cleft. This process is very rapid, requiring only several hundred microseconds to occur and is called exocytosis. The complement of neurotransmitter molecules within a single synaptic vesicle is called a quantum, and fusion of individual vesicles with the presynaptic nerve terminal membrane results in quantal release of the neurotransmitter. Hence, many medications can either alter the levels of calcium that will influence neurotransmission or in some cases act to inhibit the release of the vesicles. [Block: Nervous System | VANDERAH] [8 of 23] SYNAPTIC TRANSMISSION & NEUROTRANSMITTERS Figure 9. As the action potential reaches the axon terminal, the depolarization activates the opening of voltage sensitive calcium channels. Calcium triggers the vesicle with neurotransmitter to fuse with the membrane and release its contents into the synapse (exocytosis). (Reproduced from Principles of Neuroscience, ER. Kandel, et al. 4th ed. 2000) Once neurotransmitter is released into the synapse, the neurotransmitter diffuses across the synaptic cleft to reach receptors located on the membrane of the postsynaptic target neuron. The process of diffusion across the synaptic cleft introduces a delay of 0.3 to 1 msec associated with chemical synaptic transmission. Once the neurotransmitter is released into the synaptic cleft there is a process of vesicular reuptake back into the presynaptic nerve terminal called endocytosis. Endocytosis often involves several proteins including clathrin (a protein that marks the vesicle for endocytosis) and dynamin (a GTPase-calcium dependent protein necessary for pinching off the vesicle from the presynaptic membrane). NEUROTRANSMITTER RECEPTORS Neurotransmitters exert their physiological effects by acting on specific receptors located on the postsynaptic neuron or target cell. Generally speaking, receptors for neurotransmitters can be classified into two groups, Ligand Gated Channels and 2nd Messenger-linked receptors (Fig. 10). Ligand gated channels, by definition, are channels allowing ions to flow through a pore of the receptor. The 2nd Messenger-linked receptors, by definition, do not allow ions to flow through the receptor directly but can activate proteins within the cell that may have multiple effects including indirectly affecting nearby ion channels, activate Figure 10. Classification of Receptors. Pharmacology, Rang et kinase proteins and can even alter DNA al., 5th ed. 2003) [Block: Nervous System | VANDERAH] [9 of 23] SYNAPTIC TRANSMISSION & NEUROTRANSMITTERS transcription (Fig. 10). Furthermore, the 2nd Messenger-linked receptors can be subdivided into three types of receptors termed, 1) G-protein receptors, 2) the kinase family of receptors and 3) the nuclear receptors (Fig. 10). Remember that the activation of second messenger-coupled receptors may result in the permeability of the postsynaptic cell membrane to specific ions but as the name suggests are indirect via an intracellular second messenger that may open or close selective ion channels. Activation of the receptor by the neurotransmitter may result in a variety of changes in the physiology of the postsynaptic cell. Immediate effects include changes in the permeability of the postsynaptic membrane to specific ions. Longer-term effects include release of calcium ions from internal stores, changes in the activity of a wide variety of enzymes and even regulation of the transcription and translation of specific proteins in the postsynaptic cell. Thus, neurotransmitters may cause changes that may be manifested anywhere from milliseconds to days following receptor activation. Any given neurotransmitter may have many different types and subtypes of receptors associated with it (Remember from Foundations, drugs have more than one effect and sometimes this is due to their activity at multiple receptors). These receptors are defined by their pharmacological properties, i.e., by the actions of different agonists and antagonists on the receptor (more detail on receptors below). An agonist is a drug that mimics the effects of the neurotransmitter in causing receptor activation. An antagonist is a drug that blocks or prevents the activation of the receptor by the neurotransmitter. ELECTROPHYSIOLOGICAL SEQUELAE OF NEUROTRANSMITTER RECEPTOR ACTIVATION Immediate effects of ligand-gated receptor activation include changes in the permeability of the postsynaptic cell membrane to specific ions, resulting in a change in its membrane potential. For example, if the binding of a neurotransmitter to its receptor were to result in an increase in the permeability of the membrane to Na+ ions, Na+ ions would flow into the cell down their electrochemical gradient, resulting in a depolarization of the postsynaptic cell membrane. This depolarization is called an excitatory post- synaptic potential (EPSP) and is referred to as a graded potential, not an action potential. Activation of a neurotransmitter receptor resulting in an increase in the permeability of the membrane 2+ Figure 11. Demonstration of excitatory (a), and to Ca ions would similarly produce depolarization inhibitory graded potentials (b). Within the leading to an EPSP (Fig. 11a). nervous system these type of graded potentials Alternatively, if the activation of a receptor by are integrated by the post-synaptic neuron (c). The integration by the post-synaptic cell the neurotransmitter were to result in an increase in determines the level of excitability of the post- the permeability of the membrane to K+ ions, K+ synaptic neuron. ions would flow out of the cell down their chemical gradient. Similarly, if activation of a receptor by the [Block: Nervous System | VANDERAH] [10 of 23] SYNAPTIC TRANSMISSION & NEUROTRANSMITTERS neurotransmitter were to result in an increase in the permeability of the membrane to Cl- ions, Cl- ions would flow into the cell down their chemical gradient. Either of these ion fluxes would result in hyperpolarization of the postsynaptic cell membrane, this hyperpolarization is called an inhibitory post-synaptic potential (IPSP) and again is referred to as a graded potential, not an action potential (Fig. 11b). Individual EPSPs or IPSPs produce only “small” perturbations of the postsynaptic cell membrane potential, on the order of several millivolts. This is a consequence of the fact that relatively few “quanta” of transmitter are released into the synaptic cleft with each synaptic event, so that relatively few ion channels in the postsynaptic cell membrane are activated. Thus, one EPSP seldom results in sufficient postsynaptic cell depolarization to move the cell membrane potential to threshold, to cause the initiation of a self-propagating action potential. If the cell is to be excited to threshold, it generally requires EPSP input from many different cells, and/or repeated excitatory input from a few cells. The concepts of convergence and integration are key to understanding how information is processed through synaptic transmission. A neuron in the CNS typically has hundreds to thousands of synaptic interactions with other neuronal cells whose electrical signals converge on the target cell. The postsynaptic cell membrane, upon receiving all the excitatory and inhibitory inputs from hundreds of different discrete synaptic events, integrates all this activity, summing together all the excitatory and inhibitory input (Fig. 11c). The membrane potential locally rises and falls, depending on the preponderance of depolarizing and hyperpolarizing activity and the electrotonic spread of individual EPSPs and IPSPs. The most excitable region of the postsynaptic cell is the axon hillock, most often the region of the cell between the cell body and the axon. The axon hillock is an area of the neuron that contains a high number of voltage sensitive sodium channels making it a very excitable region. Thus, the axon hillock becomes a key site for integration (summation) of all the synaptic input. Both temporal summation and spatial summation can occur (Fig. 12). Temporal summation is the process by which consecutive synaptic potentials at the same site are added together by the post- synaptic neuron. Spatial summation involves integration of input from many presynaptic neurons acting at different sites on the post-synaptic neuron. When sufficient excitatory input is received, the threshold potential of the axon hillock is reached, resulting in the creation of a regenerative action potential that propagates down the axon. This Figure 12. Temporal (time) (repeated stimulation action potential spreads down the axon (to synapse of a neuron) and Spatial (more than one input). with another cell), as well as back across the cell Summations are important in determining the body and dendrites. This latter process effectively excitability of the post-synaptic neuron. “wipes the slate clean”, allowing the process of integration to begin again. Because of the asymmetry of the synapse (i.e., vesicles containing neurotransmitter are found only in the presynaptic neuron and not the postsynaptic cell), transmission of signals across chemical synapses for the most part is unidirectional. Also, an individual synaptic event can be either depolarizing (excitatory) or hyperpolarizing (inhibitory). Whether a particular transmitter is excitatory or inhibitory depends on the nature of the neurotransmitter receptor located on the [Block: Nervous System | VANDERAH] [11 of 23] SYNAPTIC TRANSMISSION & NEUROTRANSMITTERS postsynaptic neuron, the ion channel permeability changes linked to receptor activation and the postsynaptic membrane potential prior to the release of neurotransmitter. While some transmitters are almost always excitatory (e.g., glutamate) and some are almost always inhibitory (e.g., GABA), other transmitters may be either excitatory or inhibitory at different synapses depending on the receptor the neurotransmitter interacts with. Presynaptic release of neurotransmitter can be influenced by other presynaptic neurons (e.g., axoaxonic synapse). For example, a GABA containing neuron that synapses onto another GABA neuron can result in excitation as a result. This type of double inhibition is referred to as synaptic disinhibition. TERMINATION OF NEUROTRANSMITTER SYNAPTIC ACTIONS Neurotransmitters may be removed from the synaptic cleft by one of three processes, diffusion, reuptake back into the presynaptic nerve terminal, or enzymatic catabolism. Diffusion The simple diffusion of the neurotransmitter into the synaptic cleft allows for a dilution and termination of the neurotransmitter. All neurotransmitters undergo diffusion. Reuptake The synaptic actions of glutamate, aspartate, GABA, glycine, norepinephrine, dopamine and serotonin are primarily terminated by the reuptake process (Fig. 13). These transmitters are pumped back into the presynaptic nerve terminal against a concentration gradient by specific transport proteins located within the presynaptic nerve terminal membrane. The transmitter returned to the presynaptic cytoplasm can again be stored in vesicles,and be used again and again for neurotransmission. There are several drugs that can act at these reuptake transporters, blocking reuptake and causing the neurotransmitter to remain in the synapse and therefore have a longer Figure 13. Termination of neurotransmitter is effect. For example, the antidepressant drugs such mediated by three methods including simple as the tricyclic drugs (TCAs) block the reuptake of diffusion within the synapse, by reuptake into norepinephrine and/or serotonin. The common the presynaptic neuron and glial cells through selective transporters and by selective TCAs include amitriptyline (Elavil), imipramine enzymes that degrade the neurotransmitters. (Tofranil) and desipramine (Norpramin). MAO = monoamine oxidase, COMT = The design of Selective Serotonin Reuptake catechol-O-methyl transferase, AChase = Inhibitors (SSRI) have gained ground in the acetylcholine esterase. treatment of depression due to their reduction in side-effects. The most common SSRI antidepressant includes fluoxetine (Prozac), paroxetine (Paxil), and sertaline (Zoloft) all selectively blocking the reuptake of serotonin. Drugs of abuse can also target these reuptake transporters. Cocaine can block the reuptake of norepinephrine and dopamine hence creating an increase in concentration of catecholamines in the synapse. Amphetamines are thought to be taken-up via these transporters resulting in interactions at the vesicle transporters ultimately enhancing release of catecholamines from the presynaptic terminal initially and continual use eventually results in depletion of catecholamine neurotransmitters. [Block: Nervous System | VANDERAH] [12 of 23] SYNAPTIC TRANSMISSION & NEUROTRANSMITTERS Metabolism The synaptic actions of acetylcholine are primarily terminated by enzymatic hydrolysis (Fig. 13). The enzyme acetylcholinesterase cleaves acetylcholine into acetate and choline. At the neuromuscular junction, acetylcholinesterase is located on the postsynaptic membrane of the motor endplate. In the central nervous system, acetylcholinesterase is located on the presynaptic nerve terminal. The choline produced by acetylcholinesterase is often taken back up into the presynaptic nerve terminal, where it is reused for acetylcholine synthesis. There are a number of chemicals that inhibit acetylcholinesterase that have been used during war-time (nerve gas, SARIN) and are currently found in many of the common pesticides (DIAZINON). Although the synaptic actions of biogenic amines (dopamine, norepinephrine and serotonin) are mainly terminated by the reuptake process, these transmitters are also influenced by the metabolic processes that degrade these transmitters into pharmacologically inactive substances. Both dopamine and norepinephrine are metabolized by the sequential actions of two enzymes—monoamine oxidase (MAO) and catechol-O-methyltransferase (COMT). MAO inhibitors have been used as antidepressants as well but are less tolerable due to side effects. The MAO inhibitors include drugs like phenelzine (Nardil) and selegiline (Eldepryl). The final metabolite of dopamine is homovanillic acid (HVA), whereas the final metabolite of norepinephrine is 3-methoxy-4-hydroxyphenylglycol (MHPG). The amounts of these metabolites produced provide a rough index of the amount of neurotransmitter released. Thus, HVA levels are an index of the activity of dopamine containing neurons, whereas MHPG levels are an index of the activity of norepinephrine containing neurons. Similarly, serotonin is metabolized by the actions of monoamine oxidase, to form 5- hydroxyindole acetic acid (5-HIAA). The amount of 5-HIAA present is a rough index of the activity of brain serotonin containing neurons. Metabolism of Peptide Neurotransmitters A wide variety of hormones originally identified in the periphery are now recognized to function as neurotransmitters in the central nervous system as well. These include hypothalamic releasing hormones (i.e., thyroid releasing hormone, somatostatin), various gastric hormones (vasoactive intestinal peptide, gastrin), posterior pituitary hormones (vasopressin, oxytocin), and endogenous opioids (enkephalins, endorphins). There are similarities and differences in how the brain uses peptide neurotransmitters as compared to small “classical” neurotransmitters. Whereas small “classical” neurotransmitters are synthesized in the presynaptic nerve terminal from common precursors by specific enzymes, peptide neurotransmitters are usually synthesized in the cell body from specific mRNAs in the rough endoplasmic reticulum, packaged in large synaptic vesicles, and transported down the axon into the presynaptic nerve terminal (Fig. 7). When released, these peptide transmitters interact with specific receptors located on the postsynaptic cell. The synaptic actions of peptide neurotransmitters are usually terminated by enzymatic hydrolysis by either exopeptidases (which cleave terminal amino- or carboxyl-terminal amino acids) or endopeptidases (which cleave amide bonds in the interior of the peptide molecule) present in the synaptic cleft. Peptide transmitters are often present in presynaptic nerve terminals in 1000-fold lower molar concentrations than small “classical” neurotransmitters. NEUROTRANSMITTER RECEPTORS; ASPECTS OF ACTIVATION As mentioned above, there are two broad classes of receptors—those that are themselves ion channels called neurotransmitter-gated ion channels or ionotropic, and are thought to act within milliseconds. The other class of receptors exerts their effects through the formation of “second messengers” called metabotropic receptors, and act on the order of hundreds of milliseconds. [Block: Nervous System | VANDERAH] [13 of 23] SYNAPTIC TRANSMISSION & NEUROTRANSMITTERS Neurotransmitter-gated ion channels These receptors are themselves ion channels located within the cell membrane. Activation of this type of receptor by the neurotransmitter causes a conformational change, leading to the immediate opening or closing of an ion channel (Fig. 10). Examples include: Nicotinic cholinergic receptor: This is the receptor for acetylcholine that is present on muscle cells at the motor endplate. Motor neurons innervating skeletal muscle use acetylcholine as the neurotransmitter. Activation of this receptor by acetylcholine causes a conformational change in the nicotinic receptor located on the motor endplate of the muscle cell, leading to an increase in the permeability of the membrane to cations. As a result, the depolarization of the muscle cell membrane leads to the generation of an action potential, ultimately causing muscle contraction. Nicotinic channels are also found in the Autonomic Nervous System (ANS) between the pre- and post-synaptic neurons of both the sympathetic and parasympathetic divisions. They are made up of 5 subunits that form a cation pore. These channels are different in their subunit composition when compared to the nicotinic receptors in the neuromuscular junction. Likewise, unique nicotinic channels are also found throughout the Central Nervous System (CNS). NMDA receptor: This is a receptor for the amino acid neurotransmitter glutamate. It is called the NMDA receptor because the compound N-methyl-D-aspartate can act as an agonist and mimic the effects of glutamate at this receptor. The NMDA channel is special in that it needs both glutamate and a slight depolarization of the membrane potential to be activated. When glutamate has activated the NMDA channel, the channel pore opens allowing Na+ and Ca+2 ions to flow into the cell. These cations flow down their electrochemical gradient into the cell, leading to depolarization of the cell membrane. These receptors are found throughout the central nervous system and play an important role in learning and memory. GABAA receptor: This is a receptor for the neurotransmitter GABA. When activated by GABA, this receptor’s ion channel undergoes a conformational change, leading to an increase in the permeability of the membrane to Cl- ions (Fig. 14). As a result, these anions flow down their chemical gradient into the cell, leading to hyperpolarization of the cell membrane. These receptors throughout the CNS play an important role in many sedative and antiepileptic drugs that we will discuss later in the course. One property of neurotransmitter-gated ion channels is the rapidity of their synaptic actions. Figure 14. GABAA receptors are CL-channels Because only a conformational change in the allowing CL- to flow into the neuron resulting in receptor is required for changes in ion hyperpolarization. permeability, the electrophysiological sequelae of receptor activation occur within milliseconds. Receptors Linked to Second Messenger Formation [Block: Nervous System | VANDERAH] [14 of 23] SYNAPTIC TRANSMISSION & NEUROTRANSMITTERS With neurotransmitter-gated ion channels, only a non-enzymatic conformation change is necessary to induce physiological effects. In contrast, activation of receptors linked to the formation of second messengers results in a cascade of biochemical events. In these cases, receptor activation causes the synthesis of a biologically active compound in the interior of the cell. This compound acts through various mechanisms to exert effects in multiple areas. There are several families of second messenger linked receptors including those Figure 15. When a neurotransmitter (first linked to cyclic AMP, phosphatidylinositol and messenger) binds the receptor a conformational phospholipase A2 via G-proteins, kinase change in the receptor activates an intracellular G- protein that can result in the activation of a number of receptors that auto-phosphorylate each other different effectors (second messengers). Ultimately and receptors that can enter the nucleus and the activation of a G-protein coupled receptor results modulate gene transcription called nuclear in a cascade of events. (Pharmacology Rang et al., 5th receptors. ed. 2003) G-Proteins as Transducers of Receptor Activation Receptors linked to intracellular proteins and enzymes do not exert their effects on second messengers directly, but rather act through an intermediate set of proteins called G-proteins (Fig. 15). These proteins are so named because they bind the nucleotide GTP. Activation of the receptor by the neurotransmitter stimulates the binding of GTP, and renders dissociation of the G protein into subunits. These free subunits, in turn, affect the activities of adenylate cyclases, phospholipase C, Phospholipase A2, various kinases and indirectly ion channels. G-protein coupled receptors are found throughout the nervous system. For example, the Autonomic nervous system (ANS) has adrenergic G-protein coupled receptors (sympathetic) or muscarinic G-protein coupled receptors (parasympathetic). These same receptors are also found in the CNS. (more later in the course on the ANS) Kinase Receptors as Transducers of Receptor Activation Receptors that undergo auto phosphorylation leading to a cascade of phosphorylation of intracellular proteins are referred to as kinase receptors (Fig. 16). An example of such a receptor is the insulin receptor. Insulin binds to the receptor, which then forms a dimer with another insulin receptor resulting in the phosphorylation of each other (called auto- phosphorylation) that eventually leads to the Figure 16. Kinase receptors may go through a phosphorylation of other proteins ultimately process of auto-phosphorylation and resulting in the uptake and storage of glucose. phosphorylation of intracellular proteins once a neurotransmitter binds. (Pharmacology Rang et al., 5th ed. 2003) [Block: Nervous System | VANDERAH] [15 of 23] SYNAPTIC TRANSMISSION & NEUROTRANSMITTERS Nuclear Receptors as Transducers of Receptor Activation Nuclear receptors are receptors that are often found within the cytoplasm and/or may be found within the nucleus (Fig. 17). These types of receptors when bound to a ligand will translocate to the nucleus if not in the nucleus already and will bind to specific sequences on the DNA. The binding of the receptor to DNA results in the modulation of gene transcription. One example of a nuclear receptor is the estrogen receptors, which are known to turn on several genes that play a role in female Figure 17. Nuclear receptors are found in the cytosol or even the nucleus and when bound maturation. to an agonist (most often a steroid) they can Versatility of Synaptic Actions of Second bind to DNA and alter DNA transcription and Messenger Linked Receptors translation. The activation of receptors linked to second messenger formation involves many enzymatic reactions. Because of this, the time course for synaptic effects is slower than that of neurotransmitter-gated ion channel receptors. Generally, synaptic actions of 2nd messengers occur over the span of hundreds of milliseconds to hours. Some aspects of “synaptic plasticity” may result from the long-term effects of the biochemical changes induced by 2nd-messenger linked receptors. Alterations in the regulation of the activity of 2nd messenger linked receptors have been postulated to be involved in the pathophysiology of certain neuropsychiatric disease states. Second messenger-linked receptors have been found on presynaptic neurons and auto-regulate the release of neurotransmitter. These types of receptors have been termed auto-receptors. PHARMACOLOGY Local Anesthetics Local anesthetics are used to block acute pain in a small region typically to perform a procedure such as a biopsy, minor surgery or dental work. Local anesthetics act in the direct area where they are administered by inhibiting voltage gated sodium channels. By blocking voltage gated sodium channels, they block neuronal action potentials, hence inhibiting neuronal conduction of the pain signal. Local anesthetics are reversible and differ slightly based on their duration of action. The most common include lidocaine and bupivicaine. Cocaine is often used for nasal surgery due to its ability to cause vasoconstriction along with its local anesthetic effects. Benzocaine lacks efficacy and is the prototype “over-the-counter” local anesthetic used for tooth- aches and hemorrhoid itching. Drugs: Mechanism of action: Lidocaine Amide Voltage gated sodium channel antagonists Mepivicaine Amide Bupivicaine Amide Tetracaine Ester Benzocaine Ester Procaine (Novocain) Ester Cocaine Ester also can block reuptake of catecholamines [Block: Nervous System | VANDERAH] [16 of 23] SYNAPTIC TRANSMISSION & NEUROTRANSMITTERS Unwanted effects (side effects) include high systemic doses that can result in death due to the blockade of neurons responsible for respiration and cardiovascular problems. They can depress skeletal and smooth muscle contraction. High doses that cross the blood brain barrier (get into the CNS) can produce seizures. Ester analogs produce the metabolite PABA that can result in an allergic reaction (Amides do not do this – hint -the amides all have 2 “i”s in the name). Neuromuscular Blockers Under conditions of general anesthesia there is a need to prevent muscle reflex responses (reflex twitching) during surgery. Such drugs are referred to as muscle relaxants resulting in a flaccid paralysis by blocking the interactions between the peripheral nerves and muscle. Surgical paralysis can be achieved by two methods; 1) by competitive inhibition of acetylcholine at the nicotinic channels (skeletal muscle nicotinic receptor antagonists), and 2) by depolarization blockade with a compound like succinylcholine. Succinylcholine is an agonist at the nicotinic receptor stimulating muscle contraction. Unlike acetylcholine, it is not degraded by acetylcholinesterase and therefore degraded slowly, remaining on the nicotinic receptors and inhibiting endogenous acetylcholine interaction and therefore blocking its ability to cause muscle contraction. Hence, there is a shut-down of the receptor referred to as channel desensitization. So, succinylcholine initially causes muscle contraction (Phase I) and then is rapidly followed by a desensitization of the nicotinic channel and a resulting flaccid paralysis (Phase II). The advantage of this approach is that it is short-acting and readily reversible. Drugs: Mechanism of action: Tubocurarine Nicotinic antagonists (Non-depolarizing block) Atracurium Mivacurium Vecuronuium Pancuronium Rocuronium Succinylcholine (Depolarizing Block) Nicotinic agonist that desensitizes the nicotinic receptors Side effects are similar between the antagonists and the depolarizing agent including ganglionic blockade (you will learn that in the ganglion (cell bodies) of the autonomic nervous system lies nicotinic channels (made of differing subunits) that can be affected by these agents) causing decrease in blood pressure and tachycardia. These agents can also cause release of histamine, respiratory paralysis, malignant hyperthermia and death at high doses. Succinylcholine in a person with the genetic variant of plasma cholinesterase (Pseudocholinesterase deficiency) can result in prolonged muscle relaxation and severe apnea. Spasmolytics (muscle relaxants) An important aspect of anesthesia is the use of agents that block muscle contraction for ease in surgery and in cases of malignant hyperthermia. Malignant hyperthermia is a genetic defect that is uncovered during inhalant anesthesia. Under such conditions there is a large release of [Block: Nervous System | VANDERAH] [17 of 23] SYNAPTIC TRANSMISSION & NEUROTRANSMITTERS calcium from the sarcoplasmic reticulum resulting in continual muscle contraction. This results in a large utilization of oxygen and a lethal elevation in body temperature. This condition needs a drug that directly acts on the muscle to stop calcium release from the sarcoplasmic reticulum. The drug used is dantrolene (Dantrium). Dantrolene blocks the calcium release and prevents the malignant hyperthermia but it should be noted that dantrolene is extremely hepatotoxic (toxic to the liver). Other drugs that are considered spasmolytics used to relax skeletal muscle include diazepam (Valium), carisoprodol (Soma) (potentiates GABA- GABAA channel activity) and baclofen (GABAB agonist). You will learn more about these drugs later when we discuss anxiety and motor control. Finally, the last compound that is considered a spasmolytic compound is botulinum toxin (bo-tox). Although used frequently as an anti- wrinkle compound by inhibiting the release of acetylcholine at the neuromuscular junction resulting in the relaxation of the muscle in the local area of injection, botulinum toxin can be used in muscle spasms including multiple sclerosis, cerebral palsy, dystonias and muscle tension headache. Cyclobenzaprine (Flexeril) is used to relax muscles but the mechanism of action is still debated. It is thought to increase the release of Norepinephrine into the ventral horn of the spinal cord by activating a set of descending neurons of the brainstem called the Locus Coeruleus. Drugs: Mechanism of action: Dantrolene blocks calcium release from the Sarcoplasmic Reticulum Diazepam GABAA channel agonist (potentiates GABA Cl- influx) Baclofen GABAB agonist (GPCR inhibitory coupling) Botulinum Toxin Inhibits release of Acetylcholine at the NMJ Carisoprodol GABAA channel agonist (potentiates GABA Cl- influx) Cyclobenzaprine May activate brain-stem (LC) neurons to increase release of NE into the ventral horn of the spinal cord Each of these compounds has unique side effects that one should be aware of as well as the cause of sedation and drowsiness. For a list of side effects see drug tables. INTRODUCTION to NEUROTRANSMITTERS In order to understand psychopharmacology one must build upon the foundations that make up general brain structures that play a role in higher cortical functions. Later in the course we will cover such higher cortical functions and discuss several psychiatric diseases and their relationships to different areas of the central nervous system as well as specific treatments. Here we will begin by laying the foundation of some neurotransmitters and receptors that play a role in different psychiatric diseases. Neurotransmitters are synthesized by different neurons throughout the CNS and interact with several different receptors that are strategically placed throughout the CNS to perform particular functions. In other words, different sets of neurons synthesize specific neurotransmitters and secrete these neurotransmitters to [Block: Nervous System | VANDERAH] [18 of 23] SYNAPTIC TRANSMISSION & NEUROTRANSMITTERS large areas of the CNS. The neurons receiving the neurotransmitter input will have different types of receptors, hence resulting in different functions. For example, it is thought that the neurotransmitter serotonin has over 20 different types of receptors with varying functions. The entire CNS is bathed by certain neurotransmitters from the brainstem that help maintain several functions including general “background noise”. The brainstem neurotransmitters are synthesized by distinct sets of neurons within the brainstem and include serotonin, norepinephrine, dopamine and acetylcholine (there are many other neurotransmitters in the CNS but we will discuss these as some of the major neurotransmitters that have been correlated with different psychiatric disorders). A large source of serotonin synthesis within the CNS comes from a cluster of neurons within the brainstem called the raphe nuclei. The raphe nuclei are located along the midline and can be found all throughout the brainstem from the medulla to the midbrain (Fig. 1). These serotonergic neurons project via a pathway known as the medial forebrain bundle passing through the hypothalamus to widespread regions over the entire cerebral cortex. Serotonergic projections are also sent to the cerebellum and down the lateral funiculus of the spinal cord where they modulate synapses and maintain CNS neuronal “tone” (Fig. 2). It is thought that they continually secrete neurotransmitter establishing a resting state that varies over different levels of consciousness (i.e., more serotonin secreted when awake versus when asleep). Serotonin when released will act on postsynaptic receptors to produce a Fig 1, Cross sections of the brainstem demonstrating number of effects. These receptors are the location of serotonergic neurons called the raphe nuclei (red dots) throughout the brainstem. Nolte’s The Human Brain, 8th ed. Elsevier. all G-protein coupled receptors (GPCRs) labeled as 5-HT receptors with the exception of the 5-HT3 receptor. The 5-HT3 is a cationic, ligand gated channel. There are currently 7 different 5-HT GPCRs (5HT1-7). Some of these have subtypes. Several of the receptors couple to Galpha-s (increasing cAMP – 5HT4, 5HT6 and 5HT7) with 5HT1 and 5HT5 coupling to Galpha-i and 5HT2 coupling to Galpha-q. Fig 2, Hemisected brain illustrating the location of the serotonergic neurons in the Serotonin actions are terminated by re-uptake raphe nuclei and their projections over the through a transporter, as well as by metabolism by cortex, cerebellum and down the spinal cord. Nolte’s The Human Brain, 8th ed., Elsevier. [Block: Nervous System | VANDERAH] [19 of 23] SYNAPTIC TRANSMISSION & NEUROTRANSMITTERS monoamine oxidase (MAO). Most importantly, it is thought that the levels of serotonin are decreased in patients with depression. (Fig. 3). When patients are put on medication for their severe depression there is a significant increase in serotonin levels, as well as a reversal of their severe depression. Examples of medications that are used for depression and increase serotonin levels include drugs that block the reuptake of serotonin from the synaptic cleft (e.g., selective serotonin reuptake inhibitors SSRIs). Examples of SSRIs include Sertraline (Zoloft) Fluoxetine (Prozac) and Paroxetine (Paxil) (see drug tables, antidepressants). Likewise, other Fig. 3, Human study using fNMR psychiatric disorders that have been linked to demonstrating areas of the brain that are decreased levels of serotonin including obsessive thought to have a decrease in serotonin in compulsive disorder (OCD), Tourette’s, panic chronically depressed individuals. Harting et al., attacks and eating disorders. For example, Fig. 4 2007. displays a study performed in monozygotic twins demonstrating that women with a higher body mass index (BMI) had higher levels of the serotonin reuptake transporters suggesting a reduction in the amount of serotonin within the synapses as compared to their leaner co-twins. Finally, the serotonin receptor, 5-HT2, along with dopamine receptors have been targeted, using blockers (antagonists), for the condition of schizophrenia (see below under dopamine). Similar to serotonin, norepinephrine (NE) Fig 4, The upper row shows summed (also called noradrenaline) is made in neurons images of the female twins with higher throughout most parts of the brainstem. BMI and the lower image shows summed Norepinephrine is synthesized within discrete images of their leaner co-twins. The neurons in the rostral pons called the locus serotonin transporter was slightly higher ceruleus, as well as in neurons of the medullary in the hypothalamus/thalamus in the reticular formation women with higher BMI as compared to their leaner co-twins. Koskela et al., Physiol & Beh. 93, 2008. (RF), the dorsal motor nucleus (DMNX) and the nucleus of the solitary tract (Fig. 5). The neurons that produce NE are located more laterally, away from the midline, as compared to the serotonergic neurons. Many of the NE neurons within Fig 5, Cross sections of the brainstem illustrating areas of the reticular formation project down the neurons that synthesis high concentrations of lateral funiculus of the spinal cord whereas norepinephrine including the locus ceruleus and the the noradrenergic neurons of the locus nucleus of the solitary tract. Nolte’s The Human Brain, 8th ed, Elsevier. [Block: Nervous System | VANDERAH] [20 of 23] SYNAPTIC TRANSMISSION & NEUROTRANSMITTERS ceruleus project via the medial forebrain bundle to the cerebral cortex (Fig. 6). Locus ceruleus neurons also send projections into the cerebellum. Norepinephrine acts at 5 different GPCRs. These include the α1, α2, β1, β2, β3. The α1 receptor couple to Galpha-q whereas the α2 receptor couple to Galpha-i. All three of the β receptors couple to Galpha-s. (note, there are subtypes of some of the adrenergic receptors i.e., α1a, α1b, etc. but we will not worry about this) Like serotonin, low levels of NE have been linked to depression. The termination of NE is the same as serotonin; mainly by re-uptake Fig 6, Hemisected brain illustrating location of through a transporter as well as by MAO and neurons that synthesis norepinephrine in the COMT metabolism. locus ceruleus (LC) and their projections over the brain, cerebellum and spinal cord. Nolte’s The Human Brain, 7th ed. 2015, Elsevier. Several medications that raise both serotonin and NE levels (i.e., monoamine oxidase inhibitors (MAOi), tricyclic antidepressants (TCAs) and serotonin and norepinephrine reuptake inhibitors (SNRI) are used in patients with depression. Some examples of each include {TCAs - Imipramine (Tofranil) & Amitriptyline (Elavil)} {SNRIs - Venlafaxine (Effexor) & Duloxetine (Cymbalta)} and {MAOi - Phenelzine (Nardil)}. Another psychiatric disorder that has been linked to decreased NE levels is Attention- Deficit/Hyperactivity Disorder (ADHD) in which drugs like Methylphenidate (Ritalin), Dextroamphetamine-amphetamine (Adderall) and Atomoxetine (Straterra) are used to increase NE levels. Finally, another psychiatric disorder that is associated with INCREASED levels of NE is Post-Traumatic Stress Disorder (PTSD). A common drug used to decrease the effects of NE in PTSD is a beta receptor antagonist (i.e., Propranolol (Inderal) or an alpha2 receptor agonist (i.e., Clonidine (Catapres). An additional neurotransmitter that plays a role in psychopharmacology is dopamine. Unlike serotonin and NE, dopaminergic neurons do not bath the entire brain, but they do project via the medial forebrain bundle to several structures including the temporal cortex, limbic lobe, frontal and prefrontal cortex, as well as to the basal ganglia (Fig. 7). Dopamine acts at a family of GPCRs labeled D1-5. The D1 and D5 are coupled to Galpha-s whereas the D2, D3 and D4 are coupled to Galpha-i receptors. Many of the dopaminergic neurons that play a role in psychiatric disorders project from the ventral tegmental area of the midbrain (Fig. 8). One syndrome of psychiatry that is related to levels of dopamine is schizophrenia. Fig 7, Hemisected brain illustrating location of neurons that synthesis dopamine in the ventral tegmental area (VTA) and their projections over the brain. Nolte’s The Human Brain, 7th ed. 2015, Elsevier. [Block: Nervous System | VANDERAH] [21 of 23] SYNAPTIC TRANSMISSION & NEUROTRANSMITTERS It is thought that there is an imbalance of dopamine with excess secretion into the frontal cortex. The dopamine that travels from the midbrain to the frontal cortex is often referred to as the mesocortical pathway (meso=midbrain). You will also notice other areas of the CNS where dopamine binds, specially the basal ganglia. This dopamine on its way to the basal ganglia that is involved in the initiation of movement is often referred to as the mesostriatal pathway and dopamine on its way towards the reward and limbic parts are often called the mesolimbic Fig 8 Cross section of the midbrain illustrating pathway. areas of nuclei that synthesis high levels of dopamine including the VTA and substantia Drugs used for schizophrenia nigra. Nolte’s The Human Brain, 8th ed. Elsevier. (antipsychotics) block dopamine receptors, hence are dopamine receptor antagonists and include (i.e., Haloperidol (Haldol), and Fluphenazine (Permitil). In addition, there are antipsychotics that have mixed dopamine and serotonin antagonist activity (i.e., Risperidone (Risperdal) and Aripiprazole (Abilify). Although antipsychotic medications are dopamine receptor antagonist they typically interact (have affinity) at several receptors resulting in many side effects. Several other psychiatric disorders involve increased levels of dopamine typically in areas of the limbic parts of the basal ganglia including abuse potential for things like drugs and gambling. For example, it has been shown that individuals Fig 10, Hemisected brain illustrating that abuse drugs demonstrate a large increase in location of neurons that synthesis dopamine in areas of the limbic parts of the basal ganglia. acetylcholine from areas of the reticular Other disorders that are not necessarily psychiatric formation and basal nuclei sending their projections all over the brain. Nolte’s The disorders but are CNS problems include the lack of Human Brain, 8th ed, Elsevier. dopamine (Parkinson’s), or a genetic disorder that is dealt with by inhibiting dopamine (Huntington’s). Finally, we will highlight one other neurotransmitter, acetylcholine, which also “bathes” the CNS and is secreted from two distinct regions of the CNS. Acetylcholine is made in neurons located in the reticular formation of the brainstem as well as in the basal nucleus (Figs. 10 & 11)(NOTE: This is not the basal ganglia). Acetylcholine acts at both nicotinic ion channels and at a family of muscarinic receptors (M). The acetylcholine nicotinic channels are made up of 5 subunits that all come together to make a cationic channel. The nicotinic Fig 21, Coronal section of the brain channels in the skeletal muscle will be quite different illustrating neurons in the basal nucleus compared to the ones in the brain and the autonomic that synthesis acetylcholine and its ganglia. The muscarinic receptors are GPCRs. The M1, distribution over the brain. Nolte’s The M3 and M5 all couple to Galpha-q. The M2 and M4 couple Human Brain, 7th ed. 2015, Elsevier. to Galpha-i. It is thought that acetylcholine levels decrease [Block: Nervous System | VANDERAH] [22 of 23] SYNAPTIC TRANSMISSION & NEUROTRANSMITTERS in diseases related to dementia. The utilization of compounds that maintain levels of acetylcholine (acetylcholinesterase inhibitors) have been shown in some studies to aid in patients with dementia, yet studies are inconclusive. Acetylcholinesterase is an enzyme that lies in the synapse and degrades acetylcholine. Drugs that inhibit acetylcholinesterase and are approved for dementia include Donepezil (Aricept) and Rivastigmine (Excelon). (these are a family of acetylcholinesterase inhibitors that cross the blood brain barrier – unlike the acetylcholinesterases used for myasthenia gravis). Recently studies have demonstrated that acetylcholine levels may play a role in Autism. In addition, acetylcholine levels play an important role in REM sleep (both Autism and sleep will be further discussed later in the course). Keep in mind that these are not the only neurotransmitters in the central nervous system, are not the only neurotransmitters that may be playing a role in the psychiatric disorders, and that these are not the only sets of neurons that make and release the neurotransmitters mentioned above. Overall, it is thought that these four different neurotransmitters play an important role in maintaining a basal level or a neuronal “tone” of the CNS. Changes in these neurotransmitters have been linked to number of different psychiatric disorders that are treated with current medications that modulate such neurotransmitters. [Block: Nervous System | VANDERAH] [23 of 23]