ZCE 537/4 Ultrasound And Magnetic Resonance Imaging PDF
Document Details
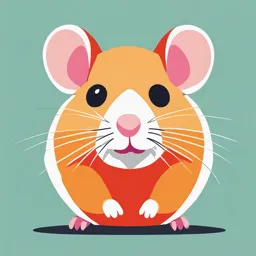
Uploaded by SmarterLagrange
Universiti Sains Malaysia
Nursakinah Suardi
Tags
Summary
This document is a lecture presentation on ultrasonic field interactions, pulse echo ultrasound, and related parameters. It covers various aspects of medical imaging using these concepts.
Full Transcript
ZCE 537/4 ULTRASOUND AND MAGNETIC RESONANCE IMAGING ULTRASONIC FIELD AND ITS INTERACTIONS NURSAKINAH SUARDI [email protected] PULSE ECHO ULTRASOUND 2 PULSED AND CONTINUOUS FIELDS For diagnostic ultrasound imaging, Continuous wave (CW)...
ZCE 537/4 ULTRASOUND AND MAGNETIC RESONANCE IMAGING ULTRASONIC FIELD AND ITS INTERACTIONS NURSAKINAH SUARDI [email protected] PULSE ECHO ULTRASOUND 2 PULSED AND CONTINUOUS FIELDS For diagnostic ultrasound imaging, Continuous wave (CW) not commonly used but pulsed ultrasound are used. The term pulse refers to ultrasound energy that starts then stops again shortly afterwards. 3 PULSED ECHO PRINCIPAL A short ultrasound pulse is delivered to the tissues, and where there are changes in the acoustic properties of the tissue, a fraction of the pulse is reflected (an echo) an returns to the source (pulse-echo principal) Collection of the echoes and analysis of their amplitudes provides information about the tissues along the path of travel. 4 PULSED ECHO PRINCIPAL The ultrasound pulse will travel at the speed of sound and the time between the pulse emission and echo return will be known. Therefore, the depth, d, at which the echo was generated can be determined and spatially encoded in the depth direction. 5 PULSED ECHO PRINCIPAL The total round path distance travelled by the ultrasound (from the probe to the reflector, then from the reflector back to the probe) is (2 × d). The time taken to travel this distance, and therefore the time between the transmit pulse and the echo: The depth of each reflector or scatterer from the echo arrival time (t): 6 PULSED ECHO PRINCIPAL The machine must know the propagation speed c to use this relationship. Since it has no way to determine the actual value for the tissues under examination, it assumes the average value in soft tissue (that is 1540m/sec). With a speed of sound of 1,540 m/s, the time delay between the transmission pulse and the detection of the echo is directly related to the depth of the interface as: It may be useful to remember that for every centimeter of depth the echo 7 delay is 13 μs. PULSED ECHO PRINCIPAL Repeating this process many times with incremental changes in pulse direction transducer transducer allow a volume to be sampled and a tomographic image to be formed. i.e. A tomographic image is formed from a large number of image lines, where each line in the image is produced by a pulse echo sequence 8 *The machine must not transmit again until all detectable echoes from the previous transmit pulse have been received. PULSED ULTRASOUND PARAMETERS Pulse repetition frequency (PRF) The pulse repetition frequency is the rate at which the transducer emits the pulses. The pulses have to be spaced. This allows enough time between pulses so the beam has enough time to reach the target and return to the transducer before the next pulse is generated. ie: Number of pulses occurring in a second. Unit: Hz (hertz). 𝟏 9 PRF = 𝑷𝑹𝑷 PULSED ULTRASOUND PARAMETERS Pulse repetition frequency is the number of pulses occurring in 1 s. In this figure, 5 pulses (containing 2 cycles each) occur in 1 s; thus the pulse repetition frequency is 5 Hz. [Frederick W.Kremkau, 1980] 10 PULSED ULTRASOUND PARAMETERS Pulse repetition frequency (PRF) - cont The PRF is limited by the maximum depth (P) to be sampled and by the acoustic velocity of the medium (c). Using the relationship between depth and echo arrival time, we can calculate when the last detectable echo will be received. Its arrival time (tP) 11 PULSED ULTRASOUND PARAMETERS Pulse repetition period (PRP) Time from the beginning of one pulse to the beginning of the next. Unit: s 𝟏 PRP = 𝑷𝑹𝑭 12 PULSED ULTRASOUND PARAMETERS Pulse repetition period is the time from the beginning of one pulse to the beginning of the next. In this figure, PRP = 0.2 s ; PRF = 5 Hz. [Frederick W.Kremkau, 1980] 13 PULSED ULTRASOUND PARAMETERS Pulse duration (PD) The time for a pulse to occur. Unit: s. [pd] = T [No. of cycles in the pulse] = [No. of cycles in the pulse] / f Duty factor (df) Fraction of time pulses is on. Unitless. [df] = [PD] / [PRP] 14 PULSED ULTRASOUND PARAMETERS Pulse duration is the time for a pulse to occur. In this figure, PD = 0.1 s. Since 2 cycles occur in a cycle, T = 0.05 s, f= 20 Hz. df = PD/PRF = 0.5. 15 ULTRASOUND INTERACTIONS 16 INTRODUCTION Diagnostic ultrasound gives useful information precisely because it interacts strongly with soft tissue. The major types of interaction are: attenuation; reflection; scattering; refraction. 17 ACOUSTIC IMPEDANCE Z1 Tissue Boundary Z2 18 ACOUSTIC IMPEDANCE Acoustic impedance (Z) is a physical property of tissue which describes how much resistance an ultrasound beam encounters as it passes through a tissue. The acoustic impedance (Z) of a material is defined as Z=ρc where ρ is the density in kg/m3 and c is the speed of sound in m/s. The SI unit for acoustic impedance is kg/(m2s). If the density of a tissue increases, impedance increases. Similarly, but less intuitively, if the velocity of sound increases, then impedance also increases. 19 ACOUSTIC IMPEDANCE Soft tissue adjacent to air-filled lungs represents a large difference in acoustic impedance; thus, ultrasonic energy incident on the lungs from soft tissue is almost entirely reflected. When adjacent tissues have similar acoustic impedances, only minor reflections of the incident energy occur. Acoustic impedance gives rise to differences in transmission and reflection of ultrasound energy. 20 21 REFLECTION The reflection of ultrasound energy at a boundary occur between two tissues occurs because of the differences in the acoustic impedances of the two tissues. When the incident ultrasound wave is perpendicular to the boundary, a fraction of it's energy is reflected (an echo) directly back towards the source. The remaining energy is transmitted into the second tissue and continues in the initial direction. 22 REFLECTION 1) Perpendicular reflection When the incident ultrasound wave is perpendicular to the boundary, a fraction of it's energy is reflected (an echo) directly back towards the source The remaining energy is transmitted into the second tissue and continues in the initial direction. 23 REFLECTION 2) Specular reflector When the wave is not incident perpendicular to the interface, non- normal incidence, the reflected angle is equal to the incident angle. Echoes are directed away from the source of ultrasound and may be undetected. The transmitted wave does not continue in the incident direction. The change in direction is described 24 by Refraction. REFLECTION 3) Non specular reflector Also known as diffuse reflection is the reflection of light or other waves or particles from a surface such that a ray incident on the surface is scattered at many angles rather than at just one angle as in the case of specular reflection. 25 REFLECTION The reflection coefficient describes the fraction of sound intensity incident on an interface that is reflected. 26 TRANSMISSION The fraction of the incident energy that is transmitted across an interface is described by the transmission 27 REFRACTION If the velocity of ultrasound is higher in the second medium, then the beam enters this medium at a more oblique (less steep) angle. This behavior of ultrasound transmitted obliquely across an interface is termed refraction. The relationship between incident and refraction angles is described by Snell’s law: 28 REFRACTION Refraction does not occur when the speed of sound is the same in the two media, or when a sound wave is incident perpendicular to the interface. This "straight-line" propagation is assumed by the ultrasound system during signal processing. When refraction does occur, this can result in image artefacts due to the misplacement of anatomy in the image. 29 SCATTERING A change in the direction of motion of a particle because of a collision with another particle. The word scattering describes the interaction of ultrasound with small structures such as red blood cells and capillaries. It differs from reflection in two important ways scattered energy is distributed in all directions, whereas reflected ultrasound goes in a single direction; the scattered energy is generally much weaker than reflected energy and so the echoes due to scattering are generally displayed in the image as low- to mid-level grey tones. 30 SCATTERING Reflection occurs at large tissue interfaces, such as those between organs, where there is a change in acoustic impedance. These large specular reflectors represent a "smooth" boundary where the size of the boundary is much larger than the wavelength of the incident ultrasound wave Within most tissues and organs there are many small-scale variations in acoustic properties which constitute small-scale reflecting particles that are similar in size or smaller than the wavelength of the ultrasound These small nan-specular reflectors represent a "rough" surface 31 and give rise to acoustic scattering within the insonated tissues SCATTERING Scattering from non-specular reflectors reflects sound in all directions. Scattering is a weak interaction in that the amplitude of the returning echoes are significantly weaker than those from tissue boundaries. Intensities of the returning echoes from nonspecular reflectors within the tissue are not greatly dependent on beam direction, unlike specular reflectors. The scattering pattern is characteristic of the particle size and gives rise to tissue or organ signatures that lead to a specific 32 speckle or textured appearance in the ultrasound image SCATTERING Tissue boundary interactions can also give rise to scatter. Specular reflection assumes a "smooth" interface, where the wavelength of the ultrasound is much greater than the structural variations of the interface. With higher frequency ultrasound waves, the wavelength becomes smaller and the interface no longer appears "smooth”. Returning echoes are diffusely scattered (nonspecular reflection) and only a fraction of the reflected intensity returns to the transducer. Scattering from non-specular reflectors increases with ultrasound 33 frequency, but specular reflection is relatively independent. SCATTERING 34 ATTENUATION Ultrasound attenuation is the loss of acoustic energy with distance traveled Caused mainly by scattering and tissue absorption of the incident beam. Absorbed acoustic energy is converted to heat in the tissue. The attenuation coefficient, μ, expressed in units of dB/cm, is the relative intensity loss per centimeter 35 of travel for a given medium. ATTENUATION As an ultrasound wave propagates through a tissue, the energy of the wave reduces with the distance travelled Attenuation describes the reduction in beam intensity with distance travelled and is primarily caused by scattering and tissue absorption of the incident beam The attenuation coefficient, a, (in units dB/cm) is the relative intensity loss per cm of travel for a given tissue The attenuation coefficient varies widely between different tissues and media The attenuation coefficient for a given tissue varies with ultrasound frequency; Attenuation increases linearly with increasing frequency 36 For "soft tissue", the attenuation coefficient can be approximated as 0.5(dB/cm)/MHz ATTENUATION The amount of attenuation is calculated as the ratio of the initial ultrasound intensity (I1) to the final intensity (I2). 37 ATTENUATION 38 ATTENUATION 39 COPYRIGHT All notes, video, exercises, assignments are Copyright @ [nursakinah & 2023]. Any illegal reproduction of this content will result in immediate legal action. 40