Theory of Flight - Aeronautical Fundamentals 2024 PDF
Document Details
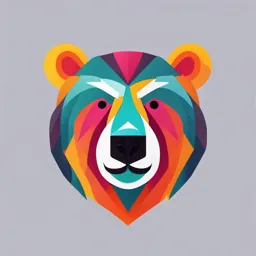
Uploaded by RationalNephrite523
Seneca College
2024
Tags
Summary
This document provides an overview of theory of flight, covering fundamental concepts in aeronautical fundamentals. It discusses various forces acting on an aircraft, including thrust, drag, lift, and weight, and delves into concepts such as airfoil geometry and types.
Full Transcript
Theory of flight AER100 Control Movements Each of the control surfaces, when deflected, changes the geometry of the surface it is attached to. Pitch Control Column, elevator deflection, nose movement Rudder Rudder Pedals, rudder deflection, nose mov...
Theory of flight AER100 Control Movements Each of the control surfaces, when deflected, changes the geometry of the surface it is attached to. Pitch Control Column, elevator deflection, nose movement Rudder Rudder Pedals, rudder deflection, nose movement Ailerons Control Column, aileron deflection, wing roll Trim Alleviates pressure – normally a wheel or push/pull button Forces Acting on an Airplane - Flight Thrust – excreted by engine and propellers pushing air backwards causing thrust in forward direction Drag – resistance to forward motion directly opposed to thrust Lift – upward force to sustain flight Weight – Downward force due to gravity, opposes lift Equilibrium Steady motion – not a state of rest Thrust and drag are equal and opposite Lift and Weight are equal and opposite Lift Generated mainly through the wings. Acts perpendicular to the relative wind and wingspan. Lift is exerted through the centre of pressure. Opposes weight: during level cruise, lift equals weight; Airfoil Any surface designed to obtain a reaction from the air through which it moves – LIFT! Curved/Cambered shape -> produces most lift Upper surface generally has a greater camber than lower Lift The center of pressure of the an airfoil cross section may be different than the center of pressure of the entire wing Likewise the centre of pressure of the wing may be different than that of the entire aircraft Airfoil Terminology Leading Edge = Forward edge of the aerofoil Trailing Edge = Aft edge of the aerofoil Chord = Line connecting the leading and trailing edge. Denotes the length of the aerofoil Mean Camber Line = Line drawn half way between the upper and lower surface of the aerofoil. Denotes the amount of curvature of the wing Point of Maximum Thickness = Thickest part of the wing expressed as a percentage of the chord Airfoil Terminology Angle of Attack=the angle between the chord line of an airfoil and the vector representing the relative motion between the body and the air Angle of Incidence = the angle between the chord line of the wing and the longitudinal axis of the aircraft. It is fixed by the manufacturer, generally between 2 - 4 degrees... Why? The Wing A wing is simply many airfoils connected side by side. Or looking at it the other way around an airfoil is a cross section of a wing The geometry of the airfoil may change from one part of the wing to another. Wing Terminology Wing root – the part attached to the fuselage Wing tip – the part of the wing most distant from the fuselage Wing span-distance from wing tip to wing tip Mean Aerodynamic Chord- this is the average chord length of all the individual slices of aerofoil making up the wing (sounds like calculus) Span x MAC gives wing area Wing Terminology Aspect ratio – The ratio of a wings span to its MAC or Length/Width Sweep back - wing in which the quarter chord line is not parallel with the lateral axis of the aircraft. Wing Terminology Dihedral and Anhedral – the degree to which the wings are canted upwards or downwards from the fuselage Washin and washout – the change in angle of incidence from the root of the wing to the tip of the wing. Washout is much more common Results in the wing root stalling before the wing tip (think of aileron location) How is Lift Created? Newton Bernoulli Coanda NEWTON’S LAWS First Law – Law of Inertia An object in motion tends to stay in straight line motion. Second Law – Law of Acceleration An external force applied will alter the state of uniform motion of a body Third Law – Law of Action / Reaction When a force acts upon an object, the object exerts an equal force in the opposite direction Newton - Lift For every action force there must be a reaction force equal in magnitude but opposite in direction. If no force acts on an object it will continue at a constant velocity By changing the direction of the flow there must be some reaction force This is lift The greater the flow is changed the greater the lift BERNOULLI’S PRINCIPLE Daniel Bernoulli (1700 – 1782) Swiss mathematician His treatise on “Hydrodynamics” of 1738 and a similar one by his father John in 1743, were properly formulated mathematically about 1755 by Leonhard Euler (1707 – 1783), another Swiss mathematician Bernoulli’s equation states: The total energy of any system remains constant Law of Conservation of Energy energy cannot be created or destroyed energy can only change forms although it may appear energy is lost it is NOT i.e. The calorific energy in avgas is dissipated into - mechanical energy, heat from friction, noise, vibration, heat in exhaust, heat in oil and the surrounding air, About 1/8 goes into turning the propeller !!!! Influid flow if the velocity (kinetic energy) increases then the pressure (potential energy) decreases and vice versa FLUID IN MOTION 1. The sum of Potential energy ( Pressure or EP ) and Kinetic energy ( Velocity or Ek ) is constant so total system energy ET = EK + EP 2. Air is a viscous compressible fluid however compressibility effects are negligible below 40% of the speed of sound (about 600 knots). For lower flight speeds at less than 240 knots we consider air is an incompressible perfect fluid. Laminar flow through a venturi CURVE CURVE Volume in = Volume out A2 A1V1 = A2V2 The wider plug at A1 is less deep from front to V2dt back ( V1 dt ) The narrower plug at A2 is deeper from front to back ( V2 dt ) to have the A1 same volume. If the flow is the same, then the plug at A1 must pass in the same time as the plug at A2. V1dt A2 passes V2 dt in the same time as A1 passes V1 dt. As the tube narrows the fluid speed increases proportionally. Coanda Effect Coanda effect is the phenomena in which a jet flow attaches itself to a nearby surface and remains attached even when the surface curves away from the initial jet direction. In free surroundings, a jet of fluid mixes with its surroundings as it flows away from a nozzle. Even if the surface is curved away from the initial direction, the jet tends to remain attached. This effect can be used to change the jet direction. Force on Fluid Coanda Effect For fluid to bend Fluid around the surface there must be a force. The force is in the direction of the bend. From Newton’s 3rd law, there must be an equal and opposite force acting on the object Force on Object Coanda Effect https://www.youtube.com/watch?v=ZDa4OmCxlnM Coanda Effect https://www.youtube.com/watch?v=AvLwqRCbGKY Spoon and Water https://www.youtube.com/watch?v=aF92B6Gon3M Start at 1:35 – Smoke example https://www.youtube.com/watch?v=WPUAq3QObp4 Start at 1:20 How Lift is Created https://www.youtube.com/watch?v=aFO4PBolwFg Typical Airflow Around a Wing RELATIVE WIND Definitions Boundary Layer - a very thin sheet of air lying immediately adjacent to the surface of the wing in which there is local retardation of airflow due to viscosity, progressively more pronounced in closer proximity to the surface, where air flow is often near zero Laminar Layer - airflow over the streamlined shape of an airfoil which occurs in smooth laminations Turbulent Layer - airflow over the wing beginning at the transition point which has developed a waviness to the flow and decays to a random turbulent flow in the process of airflow separation Boundary Layer Airflow at the Surface FREE STREAM BOUNDARY LAYER Acceleration occurs due to low pressure on top of airfoil Coanda effect results in airflow following surface of airfoil This is due to retardation of airflow in boundary layer, streams of air above accelerate into lower pressure area UPPER SURFACE ACCELERATION Lift At low speeds air considered an incompressible fluid Streamlines of air communicate through pressure and viscosity At surface of wing, velocity of air is zero Velocity of streamlines increases away from wing Area known as boundary layer Lift Faster streamlines are bent towards wing by slower streamlines closer to wing surface Theseslower streamlines create area of lower pressure that faster streamlines bend to fill in Enteringarea of lower pressure accelerates streamlines even more Air bends around wing creating downwash and lift LIFT – Center of Pressure GREATLY DECREASED PRESSURE ABOVE CENTER of PRESSURE INCREASED PRESSURE BELOW Four Forces LIFT –ofCentre (Center Pressure)of Pressure Shift With increase in C.P at the C.P. shifts forward stall LOW & HIGH or PRESSURE critical DISTRIBUTION Beyond Stall the C.P. shifts rearward 4o 16o 20o RESULTANT FORCE DECREASED RESULTANT PRESSURE FORCE ABOVE ACTS AT CENTER OF PRESSURE COMPONENTS ARE LIFT AND INDUCED DRAG INCREASED PRESSURE BELOW Angle of Attack at Stall or Critical Angle of Attack vs Resultant Force 0° 3° 8° 12° 16° 20° 0° 3°Angle of Attack 8° 12° 16° Angle of Attack Angle of attack increase - C.P. moves forward Boundary layer separation point moves forward Lift increases to a point (increased pressure differential and downwash) Beyond this point lift decreases (stall) - C.P. moves backwards Stall is independent of aircraft attitude (It is not relative to the horizon) During landing a approaches stall angle Pressure Distribution Relative Airflow Direction of airflow with Flight path and relative Direction and speed of wind respect to the wing airflow are always parallel have no effect on relative but opposite direction airflow Angle of Attack - AoA The relative airflow is not necessarily horizontal Centre of Pressure The distributed pressure in one single force Point where this line cuts the chord As AoA increases up to stall, CofP moves forward Beyond this point, it moves backward Weight Force acting vertically downward towards the centre of the earth Due to gravity Acts through the centre of gravity Resultants of weight act through Thrust Provides forward motion of aircraft Jet, propellers, rockets Pushing air backwards causing a reaction – thrust in forward direction Drag Resistance due to the aircraft moving forward through air To maintain flight, there must be sufficient thrust to overcome drag Desirable to be as low as possible There are Two Types of Drag Induced Parasite Byproduct of lift Created by everything else The more lift you make the more induced This everything else can be divided up drag is created into: Form drag One of the principal goals of wing design Skin friction drag is to make as much lift with as little Interference drag induced drag as possible Some induced drag is inevitable Induced Induced drag is created by those parts of the aeroplane that creates lift—the wings, lifting fuselage sections, and the horizontal tail surface. Induced drag is a by-product of lift. The greater the angle of attack, the greater the lift, and the greater the induced drag. Can only be reduced during initial construction High aspect ratio = less induced drag than low aspect ratio Induced Drag Formula for drag Drag =CD (1/2)ρv2S Where: CD = (CL2) / (pi x AR x e) v – velocity of the airflow ρ- air density S – The area of the wing (span x MAC) AR – aspect ratio E – efficiency factor which is ideally 1 and generally less than 1 Induced Drag Induced drag is associated with difference in pressure that exists above and below a wing surface as airspeed decreases, CL must increase to maintain level flight. This is accomplished by increasing the Angle of attack. As we know the higher the AoA the greater the pressure difference between the upper and lower surface of the wing Induced Drag The tendency for air to flow from high pressure to low pressure creates a flow over the wing tips Flows spanwise outwards Because the wing is moving forward this flow takes the form of a rotating vortex The rotating vortex is an area of low pressure sucking at the trailing edge of the wing Most when: heavy, slow, clean configuration Why? Reducing Induced Drag Induced drag can be reduced by reducing the vortex This is accomplished three main ways Wingtip shape Winglets Droopy tips Reducing Induced Drag Wing Tip Shape Giving the wingtip a higher aspect ratio than the rest of the wing reduces induced drag This is a difficult shape to build though Reducing Induced Drag Winglet Winglets both effectively increase the aspect ratio and partially block the passage of the vortices Less difficult to build than an ellipse Develop enough lift to offset own parasitic drag Droopy Tips Reducing Induced Droopy tips partially block the passage of the vortices Drag Easier to build than winglets Parasite Form drag is caused by the frontal areas of the aeroplane. Skin-friction drag is caused by the air passing over the Drag aeroplane surfaces. Interference drag is caused by the interference of airflow between parts of an aeroplane (wings and fuselage or fuselage and empennage. Reducing Parasite Drag Reducing parasite drag in general: Can never be eliminated completely Eliminate parts Streamlining part Clean aircraft Form Drag The profile of the airframe, landing gear, etc. causes the airflow to separate Depending on the shape of the object, air may either flow smoothly over it or create a turbulent wake This turbulent wake is a series of cyclones, areas of low pressure. The low pressure effectively sucks on the back surface of the object The the narrower the cross section and the smoother the leading and trailing edges, the less form drag there will be Form Drag https://www.youtube.com/watch?v=oEkiDsVGr9c :45 - :52 https://www.youtube.com/watch?v=Q85rb8iKMZk :24 - :56 https://www.youtube.com/watch?v=8F-jS6DcDDY 1:35 – 1:45 Form Drag As evident by the extent of the turbulent airflow behind the three vehicles, a smaller frontal area and a streamlined design will reduce profile drag Which aircraft will have the most profile drag? Form Drag Form drag can also be reduced by eliminating parts of the aircraft which would ordinarily be in the airflow. Examples of these are: landing gear, antennas, radomes. Skin Friction Skin friction is just like it sounds The surfaces of the aircraft are not perfectly smooth The tiny imperfections in the skin resist airflow in the same way that running your hand over sandpaper has more resistance than over glass https://www.youtube.com/watch? v=WTTKFqm20RY 1:00 – 2:00 Skin Friction Skin friction drag can be reduced by Having a good paint job Keeping the aircraft clean and waxed Flush riviting Interference Drag Interference drag is the result of vortexes created where parts of the airplane meet at sharp angles Interference drag can never be completely eliminated It can be reduced by blending components smoothly together This is accomplished either by fairings or by one piece construction methods Interference Drag Can be reduced with fairings and blended body Lift and Drag Curve Unique relationship between lift and drag Also dependent on: Airfoil shape, wing area, true airspeed, air density Lift to Drag Ratio – Cl/Cd Max angle? What does this represent? Mac L/D? What does this represent? C.P movement? Thrust Available & Drag Engine power developed to turn a propeller for thrust Large volume of air Induced backwards When do we have greatest Total amount of thrust from the propeller? As the aircraft moves thrust available decreases, drag Parasite increases 4x thrust required to double speed Streamlining Ailerons control roll They are actuated by rotating the control column Ailerons The control column is connected by way of cables and pulleys to a bell crank which is attached to a push pull rod which moves the aileron Push-pull road connected to bell crank Ailerons Aileron balance is achieved through three design characteristics First is mass balances These work in the same manner as the mass balance on the elevator They also reduce aileron flutter (vibration) Aileron The second design characteristic is call differential (not to be confused with differential ailerons) Simply put, when one aileron goes up the other goes down This improves control effectiveness because to roll you want one wing to generate more lift than the other It also balances the forces acting on the control column Aileron The third design characteristic is called frise Airflow striking the ailerons forward edge projecting below the wing helps balance the force acting on the top surface of the aileron Boundary Layer Thin sheet of air over wing surface Air has viscosity which adheres to the wing Boundary layer flows smoothly over airfoil – laminar layer Near center of wing it becomes turbulent due to skin friction Transition point: boundary layer changes from laminar to turbulent Transition point moves forward: Speed increases AoA increases How do we prevent this? Boundary Layer Thin slots in the Suction method wing, root to tip Siphons through wing Sucks air down the and exausted slot preventing flow backwards as extra from breaking away thrust Boundary Layer Laminar Flow Wing Thickest part of wing is at 50% chord Delays air flow seperation Boundary Layer Vortex Generators Small plates near leading edge Spanwise along wing at small AoA Delays airflow separation by re-energizing it Reduces low AoA performance slightly Assists in preventing stall https://www.youtube.c om/watch?v=099G6XE 8of8 Couples Wing Design Laminar Flow Airfoil Designed to go faster Thinner, more pointed, & nearly symmetrical Thickest at 50% chord – conventional is at 25% Transition point is further aft More even pressure distribution At stall, transition point moves forward more rapidly Wing Tip Design Controlling induced drag and wing tip vortices – increases efficiency Wing tip tanks Increased range Distribute weight over more surface Aids in preventing air from spilling over Wing tip plates Same shape as airfoil but thicker Droop wing tips Winglets Wing Designs Wing Fences Prevent drifting air toward tip Improved slow speed and stall characteristics Slats, Slots & Leading Edge Flaps Help keep flow attached Slat: Retractable Slot: Permanent LEF: mainly on Transport Category Spoilers Increases drag, decreases lift – some linked to ailerons Speed Brakes Small metal blades housed in wing Flaps High lift devices Increase camber and sometimes area (fowler) Increased take-off performance, steeper approaches, lower app/ldg speed Greater flap deflection, greater the drag Steeper path without increased glide sleep C.P moves reward with nose down pitch as flaps are lowered (can be opposite) Slow retraction of flaps due to loss of lift Vfe Wind conditions alter flap selection Full deflection can make the elevator & rudder less effective Axes of an Airplane Axes of Movement Axes of Movement Axes of Movement Roll and Yaw Application of rudder to right Left wing (outside of turn) moves faster than inside Left wing meets relative airflow at higher speed Produces more lift Rudder and Aileron required for a turn! COORDINATION In a roll, aircraft yaws away from turn (remember aileron drag?) Also increased camber on upper wing results in more drag Skids outwards -> required rudder! Yaw Dynamic Yaw Normal movement around the normal axis Static Yaw Condition where aircraft is flying at some angle of sideslip Longitudinal axis not aligned with aircraft flight path Sideslip is when the aircraft is yawed left or right Balanced Controls Some control surface infront of hinge Air strikes forward portion to assist moving the control surface in required direction Sometimes balanced to prevent flutter – mass balance Stability Static Stability Initial tendency to return to the original position when disturbed, in other words a built in resistance to changing attitude Dynamic Stability Overall tendency to return to the original position after attitude has been changed NOTE: An aircraft that is dynamically stable means it must be statically stable, but static stability does not ensure dynamic stability. Static Stability Neutral Negative Positive Dynamic Stability An aircraft may undergo three types of oscillatory motions when disturbed Decaying oscillations – Dynamically Stable Non decaying oscillatory motion – Dynamically Neutral Oscillations of increasing magnitude – Dynamically Unstable Axes of Stability An aircraft may (and often does) have different degrees of stability about each of its three axes For example an aircraft may be statically stable and dynamically neutral longitudinally about the lateral axis; and also be statically and dynamically neutral laterally about the longitudinal axis. This allows designers to tailor the aircrafts flight characteristics to its intended purpose. More maneuverable aircraft are generally less stable Stable aircraft are easy to fly but sluggish Pitch stability is about the lateral axis which runs from wingtip to wingtip Longitudinal Size and position of horizontal stabilizer relative to the Stability center of gravity affect longitudinal stability. The larger the horizontal stabilizer and the farther from the CofG the more stable the aircraft will be. Lateral Stability Roll or lateral stability is about the longitudinal axis which runs from tip of nose to tail It is incorporated into aircraft designs by using: Dihedral Keel Effect Sweepback Lateral Stability Dihedral Normally found on low wing aircraft When a disturbance causes a wing to drop, the lower wing, will have a greater angle of attack and create more lift. Lateral Stability Keel Effect High wing aircraft have most of their weight below the wings. One wing drops, aircraft weight acts like a pendulum, the aircraft returns to the original attitude. Examples of high wing aircraft are the C172 and Dash 8. Note the lack of necessity for dihedral on high wing aircraft. Though some high wings have dihedral as well Anhedral can be built in to give an aircraft the desired maneuverability if other characteristics Lateral Stability make it too stable. Lateral Stability Directional Stability Yaw is controlled about the vertical or normal axis To ensure directional stability, side area of the aircraft must be greater behind the C of G That’s why the vertical stabilizer is at the back Size of the vertical stabilizer and any other vertical surfaces (ventral fins, sea fins etc.) Directional Stability and body length are important aspects in directional stability. Directional Stability Causes of Adverse Yaw 1.Slipstream 2.Asymmetric Thrust 3.Torque 4.Precession 5.Turbulence 6.Aileron Drag 7.Misuse of Rudder As the propeller turns, air moves back in a corkscrew motion under the belly and up the left side Slipstream This causes increased pressure on the left side of the vertical fin and causes a yaw to the left This corkscrew effect of air, shown on the previous slide, increases as thrust increases Tractor vs. Pusher type propeller Asymmetric Thrust (P Factor) At high angles of attack, the descending blade of the propeller has a greater angle of attack than the ascending blade This produces more thrust from the right blade than the left The aircraft will yaw to the left Only significant at high angles of attack and high power settings In cruise flight, both propeller blades meet the relative wind at the same angle and create the same amount of thrust on opposing sides https://www.youtube.com/watch?v=H880hnwtAh0 The left turning tendency of the aircraft due to the Torque propeller rotating clockwise (from the pilot’s perspective) In flight the result is roll but on the ground it creates yaw The manufacturer can compensate for yaw by building a slight right turn tendency into the aircraft Yaw Correction while in cruise flight This is done by offsetting the vertical fin or by offsetting the engine thrust line. Precession Gyroscopic Precession The spinning propeller of the airplane acts like a gyroscope (spinning mass) Spinning masses have a nifty property called precession When a force is applied on one edge of the mass it will react as though the force was applied 90 degrees in the direction of rotation https://www.youtube.com/watch?v=PZU-s8DmqpI Start at :10 When an aircraft changes pitch suddenly from nose up to nose down, the aircraft will yaw to the left Likewise pitching nose up will cause a yaw to the right Turbulence Very difficult to control (unpredictable) Note: Rudder use is usually more effective in controlling roll from turbulence than aileron input Aileron Drag When the aircraft is rolled by the ailerons, the up going wing creates more lift as illustrated earlier. But this means this up going wing creates more drag (see lift/drag relationship from the drag lesson) The aircraft will try to yaw in the direction opposite to the roll’s intended direction Rudder input is needed. The effect is nominal with a slow roll but more pronounced with greater aileron displacement, ie. with a faster roll Frise and differential ailerons also have the benefit of reducing aileron drag induced yaw. The up ailerons leading edge projects below the wing creating drag on the down going wing reducing the adverse yaw. Differential Ailerons The down going wing’s (planned direction of turn) aileron is deflected more than the up going wing’s aileron. This increases form drag counteracting the increased induced drag on the up going side. Frise Ailerons The leading edge of the down going wings aileron sticks out into the airflow creating more from drag. This counteracts the increased induced drag from the up going wings aileron. Misue of Rudder Rudder use must be coordinated with the amount of aileron displacement. On occasion, pilots press too little or too much on the rudder pedals and create yaw. The End Just kidding there is more Climbing Function of elevators to divide energy, produced by the engine in the form of thrust into speed and altitude Effects with no change in thrust Effects with adding thrust Climb until reaching absolute altitude – impossible to climb Best Rate Vy – least time Best Angle Vx – given distance Gliding Thrust is no longer available Angle vs airspeed relationship 20% further glide with stopped propeller vs windmilling Windmilling propeller providing negative thrust -> Drag Best Glide – AoA for max L/D Still air -> any variation reduces distance Headwind -> glide distance reduced (can increase distance if you lower nose) Tailwind -> glide distance increased (can increase distance if you raise nose) Weight has no effect on glide angle assuming correct glide airspeed is maintained Turns Lift vector inclined away from vertical What does this imply? Creates new horizontal force Centripetal force – counteracts centrifugal force Steeper angle of bank Greater rate of turn, less radius of turn, higher stall speed, greater loading Higher the airspeed Slower rate of turn, larger radius Climbing/descending turns are same as level, except adjusting power Turns Lateral stability for climbing/descending turn affected by relative airflow for each wing Descending Inner wing – higher AoA – more lift Outwer wing – travelling faster - also more lift Compensate each other and angle of bank remains constant Climbing Inner wing – smaller AoA Outer wing – higher AoA – greater speed – lots of lift -> results in tendency to increase angle of bank Load Factor Load factor increases in a turn Other maneuvers can rapidly increase load factor https://mediafiles.aero.und.edu/aero.u nd.edu/aviation/trainers/forces-in-a- turn/ Stall Just like when the spoon was moved too far from the water stream, if the angle of attack is increased too far airflow will separate from the upper surface ThAircraft passes critical AoA This is called aerodynamic stall. Sense buffeting Stall Aerodynamic stall is the condition where the wing no longer produces sufficient lift to sustain flight Stall occurs when the smooth flow of air over the wing become disconnected by a too high angle of attack The abruptness and predictability of this varies with wing and aerofoil design https://www.youtube.com/watch?v=6UlsArvbTeo Start at :53 – Smoke example of airfoil Must reduce AoA to recover! Factors Affecting Stall Weight Centre of Gravity Turbulence Turns Flaps Contamination – snow, frost, ice, dirt Heavy Rain Preventing Stall Why are wings not just flat plates? If they are at a positive angle of attack and Coanda effect makes flow change direction and create lift why not use flat wings? The main reason is to keep flow attached High Lift Devices (flaps) Vortex Generators Airfoil Shape Wash-out Preventing Stall Plain Flaps The effect on keeping flow attached is very slight The main effect flaps have is that they increase camber/amount of deflection of flow/lift, at lower angles of attack Slotted Flaps Slotted flaps leave a gap between the wing and flap surface. This helps keep flow attached to the upper surface of the flap Separation if flow is further ahead compared to other flaps for same AoA Slat Slot Slots and Slats are leading edge devices which help keep flow attached. Preventing Stall Slots are permanently mounted Slats are retractable Maneuvers Spin: Autorotation after aggravated stall Increasing AoA reduces lift Ailerons worsen effect Downgoing wing has high AoA, upgoing wing has lower AoA Accelerates rolling moment Spiral Excessive nose-down descending turn Structural damage – airspeed limit and load factor Airspeed Limitations Rate of movement of aircraft relative to air mass Never Exceed Speed/Max Permissible Dive Speed (Vne) Max operate in smooth air Max Structural Cruise Speed/Normal Operating Limit Speed (Vno) Max speed in normal category Maneuvering Speed (Va) Full deflection without damange to structure (stall before failure) Max Flap Extension Speed (Vfe) Max speed with flaps lowered