2023 Biochemistry Lecture Notes PDF
Document Details
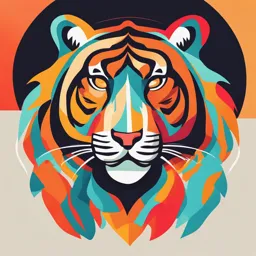
Uploaded by ProfoundBrown
2023
Tags
Related
- Lecture #1 Carbohydrates Metabolism (Glycolysis) PDF
- Biochemistry: Metabolism of Monosaccharides & Disaccharides PDF
- Carbohydrates Biochemistry Lecture PDF
- Carbohydrates PDF - Biochemistry & Molecular Biology
- Lecture 24 Chemistry, Functions and Metabolism of Carbohydrates PDF
- Carbohydrates and Glycogen Metabolism Lecture 3 PDF
Summary
These are lecture notes from a biochemistry course covering metabolism and carbohydrate metabolism. The notes include discussions of catabolism, anabolism, and various metabolic pathways. The notes seem suitable for use by biochemistry students, and the focus is on fundamental concepts.
Full Transcript
1 Lecture 5&6 Introduction to metabolism and Carbohydrates metabolism A First Look at Metabolism 2 Metabolism can be subdivided into two major categories: Catabolism - those processes in which complex substances are degraded to simpler molecules. Ana...
1 Lecture 5&6 Introduction to metabolism and Carbohydrates metabolism A First Look at Metabolism 2 Metabolism can be subdivided into two major categories: Catabolism - those processes in which complex substances are degraded to simpler molecules. Anabolism - those processes concerned primarily with the synthesis of complex organic molecules. Catabolism is generally accompanied by the net release of chemical energy. Anabolism requires a net input of chemical energy. These two sets of reactions are coupled together by ATP. A First Look at Metabolism 3 Both catabolic and anabolic pathways occur in three stages of complexity: Stage 1: the interconversion of polymers and complex lipids with monomeric intermediates Stage 2: the interconversion of monomeric sugars, amino acids, and lipids with still simpler organic compounds Stage 3: the ultimate degradation to, or synthesis from, inorganic compounds, including CO2, H2O and NH3. 4 A First Look at Metabolism A fundamental distinction among organisms lies in the source of their fuel molecules. Autotrophs (from Greek, “self-feeding”) synthesize glucose and all of their other organic compounds from inorganic carbon, supplied as CO2. Heterotrophs (“feeding on others”) can synthesize their organic metabolites only from other organic compounds, which they must therefore consume. A primary difference between plants and animals is that plants are autotrophs and animals are heterotrophs. 5 A First Look at Metabolism Microorganisms show adaptability with respect to their ability to survive in the absence of oxygen. Virtually all multicellular organisms and many bacteria are strictly aerobic organisms; they depend absolutely upon respiration, the coupling of energy generation to the oxidation of nutrients by oxygen. By contrast, many microorganisms either can, or must, grow in anaerobic environments, deriving their metabolic energy from processes that do not involve molecular oxygen. 6 A First Look at Metabolism A brief overview of metabolism: Intermediary metabolism refers primarily to the biosynthesis, utilization, and degradation of low-molecular- weight compound (intermediates). Freeways on the Metabolic Road Map 7 The first pathway that we study is glycolysis, a stage 2 pathway for degradation of carbohydrates, in either aerobic or anaerobic cells. The major input to glycolysis is glucose, usually derived from either energy-storage polysaccharides or dietary carbohydrates. This pathway leads to pyruvate, a three-carbon a-keto acid. Anaerobic organisms reduce pyruvate to a variety of products, for example lactate, or ethanol plus carbon dioxide. These processes are called fermentations. Freeways on the Metabolic Road Map 8 In oxidative metabolism (respiration), the major fate of pyruvate is its oxidation to a metabolically activated two-carbon fragment, acetyl-CoA. The two carbons in the acetyl group then undergo oxidation in the citric acid cycle. In aerobic organisms the citric acid cycle is the principal stage 3 pathway. This cyclic pathway accepts simple carbon compounds, derived not only from carbohydrate but also from lipid or protein, and oxidizes them to CO2. 9 Freeways on the Metabolic Road Map Oxidative reactions of the citric acid cycle generate reduced electron carriers whose reoxidation drives ATP biosynthesis, primarily through processes in the mitochondrial respiratory chain—electron transport and oxidative phosphorylation. The mitochondrial membrane uses oxidative energy to maintain a transmembrane gradient of hydrogen ion concentration (the protonmotive force), and discharge of this electrochemical potential energy powers the synthesis of ATP from ADP + Pi. 10 Freeways on the Metabolic Road Map Overview of metabolism: Shown here are the central metabolic pathways and some key intermediates. Catabolic pathways (red) proceed downward and Anabolic pathways (blue) proceed upward. Note the three stages of metabolism. 11 Freeways on the Metabolic Road Map 12 Freeways on the Metabolic Road Map 13 Freeways on the Metabolic Road Map 14 Freeways on the Metabolic Road Map 15 Freeways on the Metabolic Road Map The initial phase of carbohydrate catabolism: glycolysis: Pyruvate either undergoes reduction in fermentation reactions or enters oxidative metabolism (respiration) via conversion to acetyl-CoA 16 Freeways on the Metabolic Road Map Oxidative metabolism: Oxidative metabolism includes o Pyruvate oxidation o The citric acid cycle o Electron transport o Oxidative phosphorylation Pyruvate oxidation supplies acetyl-CoA to the citric acid cycle. 17 Freeways on the Metabolic Road Map Carbohydrate anabolism: Biosynthesis of carbohydrates includes gluconeogenesis and polysaccharide synthesis. 18 Freeways on the Metabolic Road Map Photosynthesis: 19 Freeways on the Metabolic Road Map Degradative and biosynthetic pathways are distinct for two reasons: A pathway can be exergonic in only one direction Pathways must be separately regulated to avoid futile cycles. Compartmentation and allosteric control of anabolic and catabolic processes prevent futile cycles, which simply waste energy. 20 Freeways on the Metabolic Road Map A similar futile cycle could result from the interconversion of fructose-6-phosphate with fructose- 1,6- bisphosphate in carbohydrate metabolism. 21 Biochemical Reaction Types Much of the chemistry of biological molecules is the chemistry of the carbonyl group because the vast majority of biological molecules contain them. Most of the chemistry of carbonyl groups involves nucleophiles (abbreviated “Nu:”) and electrophiles. Biochemical Reaction Types 22 Energy production in most cells involves the oxidation of fuel molecules such as glucose. Oxidation-reduction, or redox, chemistry thus lies at the core of metabolism. Redox reactions involve reversible electron transfer from a donor (the reductant) to an acceptor (the oxidant). In this example, because the alcohol has lost a pair of electrons and two hydrogen atoms, this type of oxidation is called dehydrogenation, and enzymes that catalyze this reaction are called dehydrogenase. 23 Some Bioenergetic Considerations Most biological energy derives from oxidation of reduced metabolites in a series of reactions, with oxygen as the final electron acceptor. ??? 24 Some Bioenergetic Considerations Not all metabolic energy comes from oxidation by oxygen. Substances other than oxygen can serve as terminal electron acceptors. Many microorganisms either can or must live anaerobically. For example, Desulfovibrio carry out anaerobic respiration using sulfate as the terminal electron acceptor: 25 Some Bioenergetic Considerations The combustion of fat provides more heat energy than the combustion of an equivalent mass of carbohydrate. In other words, fat has a higher caloric content than carbohydrate. Compare the oxidation of glucose with the oxidation of a typical saturated fatty acid, palmitic acid. 26 Some Bioenergetic Considerations Another way to express the degree of substrate oxidation is to say that more reducing equivalents are derived from oxidation of fat than from oxidation of carbohydrate. A reducing equivalent can be defined as 1 mole of hydrogen atoms (one proton and one electron per H atom). For example, two moles of reducing equivalents are used in the reduction of one-half mole of oxygen to water: ½ O2 + 2e- + 2H+ à H2O 27 Some Bioenergetic Considerations The major source of electrons for reductive biosynthesis is NADPH, nicotinamide adenine dinucleotide phosphate (reduced). NADP+ and NADPH are identical to NAD+ and NADH, respectively, except that the former have an additional phosphate esterified at C-2 on the adenylate moiety. Some Bioenergetic Considerations 28 Nicotinamide nucleotides in catabolism and biosynthesis: NAD+ is the cofactor for most enzymes that act in the direction of substrate oxidation (dehydrogenases). NADPH usually functions as a cofactor for reductases, enzymes that catalyze substrate reduction. 29 Some Bioenergetic Considerations The fundamental biological role of ATP as an energy-coupling compound is to convert thermodynamically unfavorable processes into favorable processes. Activated intermediates, such as ATP, allow reactions to occur under physiologically relevant concentrations of metabolic intermediates. Some Bioenergetic Considerations 30 Phosphoanhydride (High-energy phosphate) bonds are thermodynamically unstable, but kinetically stable—large free energies of activation require enzymes to lower the activation barrier. 31 Some Bioenergetic Considerations ATP can drive the synthesis of higher- energy compounds, if nonequilibrium intracellular concentrations make such reactions exergonic. (creatine phosphate) 32 Some Bioenergetic Considerations ATP can also drive the synthesis of compounds of even higher phosphate transfer potential, such as creatine phosphate. This compound shuttles phosphate bond energy from ATP in mitochondria to myofibrils, where that bond energy is transduced to the mechanical energy of muscle contraction. Creatine phosphate (CrP) is produced from creatine by the enzyme creatine kinase: 33 Some Bioenergetic Considerations Enzyme levels in a cell may change in response to changes in metabolic needs. Enzyme activity is regulated by interaction with substrates, products, and allosteric effectors and by covalent modification of enzyme protein. Enzymes catalyzing sequential reactions are often associated, even in the cytosol, where organized structures are difficult to visualize. 34 Major Metabolic Control Mechanisms Locations of major metabolic pathways within a eukaryotic cell: This hypothetical cell combines features of a plant cell and an animal cell. 35 Major Metabolic Control Mechanisms Second messengers transmit information from hormones bound at the cell surface, thereby controlling intracellular metabolic processes. Experimental Analysis of Metabolism 36 By inactivating individual enzymes, mutations and enzyme inhibitors help identify the metabolic roles of enzymes. The steps of a hypothetical metabolic pathway are identified by analysis of mutants defective in individual steps of the pathway. We can identify metabolite C as the substrate for enzyme III by the absence of this enzyme in mutants that accumulate C. We know that D and E follow C in the pathway because feeding either D or E to mutants defective in enzyme III bypasses the genetic block and allows the cells to grow. Radioisotopes and the 37 Liquid Scintillation Counter Radioisotopes revolutionized biochemistry when they became available to investigators shortly after World War II. Radioisotopes extend by orders of magnitude the sensitivity with which chemical species can be detected. Traditional chemical analysis can detect and quantify molecules in the micromole or nanomole range (i.e., 10-6 to 10-9 mole). A compound that is “labeled,” containing one or more atoms of a radioisotope, can be detected in picomole or even femtomole amounts (i.e., 10-12 to 10-15 mole). Radiolabeled compounds are called tracers because they allow an investigator to follow specific chemical or biochemical transformations in the presence of a huge excess of nonradioactive material. Radioisotopes and the 38 Liquid Scintillation Counter Radioisotopes and the 39 Liquid Scintillation Counter Of the many uses of stable isotopes in biochemical research, we mention three applications here. First, incorporation of a stable isotope often increases the density of a material because the rare isotopes usually have higher atomic weights than their more abundant counterparts. o This difference presents a way to separate labeled from nonlabeled compounds physically, as in the Meselson–Stahl experiment on DNA replication. Second, compounds labeled with stable isotopes, particularly are widely used in NMR (nuclear magnetic resonance) studies of molecular structure and dynamics. Third, stable isotopes are used to study reaction mechanisms. The “kinetic isotope effect” refers to the effect on reaction rate of substitution of an atom by a heavy isotope. Radioisotopes and the 40 Liquid Scintillation Counter Meselson–Stahl experiment on DNA replication Metabolomics 41 The development of these new technologies has driven the “- omics” revolution: o Genomics o Transcriptomics o Proteomics o Metabolomics Hundreds or even thousands of specific components are measured simultaneously in a biological sample. Thus, it is now possible to measure the full set of transcripts (transcriptome), proteins (proteome), or metabolites (metabolome) in a particular cell or tissue. The metabolome represents the ultimate molecular phenotype of a cell under a given set of conditions because all the changes in gene expression and enzyme activity eventually lead to changes in cellular metabolite levels (the metabolic state or profile). 42 Carbohydrate Metabolism: Glycolysis, Gluconeogenesis, Glycogen Metabolism, and the Pentose Phosphate Pathway Glycolysis: An Overview 43 Catabolic and anabolic processes in anaerobic carbohydrate metabolism: The gold arrows show the glycolytic pathway and the breakdown of polysaccharides that supply this pathway. Glycolysis generates ATP anaerobically and provides fuel for the aerobic energy-generating pathways. The green arrows show the gluconeogenesis pathway, the synthesis of polysaccharides such as glycogen. The blue arrow shows the pentose phosphate pathway, an alternative carbohydrate oxidation pathway needed for nucleotide synthesis. The numbers 1, 2, and 3 identify the three stages of metabolism 44 Glycolysis: An Overview The two phases of glycolysis and the products of glycolysis: 45 Reactions of Glycolysis The chemical strategy of glycolysis can be condensed into three processes: 1.Add phosphoryl groups to glucose, yielding compounds with low phosphate group–transfer potential priming. 2.Chemically convert these low phosphate group–transfer potential intermediates into compounds with high phosphate group–transfer potential. 3.Chemically couple the energy–yielding hydrolysis of these high phosphate group–transfer potential compounds to the synthesis of ATP by transfer of the phosphate group to ADP. 46 Reactions of Glycolysis ATP is synthesized by three major routes: o Substrate-level phosphorylation o Oxidative phosphorylation o Photophosphorylation Glycolysis produces ATP via substrate-level phosphorylation 47 Reactions of Glycolysis An overview of glycolysis: This condensed view of glycolysis shows the key intermediates and reactions in each of the two major phases. In the energy-generating phase, two ATPs are produced for each ATP utilized in the energy-investment phase. 48 Reactions of Glycolysis The first five reactions constitute the energy- investment phase of glycolysis. 49 Reactions of Glycolysis Reaction 1: The First ATP Investment Glycolysis begins with the ATP-dependent phosphorylation of glucose, catalyzed by hexokinase. Reactions of Glycolysis 50 Mammals possess several molecular forms of hexokinase. Different molecular forms of an enzyme catalyzing the same reaction are called isoenzymes, isozymes, or isoforms. Most tissues express hexokinase I, II, or III, all low KM enzymes. Because intracellular glucose levels (2–15 mM) are usually far higher than the KM value for hexokinase, the enzyme often functions in vivo at saturating substrate concentrations. A low affinity isozyme of hexokinase IV in liver with a sigmoidal dependence on glucose concentration permits that organ to adjust glucose utilization to glucose supply at high blood glucose levels. 51 Reactions of Glycolysis Reaction 2: Isomerization of Glucose-6-phosphate. The next reaction, catalyzed by glucose-6-phosphate isomerase (also called phosphoglucoisomerase), is the reversible isomerization of the aldose, glucose-6-phosphate (G6P), to the corresponding ketose, fructose-6-phosphate (F6P). 52 Reactions of Glycolysis This isomerization reaction proceeds via an enediolate intermediate. B: and B-H represent active-site amino acid residues acting as bases and acids, respectively. Reactions of Glycolysis 53 Reaction 3: The Second Investment of ATP Phosphofructokinase catalyzes a second ATP-dependent phosphorylation, to give a hexose derivative, fructose-1,6-bisphosphate (FBP), phosphorylated at both carbons 1 and 6. Transferring the carbonyl oxygen from carbon 1 to carbon 2 has two important effects: 1. The hydroxyl group generated at carbon 1 can be phosphorylated in the reaction 3. 2. It also sets up the sugar for a symmetric aldol cleavage in reaction 4. The phosphofructokinase reaction is the primary step at which glycolysis is regulated. Reactions of Glycolysis 54 Reaction 4: Cleavage to Two Triose Phosphates Fructose-1,6-bisphosphate aldolase, catalyzes the “splitting of sugar” that is connoted by the term glycolysis. The six-carbon sugar fructose-1,6-bisphosphate is cleaved to give 2 three-carbon intermediates, glyceraldehyde-3-phosphate and dihydroxyacetone phosphate (DHAP). Aldolase cleaves fructose-1,6-bisphosphate under intracellular conditions, even though the equilibrium lies far toward fructose-1,6- bisphosphate under standard conditions. Reactions of Glycolysis 55 Reaction mechanism for fructose-1,6-bisphosphate aldolase: A protonated Schiff base intermediate (iminium ion) occurs between the substrate and an active site lysine residue. An aspartate residue facilitates the reaction via general acid–base catalysis. 56 Reactions of Glycolysis Reaction 5: Isomerization of Dihydroxyacetone Phosphate The function of reaction 5, catalyzed by triose phosphate isomerase, is conversion of one of the products from reaction 4, dihydroxyacetone phosphate (DHAP), to the other, glyceraldehyde-3-phosphate (GAP). 57 Reactions of Glycolysis Reactions 6–10: The Energy Generation Phase 58 Reactions of Glycolysis Reaction 6: Generation of the First Energy-Rich Compound This reaction, catalyzed by glyceraldehyde-3-phosphate dehydrogenase, is among the most important in glycolysis, partly because it generates the first intermediate with high phosphate transfer potential and partly because it generates a pair of reducing equivalents. 59 Reactions of Glycolysis Reaction 7: The First Substrate-Level Phosphorylation Because of its high phosphate transfer potential, 1,3- bisphosphoglycerate has a strong tendency to transfer its acyl- phosphate group to ADP, with resultant formation of ATP. This substrate-level phosphorylation reaction is catalyzed by phosphoglycerate kinase. 60 Reactions of Glycolysis Phosphoglycerate kinase catalyzes the first glycolytic reaction that forms ATP. The glyceraldehyde-3-phosphate dehydrogenase and phosphoglycerate kinase are thermodynamically coupled: 61 Reactions of Glycolysis Reaction 8: Preparing for Synthesis of the Next High- Energy Compound Activation of 3-phosphoglycerate begins with an isomerization catalyzed by phosphoglycerate mutase. The enzyme transfers phosphate from position 3 to position 2 of the substrate to yield 2-phosphoglycerate. Reactions of Glycolysis 62 Reaction 8 is slightly endergonic under standard conditions. However, the intracellular level of 3-phosphoglycerate is high relative to that of 2-phosphoglycerate (2PG), so that in vivo the reaction proceeds to the right without difficulty. The enzyme contains a phosphohistidine residue in the active site. In the first step of the reaction, the phosphate is transferred from the enzyme to the substrate to give an intermediate, 2,3 bisphosphoglycerate. Transfer of the phosphate from C-3 to the active site of the enzyme regenerates the phosphorylated enzyme and forms the product, which is released. 63 Reactions of Glycolysis Reaction 9: Synthesis of the Second High-Energy Compound Reaction 9, catalyzed by enolase, generates phosphoenolpyruvate (PEP), another compound with very high phosphate transfer potential. PEP participates in the second substrate-level phosphorylation of glycolysis. Reactions of Glycolysis 64 Reaction 10: The Second Substrate-Level Phosphorylation In the last reaction, catalyzed by pyruvate kinase, phosphoenolpyruvate transfers its phosphoryl group to ADP in another substrate-level phosphorylation. Note that the enzyme is named as if it were acting leftward in the reaction shown below, even though it is strongly exergonic in the rightward direction. Many enzymes were named before the function or direction of intracellular catalysis had been identified. 65 Reactions of Glycolysis The reaction mechanism of pyruvate kinase: Reactions of Glycolysis 66 Pyruvate kinase (PK) is another site for metabolic regulation. PK, like many enzymes, exists in animal tissues as multiple isozymes. Mammals carry two paralogous pyruvate kinase genes, which, through alternative splicing, produce four different PK isozymes. All four PK isozymes are homotetramers of 60 kDa subunits, and except for the M1 isozyme, require allosteric activation by fructose-1,6-bisphosphate for full activity. The L and R isozymes are allosterically inhibited at high ATP concentrations. The M1 isozyme, on the other hand, is always active. Dietary carbohydrate induces the biosynthesis of pyruvate kinase and increases the ability of the body to obtain energy from glycolysis. Reactions of Glycolysis 67 Reactions of Glycolysis 68 Net: Glucose + 2ADP +2Pi + 2NAD+ -> 2 pyruvate +2ATP +2NADH + 2H+ +2H20 ΔGo- -83.1 ΔG -102.9 69 Metabolic Fates of Pyruvate Pyruvate represents a central metabolic branch point. Its fate depends crucially on the oxidation state of the cell, which is related to the reaction catalyzed by glyceraldehyde- 3-phosphate dehydrogenase (reaction 6). Recall that this reaction converts 1 mole of NAD+ per mole of triose phosphate to NADH. The cytoplasm has a finite supply of NAD+ so this NADH must be reoxidized to for glycolysis to continue. 70 Glycolysis: An Overview A fermentation is an energy-yielding metabolic pathway with no net change in the oxidation state of products compared to substrates. Anaerobic glycolysis (like aerobic glycolysis) leads to pyruvate, but the pyruvate is then reduced, so no net oxidation of glucose occurs. 71 Glycolysis: An Overview Alcoholic fermentation involves cleavage of pyruvate to acetaldehyde and CO2 with the acetaldehyde then reduced to ethanol by alcohol dehydrogenase: 72 Glycolysis: An Overview Fermentations use a common strategy to regenerate oxidized NAD+. 73 Metabolic Fates of Pyruvate Pyruvate must be reduced to lactate when tissues are insufficiently aerobic to oxidize all of the NADH formed in glycolysis. Microorganisms can oxidize NADH via fermentations producing lactate or ethanol. 74 Metabolic Fates of Pyruvate Yeast converts pyruvate to ethanol in a two-step pathway: This alcoholic fermentation starts with the nonoxidative decarboxylation of pyruvate to acetaldehyde, catalyzed by pyruvate decarboxylase. NAD+ is regenerated in the next reaction, the NADH-dependent reduction of acetaldehyde to ethanol, catalyzed by alcohol dehydrogenase. Metabolic Fates of Pyruvate 75 The first reaction requires thiamine pyrophosphate as a coenzyme. The vitamin is structurally complex, but its conversion to the coenzyme form, thiamine pyrophosphate, or TPP, involves simply an ATP-dependent pyrophosphorylation. Thiamine pyrophosphate is the coenzyme for al decarboxylations of a-keto acids. Unlike b-keto acids, a-keto acids cannot stabilize the carbanion transition state that develops during decarboxylation, and they thus require the aid of a cofactor (TPP). The thiazole ring of TPP is the functional part of the coenzyme, allowing it to bind and transfer activated aldehydes. Metabolic Fates of Pyruvate 76 Because the thiazole ring is weakly acidic (pKa ~ 18 for the ring hydrogen), a general base is required in its ionization. As shown in the following diagram, a glutamate carboxyl group in the enzyme deprotonates N-1 of the pyrimidine, which in turn increases the basicity of the amino group, facilitating the deprotonation of C-2 of the thiazole ring to give a dipolar thiazolium carbanion (or ylid). Metabolic Fates of Pyruvate 77 Thiamine pyrophosphate in the pyruvate decarboxylase reaction: The key reaction (step 2) is attack by the carbanion of TPP on the carbonyl carbon of pyruvate and is followed by nonoxidative decarboxylation of the coenzyme-bound pyruvate to give another carbanion (step 3), which is resonance-stabilized. 78 Energy and Electron Balance Sheets Glycolysis, which yields 2 ATP per glucose, is fast but releases only a small fraction of the energy available from glucose. In alcoholic fermentation as in lactate fermentation, an exergonic process coupled to an endergonic process. 79 Energy and Electron Balance Sheets Energy profile of anaerobic glycolysis: Most of the reactions function at or near equilibrium and are freely reversible in vivo. The three enzymes that catalyze reactions so highly exergonic as to be virtually irreversible(arrows) are subject to allosteric control. 80 Gluconeogenesis Synthesis and use of glucose in the human body: Liver and kidney cortex are the primary gluconeogenic tissues. Brain, skeletal muscle, kidney medulla, erythrocytes, and testes use glucose as their sole or primary energy source, but they lack the enzymatic machinery to synthesize it. Synthesis of glucose from noncarbohydrate precursors is essential for maintenance of blood glucose levels within acceptable limits. 81 Gluconeogenesis Gluconeogenesis uses specific enzymes to bypass three irreversible reactions of glycolysis. Reactions of glycolysis and gluconeogenesis: Irreversible reactions of glycolysis are shown in dark purple. The opposed reactions in gluconeogenesis, which bypass these steps, are shown in dark blue. Pale arrows identify reversible reactions used in both pathways. 82 Gluconeogenesis Bypass 1: Conversion of Pyruvate to Phosphoenolpyruvate The bypass of pyruvate kinase begins in the mitochondrion and involves two reactions. Pyruvate carboxylase catalyzes the ATP- and biotin-dependent conversion of pyruvate to oxaloacetate. The enzyme requires acetyl-CoA as an allosteric activator: Gluconeogenesis 83 To be used for gluconeogenesis, oxaloacetate must move out of the mitochondrion to the cytosol, where the remainder of the pathway occurs. However, the mitochondrial membrane does not have an effective transporter for oxaloacetate. Therefore, oxaloacetate is reduced by mitochondrial malate dehydrogenase to malate, which is transported into the cytosol by exchange for orthophosphate and then reoxidized by cytosolic malate dehydrogenase. Once in the cytosol, oxaloacetate is acted on by phosphoenolpyruvate carboxykinase (PEPCK) to give phosphoenolpyruvate: Gluconeogenesis 84 Bypass 2: Conversion of Fructose-1,6-bisphosphate to Fructose-6-phosphate o The PFK reaction of glycolysis is essentially irreversible, but only because it is driven by phosphate transfer from ATP. o A bypass reaction in gluconeogenesis involves a simple hydrolytic reaction, catalyzed by fructose-1,6- bisphosphatase. Bypass 3: Conversion of Glucose-6-phosphate to Glucose o Glucose-6-phosphate cannot be converted to glucose by reverse action of hexokinase or glucokinase because of the high positive DGo of that reaction; phosphate transfer from ATP makes that reaction virtually irreversible. o Another enzyme specific to gluconeogenesis, glucose-6- phosphatase, also involves a simple hydrolysis. 85 Gluconeogenesis PEPCK isozymes provide alternative routes to PEP and cytoplasmic reducing equivalents: 86 Gluconeogenesis 87 Gluconeogenesis Outline of pathways for glucose synthesis from the major gluconeogenic precursors: Note that both glucose and lactate are carried in the blood. The equivalent of 11 high-energy phosphates are consumed per mole of glucose synthesized by gluconeogenesis. The most important gluconeogenic precursors are lactate, alanine, glycerol, and propionate. Animals cannot undergo net conversion of fat to carbohydrate. Gluconeogenesis 88 The Cori cycle: Lactate produced in glycolysis during muscle exertion is transported to the liver, for resynthesis of glucose by gluconeogenesis. Transport of glucose back to muscle for synthesis of glycogen, and its reutilization in glycolysis, completes the cycle. Gluconeogenesis 89 In all organisms a three-carbon acyl-CoA, propionyl-CoA, is generated either from the breakdown of some amino acids or from the oxidation of fatty acids with odd numbers of carbon atoms. Propionyl-CoA enters gluconeogenesis via its conversion to succinyl-CoA and then to oxaloacetate. The process involves a coenzyme derived from vitamin B12. Coordinated Regulation of 90 Glycolysis and Gluconeogenesis Louis Pasteur observed that when anaerobic yeast cultures metabolizing glucose were exposed to air, the rate of glucose utilization decreased dramatically. It became clear that this phenomenon, known as the Pasteur effect, involves the inhibition of glycolysis by oxygen. This effect makes biological sense because far more energy is derived from complete oxidation of glucose than from glycolysis alone. Coordinated Regulation of 91 Glycolysis and Gluconeogenesis Alternative fates of glycolytic intermediates in biosynthetic pathways: Coordinated Regulation of 92 Glycolysis and Gluconeogenesis Comparative substrate cycles in glycolysis/gluconeogenesis: Coordinated Regulation of 93 Glycolysis and Gluconeogenesis Major control mechanisms affecting glycolysis and gluconeogenesis: Note the strongly exergonic reactions of glycolysis and gluconeogenesis and the major activators and inhibitors of these reactions. Conditions that promote glycolysis inhibit gluconeogenesis, and vice versa. Coordinated Regulation of 94 Glycolysis and Gluconeogenesis Fructose-2,6-bisphosphate is the most important regulator of glycolysis and gluconeogenesis. It is synthesized and degraded by different forms of the same enzyme. Coordinated Regulation of 95 Glycolysis and Gluconeogenesis Regulation of the synthesis and degradation of fructose- 2,6-bisphosphate in liver: The bifunctional PFK-2/FBPase-2 is controlled by reversible phosphorylation of a specific serine residue near the N- terminus of each subunit of the homodimeric protein. In the unphosphorylated form, the 6-phosphofructo-2-kinase domain (K) is active, and fructose-2,6-bisphosphate (F2,6BP) is synthesized. In the phosphorylated form, the fructose-2,6- bisphosphatase domain (B) is active, and F2,6BP is degraded. Glucagon stimulates phosphorylation by activating cAMP- dependent protein kinase (PKA). Insulin and glucose (via xyulose-5-phosphate) stimulate dephosphorylation by activating a protein phosphatase. Coordinated Regulation of 96 Glycolysis and Gluconeogenesis Regulation of liver hexokinase by protein–protein interactions. Activity of the liver hexokinase isozyme IV (HKIV) is regulated by alternative protein–protein interactions with the glucokinase regulatory protein (GKRP) in the nucleus and dephosphorylated PFK- 2/FBPase-2 in the cytoplasm. Insulin binding to its plasma membrane receptor stimulates dephosphorylation of PFK-2/FBPase-2. Glut2, plasma membrane glucose transporter. Entry of Other Sugars into the 97 Glycolytic Pathway Routes for utilizing substrates other than glucose in glycolysis: In animals, most of the carbohydrate other than glucose and glycogen comes from the diet, and most of the glycerol is derived from lipid catabolism. Entry of Other Sugars into the 98 Glycolytic Pathway Leloir pathway for utilizing galactose by converting it to glucose-6-phosphate: The galactose and glucose rings are colored blue and orange, respectively, to emphasize that reaction 3 is a group transfer, not an epimerization. Recall that glucose and galactose are epimers at C-4. Entry of Other Sugars into the 99 Glycolytic Pathway The three disaccharides most abundant in foods are maltose, lactose, and sucrose: Maltose is available primarily as an artificial sweetener, derived from starch, while lactose and sucrose are abundant natural products. In animal metabolism they are hydrolyzed in cells lining the small intestine, to give the constituent hexose sugars: Entry of Other Sugars into the 100 Glycolytic Pathway The digestion of neutral fat (triacylglycerols) and most phospholipids generates glycerol as one product. In animals, glycerol first enters the glycolytic pathway by the action in liver of glycerol kinase. The product is then oxidized by glycerol-3-phosphate dehydrogenase to yield dihydroxyacetone phosphate, which is catabolized by glycolysis. 101 Polysaccharide Metabolism In animal metabolism, two primary sources of glucose are derived from polysaccharides: 1. Digestion of dietary polysaccharides, chiefly starch from plant foodstuffs and glycogen from meat. 1. Mobilization of the animal’s own glycogen reserves. 102 Glycogen Metabolism in Muscle and Liver The principal glycogen stores in vertebrates are in skeletal muscle and liver. Breakdown of these stores into usable energy, or mobilization of glycogen, involves sequential phosphorolytic cleavages of bonds, catalyzed by glycogen phosphorylase. In plants, starch is similarly mobilized by the action of starch phosphorylase. Both reactions release glucose-1-phosphate from nonreducing ends of the glucose polymer: 103 Polysaccharide Metabolism Cleavage of a glycosidic bond by hydrolysis or Phosphorolysis: This formal diagram shows how the elements of water or phosphoric acid, respectively, are added across a glycosidic bond. 104 Polysaccharide Metabolism Dietary polysaccharides are metabolized by hydrolysis to monosaccharides. Intracellular carbohydrate stores, as glycogen, are mobilized as phosphorylated monosaccharides by phosphorolysis. a-Amylase in saliva cleaves bonds a(1à4) between the maltose units of amylopectin (or glycogen). However, it cannot cleave a(1à6) glycosidic bonds in the branched polymer, and a limit dextrin accumulates unless a(1à6)-glucosidase (debranching enzyme) is present. Glycogen Metabolism in Muscle and Liver 105 The debranching process in glycogen catabolism: (a)A glycogen chain following activity by phosphorylase, which cleaves off glucose residues to within four residues of the branch point. (a)The glycogen chain following transferase activity by the debranching enzyme. The three remaining glucose residues with linkage have been transferred to a nearby nonreducing end. (a)The glycogen chain following a(1à6)-glucosidase activity by the debranching enzyme, which has removed the last remaining glucose residue of the branch. Phosphorylase will cleave off all but four glucose units of the newly elongated branch, beginning the debranching process again. Glycogen Metabolism in Muscle and Liver 106 UDP-glucose is the metabolically activated form of glucose for glycogen synthesis. Glycogen biosynthesis requires glycogen synthase for polymerization and a transglycosylase to create branches. 107 Glycogen Metabolism in Muscle and Liver The glycogen synthase reaction: 108 Coordinated Regulation of Glycogen Metabolism The branching process in glycogen synthesis. Branching is brought about by the action of amylo-(1,4à1,6)- transglycosylase. Coordinated Regulation of Glycogen Metabolism 109 The regulatory cascade controlling glycogen breakdown: 110 Coordinated Regulation of Glycogen Metabolism Glycogen mobilization is controlled hormonally by a metabolic cascade that is activated by cAMP formation and involves successive phosphorylations of enzyme proteins. The rapid mobilization of muscle glycogen triggered by epinephrine is one of several components of the “fight or flight” response. 111 Coordinated Regulation of Glycogen Metabolism Control of glycogen phosphorylase activity: 112 Coordinated Regulation of Glycogen Metabolism Conditions that activate glycogen breakdown inhibit glycogen synthesis, and vice versa. Glycogen synthase activity is controlled by phosphorylation, through mechanisms comparable to those controlling glycogen breakdown by phosphorylase but having reciprocal effects on enzyme activity. 113 Coordinated Regulation of Glycogen Metabolism Control of glycogen synthase activity: 114 Coordinated Regulation of Glycogen Metabolism Regulation of phosphoprotein phosphatase 1 (PP1) in muscle: 115 Coordinated Regulation of Glycogen Metabolism Regulation of phosphoprotein phosphatase 1 (PP1) in liver: 116 Coordinated Regulation of Glycogen Metabolism A Biosynthetic Pathway That Oxidizes Glucose: 117 The Pentose Phosphate Pathway The pentose phosphate pathway converts glucose to various other sugars, which can be used for energy. Its most important products, however, are NADPH and ribose-5-phosphate. In stage 1, the oxidative phase, glucose-6- phosphate is oxidized to ribulose-5-phosphate and CO2, with production of NADPH. The remaining stages constitute the nonoxidative phase of the pathway. A Biosynthetic Pathway That Oxidizes Glucose: 118 The Pentose Phosphate Pathway Oxidative phase of the pentose phosphate pathway: The three reactions of the oxidative phase include two oxidations, which produce NADPH. The pentose phosphate pathway primarily generates NADPH for reductive biosynthesis and ribose-5-phosphate for nucleotide biosynthesis. A Biosynthetic Pathway That Oxidizes Glucose: 119 The Pentose Phosphate Pathway The reaction sequence of the nonoxidative branch converts 3 five-carbon sugar phosphates to 2 six-carbon sugar phosphates and 1 three-carbon sugar phosphate. The hexose phosphates formed can be catabolized either by recycling through the pentose phosphate pathway or by glycolysis. The triose phosphate is glyceraldehyde-3-phosphate, a glycolytic intermediate. Three enzymes involved are: o phosphopentose epimerase o transketolase o transaldolase A Biosynthetic Pathway That Oxidizes Glucose: 120 The Pentose Phosphate Pathway The pentose phosphate pathway has different modes of operation to meet varying metabolic needs. a) When the primary need is for nucleotide biosynthesis, the primary product is ribose-5- phosphate. Reducing equivalents from NADPH are used to reduce ribonucleotides to deoxyribonucleotide. a) When the primary need is for reducing power (NADPH), fructose phosphates are reconverted to glucose-6-phosphate for reoxidation in the oxidative phase. a) When only moderate quantities of pentose phosphates and NADPH are needed, the pathway can also be used to supply energy, with the reaction products being oxidized through glycolysis and the citric acid cycle.