2 Physical Foundations, Bioenergetics.pdf
Document Details
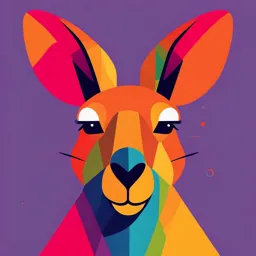
Uploaded by DazzlingFreedom
Tags
Related
- Bioenergetics and Thermodynamics.pdf
- Bioenergetics 1_2024.pdf
- BIOCHEMISTRY TRANS - BIOENERGETICS, MITOCHONDRIAL ELECTRON TRANSPORT, and OXIDATIVE PHOSPHORYLATION.pdf
- BIOCHEMISTRY TRANS - BIOENERGETICS, MITOCHONDRIAL ELECTRON TRANSPORT, and OXIDATIVE PHOSPHORYLATION.pdf
- Harper ENERGETIC.pdf
- Módulo 1. Fundamentos de la Bioquímica y Bioenergética OTOÑO24 parte 2.pptx.pdf
Full Transcript
Physical Foundations of Biochemistry Physical Foundations Physical Foundations Energy from sunlight Photosynthesis O2, glucose H2O, O2 Respiration Biochemical energy conversions obey the laws of thermodynamics. Stored chemical energy and heat Physical Foundations Organisms maintain a c...
Physical Foundations of Biochemistry Physical Foundations Physical Foundations Energy from sunlight Photosynthesis O2, glucose H2O, O2 Respiration Biochemical energy conversions obey the laws of thermodynamics. Stored chemical energy and heat Physical Foundations Organisms maintain a constant composition within them. Physical Foundations The constant composition is brought about by a dynamic steady state, a state far from equilibrium. SYSTEM: ISOLATED, CLOSED, OPEN STATE OF THE SYSTEM EXTENSIVE, INTENSIVE PROPERTIES REVERSIBLE, IRREVERSIBLE HEAT INTERNAL ENERGY ENTHALPY ENTROPY Energy Interconversions in Living Organisms Organisms transform energy and matter from their surroundings. Oxidation-reduction reactions The flow of electrons provides energy for organisms. Entropy, S • “ a change within” • 1851, Rudolf Clausius • randomness and disorder Entropy, S Entropy Cells invest energy to order subunits in their correct sequence within biomolecules. Entropy with font size 10 Free energy must be spent to allow synthesis of macromolecules. Energy changes in processes ∆G = ∆H – T∆S • Gibbs free energy, G Amount of energy capable of doing work during a reaction at constant T and P with font size 10 • Enthalpy, J. Williard Gibbs 1839 – 1903 H Heat content of the system; number and kinds of bonds in the reactants and products • Entropy, S Randomness or disorder in a system Energy changes in processes ∆G = ∆H – T∆S ∆G < 0, spontaneous process Endergonic process Amino Acids Amino Acid Energy changes in processes ∆G = ∆H – T∆S ∆G < 0, spontaneous process Endergonic process Amino Acids Amino Acid Amino acids → protein ∆G1 is positive (endergonic) Energy changes in processes ∆G = ∆H – T∆S ∆G < 0, spontaneous process Endergonic process Amino Acids Amino Acid Amino acids → protein ATP → AMP + P–P [or ATP → ADP + P] ∆G1 is positive (endergonic) ∆G2 is negative (exergonic) Bioenergetics • Energy transformations in living systems • How energy from fuel metabolism or light capture is coupled to the cell’s energyrequiring reactions • ∆G: energy change as the system moves from its initial state to equilibrium • Magnitude of ∆G depends on the particular chemical reaction and how far from equilibrium the system is initially Keq, ∆G°: measures of spontaneity Keq, ∆G°: measures of spontaneity Keq, ∆G°: measures of spontaneity Keq, ∆G°: measures of spontaneity Keq, ∆G°: measures of spontaneity Calculation of ∆G’° Calculate the standard free-energy change of the reaction catalyzed by the enzyme phosphoglucomutase Glucose 1-phosphate ⇄ Glucose 6-phosphate given that, starting with 20 mM glucose 1-phosphate and no glucose 6phosphate, the final equilibrium mixture at 25°C and pH 7.0 contains 1.0 mM glucose 1-phosphate and 19 mM glucose 6-phosphate. Does the reaction in the direction of glucose 6-phosphate formation proceed with a loss or a gain of free energy? Calculation of ∆G’° Calculate the standard free-energy change of the reaction catalyzed by the enzyme phosphoglucomutase Glucose 1-phosphate ⇄ Glucose 6-phosphate given that, starting with 20 mM glucose 1-phosphate and no glucose 6phosphate, the final equilibrium mixture at 25°C and pH 7.0 contains 1.0 mM glucose 1-phosphate and 19 mM glucose 6-phosphate. Does the reaction in the direction of glucose 6-phosphate formation proceed with a loss or a gain of free energy? 𝑔𝑙𝑢𝑐𝑜𝑠𝑒 6 − 𝑝ℎ𝑜𝑠𝑝ℎ𝑎𝑡𝑒 19 𝑚𝑀 # = 𝐾!" = = 19 𝑔𝑙𝑢𝑐𝑜𝑠𝑒 1 − 𝑝ℎ𝑜𝑠𝑝ℎ𝑎𝑡𝑒 1.0 𝑚𝑀 Calculation of ∆G’° Calculate the standard free-energy change of the reaction catalyzed by the enzyme phosphoglucomutase Glucose 1-phosphate ⇄ Glucose 6-phosphate given that, starting with 20 mM glucose 1-phosphate and no glucose 6phosphate, the final equilibrium mixture at 25°C and pH 7.0 contains 1.0 mM glucose 1-phosphate and 19 mM glucose 6-phosphate. Does the reaction in the direction of glucose 6-phosphate formation proceed with a loss or a gain of free energy? 𝑔𝑙𝑢𝑐𝑜𝑠𝑒 6 − 𝑝ℎ𝑜𝑠𝑝ℎ𝑎𝑡𝑒 19 𝑚𝑀 # = 𝐾!" = = 19 𝑔𝑙𝑢𝑐𝑜𝑠𝑒 1 − 𝑝ℎ𝑜𝑠𝑝ℎ𝑎𝑡𝑒 1.0 𝑚𝑀 # Δ𝐺 #$ = −𝑅𝑇𝑙𝑛𝐾!" Δ𝐺 #$ = − 8.314 𝐽/𝑚𝑜𝑙 B 𝐾 298 𝐾 ln 19 Δ𝐺 #$ = −7.3 𝑘𝐽/𝑚𝑜𝑙 Phosphoryl Group Transfers and ATP Phosphoryl Group Transfers and ATP The hydrolysis of ATP is highly exergonic. Phosphoryl Group Transfers and ATP Phosphoryl Group Transfers and ATP Free Energy of Hydrolysis of ATP with Cells: The Real Cost of Doing Metabolic Business In human erythrocytes, the concentrations of ATP, ADP, and Pi are 2.25, 0.25, and 1.65 mM, respectively. Let us assume for simplicity that the pH is 7.0 and the temperature is 25°C, the standard pH and temperature. The actual free energy of hydrolysis of ATP in the erythrocyte under these conditions is given by the relationship Δ𝐺! = Δ𝐺 "# 𝐴𝐷𝑃 𝑃$ + 𝑅𝑇 ln 𝐴𝑇𝑃 Free Energy of Hydrolysis of ATP with Cells: The Real Cost of Doing Metabolic Business In human erythrocytes, the concentrations of ATP, ADP, and Pi are 2.25, 0.25, and 1.65 mM, respectively. Let us assume for simplicity that the pH is 7.0 and the temperature is 25°C, the standard pH and temperature. The actual free energy of hydrolysis of ATP in the erythrocyte under these conditions is given by the relationship Δ𝐺! = Δ𝐺 "# 𝐴𝐷𝑃 𝑃$ + 𝑅𝑇 ln 𝐴𝑇𝑃 Δ𝐺! = Δ𝐺 "# + 𝑅𝑇 ln = −30.5 + 𝑘𝐽 𝑚𝑜𝑙 𝐴𝐷𝑃 𝑃$ 𝐴𝑇𝑃 0.25×10%& 1.65×10%& 8.315 𝐽/𝑚𝑜𝑙 : 𝐾 298𝐾 ln 2.25×10%& Δ𝐺! = −52 𝑘𝐽/𝑚𝑜𝑙 Other Phosphorylated Compounds and Thioethers Other Phosphorylated Compounds and Thioethers Other Phosphorylated Compounds and Thioethers Other Phosphorylated Compounds and Thioethers Other Phosphorylated Compounds and Thioethers Other Phosphorylated Compounds and Thioethers Other Phosphorylated Compounds and Thioethers Other Phosphorylated Compounds and Thioethers Other Phosphorylated Compounds and Thioethers For hydrolysis reactions with large, negative free energy changes, the products are more stable than the reactants for one or more of the following reasons: 1. The bond strain in reactants due to electrostatic repulsion is relieved by charge separation (e.g. ATP); 2. The products are stabilized by ionization (e.g. ATP, acyl phosphates, thioesters); 3. The products are stabilized by isomerization (e.g. PEP); 4. The products are stabilized by resonance (e.g. creatine from phosphocreatine, carboxylate ion from acyl phosphates and thioesters, Pi released from anhydride or ester linkages. Group Transfers Simple hydrolysis in muscle contraction Simple hydrolysis in movement of enzymes along DNA “High-Energy,” “Low-Energy” Compounds Coupling highly exergonic reactions; phosphoryl group transfer potential The ∆G’° values of sequential reactions are additive. Any phosphorylated compound can be synthesized by coupling the synthesis to the breakdown of another phosphorylated compound with a more negative free energy of hydrolysis. Coupling highly exergonic reactions; phosphoryl group transfer potential Transphosphorylations between nucleotides • Nucleoside triphosphates (GTP, UTP, CTP) and deoxynucleoside triphosphates (dATP, dGTP, dTTP, and dCTP) are energetically equivalent to ATP. Transphosphorylations between nucleotides Nucleoside diphosphate kinase (NDPK) Transphosphorylations between nucleotides Nucleoside diphosphate kinase (NDPK): Ping-pong mechanism Transphosphorylations between nucleotides Adenylate kinase: is a phosphotransferase enzyme that catalyzes the interconversion of adenine nucleotides (ATP, ADP, and AMP). By constantly monitoring phosphate nucleotide levels inside the cell, ADK plays an important role in cellular energy homeostasis. Transphosphorylations between nucleotides Creatine Kinase Inorganic polyphosphate is a potential phosphoryl group donor Inorganic polyphosphate is a potential phosphoryl group donor Polyphosphate kinase-1 (PPK-1) in prokaryotes Polyphosphate kinase-2 Inorganic polyphosphate is a potential phosphoryl group donor Helicobacter pylori exposed in anaerobic conditions