Nucleic Acids Metabolism PDF
Document Details
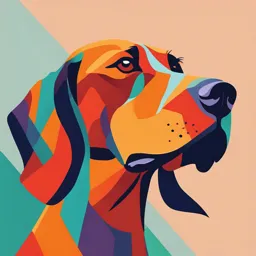
Uploaded by EffortlessRosemary
Tags
Summary
This document details the synthesis and metabolism of nucleic acids, focusing on purines and pyrimidines. It describes the processes involved and the key enzymes involved in both pathways. It also mentions the regulation of the pathways and inhibitors related to them.
Full Transcript
Nucleotide structure of DNA The structures of the nitrogenous bases that make up the nucleotides in DNA, categorized into purines and pyrimidines. Biosynthesis of purine nucleotides Regulation of pur...
Nucleotide structure of DNA The structures of the nitrogenous bases that make up the nucleotides in DNA, categorized into purines and pyrimidines. Biosynthesis of purine nucleotides Regulation of purine nucleotide synthesis 1- De novo synthesis Formation of deoxynucleotides 2- Purine salvage system Lesch-Nyhan syndrome Formation of nucleoside di- and triphosphate Summery of De novo Synthesis: ❖ The de novo pathway of purine synthesis is complex, consisting of 11 steps, and requiring 6 molecules of ATP for every purine synthesized. ❖ The precursors that donate components to produce purine nucleotides include: glycine, ribose 5-phosphate, glutamine, aspartate, carbon dioxide, and N10-formyl FH4 (N¹⁰-formyl tetrahydrofolate). ❖ Purines are synthesized as ribonucleotides, with the initial purine synthesized being inosine monophosphate (IMP). ❖ Adenosine monophosphate (AMP) and guanosine monophosphate (GMP) are each derived from IMP in two-step reaction pathways. 1- De novo synthesis ❖ Little of the dietary purines are utilized for the biosynthesis of nucleotides and nucleic acids. ❖ Most tissues are capable of synthesizing their own purines "de novo". ❖ The origin of the atoms comprising the purine nucleus is shown in the following diagram: CO2 Glycine C 5 7 1 N Aspartate N 6 C 8 C N10-Formyl THF N10-Formyl THF C C 9 2 N 4 N 3 Amide nitrogen of glutamine 1. Ribose 5-phosphate (obtained from the pentose phosphate pathway) is phosphorylated, forming 5-phosphoribosyl-1- pyrophosphate (PRPP), in a reaction catalyzed by the enzyme PRPP synthetase. (PRPP) 2. PRPP is converted to 5- phosphoribosylamine by an amidotransferase enzyme, using the amide nitrogen of glutamine as donor of amino group. ❖ The purine ring is built upon the amino group of the 5-phosphoribosylamine, forming inosine monophosphate (IMP), the parent nucleotide from which AMP and GMP are formed. ❖ This occurs after several steps, requiring glycine, N10-formyltetrahydrofolate (N10-formyl- THF), CO2 (does not require biotin or ATP), aspartic acid and glutamine as precursors. Ribose 5-phosphate PRPP synthetase PRPP Glutamine-PRPP amidotransferase 5’-phosphribosylamine several steps IMP Synthetic inhibitors of purine synthesis 1. Some synthetic inhibitors of purine synthesis (for example, the sulfonamides), are designed to inhibit the growth of rapidly dividing microorganisms without interfering with human cell functions. How? ❖ Sulfonamides are structural analogs of PABA (para-aminobenzoic acid; , a compound that bacteria use to synthesize folic acid) that competitively inhibit bacterial synthesis of folic acid. Because purine synthesis requires THF as a coenzyme, the sulfa drugs slow down this pathway in bacteria. ❖ Humans cannot synthesize folic acid, and must rely on external sources of this vitamin. So, sulfa drugs do not interfere with human purine synthesis. COOH SO2.NH2 Folic acid is synthesized by bacteria from the substrate, para-amino- NH2 NH2 benzoic acid (PABA) PABA Sulfanilamide 2. Other purine synthesis inhibitors, such as structural analog of folic acid (methotrexate), which inhibits human dihydrofolate reductase is used pharmacologically to control the spread of cancer by interfering with the synthesis of nucleotides and, therefore, of DNA and RNA. 3. Trimethoprim is another folate analog, has potent antibacterial activity because of its selective inhibition of bacterial dihydrofolate reductase. The side effects of these anticancer drugs ❖ Inhibitors of human purine synthesis (as methotrexate) are extremely toxic to tissues, especially to developing structures such as in a fetus, or to cell types that normally replicate rapidly, including those of bone marrow, skin, gastrointestinal tract, immune system, or hair follicles. ❖ As a result, individuals taking such anti-cancer drugs can experience adverse effects, Including: anemia, scaly skin, GI tract disturbance, immunodeficiency, and baldness. 2- Purine salvage system ❖ Purine salvage is the conversion of purines and purine nucleosides (resulting from catabolism of purine nucleotides) to the corresponding nucleotides. ❖ This saves purines and purine nucleosides from undergoing further catabolism and excretion. ❖ Thus; 1. Purine salvage decreases de novo synthesis of purine nucleotides and, 2. Decreases the production of uric acid, the end product of purine catabolism. ❖ Tissues incapable of de novo synthesis of purine nucleotides, such as the brain, red blood cell precursors, and the polymorph nuclear leukocytes, receive purines or purine nucleosides from the liver, these are converted by purine salvage systems to the corresponding nucleotides. Two mechanisms are involved in purine salvage: A. Phosphoribosylation of purines, which is more active; and B. Phosphorylation of purine nucleosides, which is less active. ❖ The major enzymes required for purine salvage are purine nucleoside phosphorylase, phosphoribosyl transferases, and deaminases. A. Phosphoribosylation of purines ❖ This involves conversion of purines to the corresponding nucleotides, using PRPP as ribose 5- phosphate donor. Two enzymes catalyze such reactions (1) Hypoxanthine guanine phosphoribosyltransferase (HGPRTase), which is more active; and (2) Adenine phosphoribosyltransferase (APRTase), which is less active. (2) (1) B. Phosphorylation of purine nucleosides ❖ This involves the conversion of adenosine to AMP by the enzyme adenosine kinase, with ATP as phosphate donor. Lesch-Nyhan syndrome ❖ This is an X-linked, recessive, inherited disorder associated with a virtually complete deficiency of HGPRTase and, therefore, the inability to salvage hypoxanthine or guanine. ❖ The enzyme deficiency results in increased levels of PRPP and decreased IMP and GMP, causing increased de novo purine synthesis. ❖ This results in the excessive production of uric acid; plus characteristic neurologic features, including self-mutilation (biting of lips and fingers) and involuntary movements. Formation of deoxynucleotides ❖ Deoxyribonucleotides (of purines and pyrimidines) are formed by reduction of the corresponding ribonucleoside diphosphates, e.g., ADP → Deoxy-ADP (dADP). ❖ The reaction is catalyzed by the enzyme ribonucleotide reductase with reduced thioredoxin as hydrogen donor. O- O- O- O- I I Base I I Base -O-P- O-P-O-CH -O-P- O-P-O-CH 2 O 2 II II Inhibitor: II II O O O dATP O O H H H H H H H H OH OH OH H Ribonucleoside diphosphate Deoxyribonucleoside diphosphate – Ribonucleotide reductase Thioredoxin (2 SH) H2O Thioredoxin (S-S) (reduced) oxidized Thioredoxin reductase NADP+ NADPH + H+ Formation of nucleoside di-and triphosphate ❖ Nucleotides (AMP, GMP, and IMP) are converted to the corresponding diphosphates by a base-specific nucleoside monophosphate kinase enzyme with ATP as phosphate donor. ❖ The nucleoside diphosphate is converted to the corresponding triphosphate by a nonspecific nucleoside diphosphate kinase enzyme, with ATP as phosphate donor. ATP ADP ATP ADP Nucleoside GMP GTP GMP GDP diphosphate kinase kinase Regulation of purine nucleotide synthesis Purine nucleotide synthesis is regulated by many ways, example: The concentration of PRPP is the most important factor in determining the rate of de novo synthesis of purines. The concentration of PRPP is, in turn, determined by the rates of its synthesis and utilization. The rate of synthesis of PRPP depends on the availability of ribose 5-phosphate and the activity of the enzyme PRPP synthetase. The activity of the enzyme PRPP synthetase is allosterically inhibited by AMP, ADP, GMP, and GDP. The utilization of PRPP is chiefly determined by the activity of the enzyme HGPRTase of the purine salvage system. ❖ Normally more PRPP is utilized for purine salvage than for de novo synthesis Thus, deficiency of HGPRTase markedly increases de novo synthesis of purines, leading to hyperuricemia. ❖ The enzyme glutamine-PRPP amidotransferase catalyzes the committed step of de novo synthesis of purine nucleotides It is allosterically activated by PRPP and inhiblied by IMP, AMP, and GMP. ❖ In addition, GTP is required for the conversion of IMP to AMP and ATP is required for the conversion of IMP to GMP. This increases the formation of one purine nucleotide when there is excess of the other. Ribose 5-phosphate – PRPP synthetase – PRPP + Glutamine-PRPP aminotransferase – – IMP – – + AS XMP + AMP GMP ADP GDP ATP GTP Purine catabolism Digestion Hyperuricemia and gout Catabolism Adenosine deaminase deficiency Excretion of uric acid Digestion ❖ Nucleic acids are split into nitrogenous bases (purines and pyrimidines), pentoses (ribose or deoxyribose) and inorganic phosphate. by: 1. Nucleases enzymes (in pancratic juice) 2. Nucleotidases, nucleosidases, and phosphatase (in the intestinal juice). ❖ Dietary purines and pyrimidines are not used to a large extent for the synthesis of tissue nucleic acids. ❖ Instead, the dietary purines are generally converted to uric acid by intestinal mucosal cells. Most of the uric acid enters the blood and excreted in the urine. For this reason, individuals with a tendency toward gout should be careful about consuming foods such as meats, sardines, or dried beans, which contain high amounts of nucleic acids. Catabolism 1. The nucleic acids are hydrolyzed by nucleases, forming the nucleotides AMP and GMP. 2. Nucleotides are further hydrolyzed by nucleotidases, forming a phosphate and the nucleosides: adenosine, inosine, and guanosine. 3. Adenosine undergoes hydrolytic deamination forming inosine and inosine is broken into hypoxanthine and ribose 1-phosphate. 4. Guanosine is broken into guanine and ribose-1-P. Guanine undergoes hydrolytic deamination forming xanthine. 5. In the liver, hypoxanthine is oxidized to xanthine, which is further oxidized to uric acid. 6. Both reactions are catalyzed by xanthine oxidase, which is a metalloflavoprotein containing FAD, molybdenum (Mo), and nonheme iron. The uric acid formed goes via the blood to the kidney to be excreted in the urine. NH2 O N N N HN Guanosine N Adenosine H2N N I Pi I Ribose H2O Ribose Purine nucleoside phosphorylase Adenosine deaminase Ribose 1-P O NH3 O N N HN HN Inosine Guanine N H2O H2N N I Pi H Ribose Guanase Purine nucleoside phosphorylase NH3 Ribose 1-P O O2 H2O2 O N HN N HN O N N H I H2O H Xanthine Xanthine oxidase O2 H2O Hypoxanthine [FAD, Fe, Mo] H2O2 O H HN N Uric acid O O N H Hyperuricemia and gout Uric acid is the main end product of purine catabolism in human liver. Plasma uric acid (urate) levels are 4-7 mg/dL for males and 3-6 mg/dL for females during fasting. Gout is a disease characterized by hyperuricemia. This leads to deposition of monosodium urate under the skin forming tophi, and in joint cartilage, leading to arthritis that usually starts in the joint of the big toe. 1- Increased production of uric acid ❖ This may be metabolic, by increased de novo synthesis of purine nucleotides, or dietary, due to increased ingestion of nucleic acids. A. Primary metabolic hyperuricemia i. Due to Increased activity of the key enzymes of de novo synthesis of purine nucleotides. These include the enzyme PRPP synthetase and glutamine-PRPP amidotransferase. ii. Complete deficiency of the enzyme HGPRTase (the Lesch-Nyhan syndrome). It is characterized by decreased purine salvage, leading to increased availability of PRPP for de novo synthesis of purine nucleotides. ❖ There is marked increase in the production of uric acid. B. Secondary metabolic hyperuricemia ❖ Examples include hemolytic anemia, polycythemia ( increased red blood cells by bone marrow), leukemia. All these conditions are associated with increased biosynthesis and catabolism of nucleic acids. 2. Decreased excretion (Renal hyperuricemia) A. Primary renal hyperuricemia: ❖ This is an inherited isolated defect in the kidneys caused by decreased tubular secretion of uric acid. B. Secondary renal hyperuricemia ❖ Chronic renal failure. Treatment of gout 1. Diet. Gouty patients should receive diet poor in nucleic acids; liver, kidney, heart, meat, and fish should be avoided. Milk, cheese, yogurt, and eggs are the recommended sources of proteins. 2. Uricosuric drugs. Gout may be treated by uricosuric drugs, which inhibit the renal tubular reabsorption of urate, increasing the excretion of urate in the urine. In such cases urine should be alkalinized to prevent precipitation of uric acid and stone formation in the renal tract. 3. Allopurinol is a structural analog of hypoxanthine.It is a substrate as well as a competitive inhibitor of the enzyme xanthine oxidase, inhibiting the formation of uric acid. Biosynthesis of pyrimidine nucleotides ❖ Almost all cells can synthesize their requirements of pyrimidines. ❖ The origin of the atoms comprising the pyrimidine nucleus is shown in the following diagram. 4 3 C 5 Glutamine N C Aspartic acid CO2 C C 2 N 6 1 ❖ Aspartate and carbamoyl phosphate form all components of the pyrimidine ring. ❖ Pyrimidine bases are first synthesized as the free base and then converted to a nucleotide. ❖ All reactions of pyrimidine synthesis occur in the cytosol except the dihydroorotate dehydrogenase reaction which occurs on the inner mitochondrial membrane. ❖ The biosynthesis of pyrimidines begins with the formation of carbamoyl phosphate in a reaction catalyzed by carbamoyl phosphate synthetase II. [Remember that. this cytosolic enzyme differs from the mitochondrial carbamoyl phosphate synthetase I needed for urea]. ❖ Ribose 5-phosphate is required to donate the sugar phosphate to form a nucleotide. ❖ The first pyrimidine nucleotide produced is orotate monophosphate (OMP). ❖ The OMP is converted to uridine monophosphate (UMP), which will become the precursor for both cytidine triphosphate (CTP) and deoxythymidine monophosphate (dTMP) production. Glutamine CO2 + 2 ATP Carbamoyl phosphate H2N synthetase II Carbamoyl I O=C 2 ADP + Pi phosphate I O ~ P HOOC Glutamate CH2 I Aspartic acid CH-COOH H2N Aspartate transcarbamoylase Pi HOOC N-Carbamoyl H2N CH2 I I aspartic acid O=C CH-COOH N Dihydroorotase H H2O Dihydroorotic acid NAD+ Dihydroorotate (mitochondria) dehydrogenase CO2 PP i PRPP NADH + H+ Orotidine Uridine monophosphate Orotic acid monophosphate OPRTase OMP O O decarboxylase II II HN HN O N O N I I R 5-P Glutamine CO 2 + 2 ATP +2 H2O (cytidine triphosphate) CTP H2N-COO ~ P Carbamoyl phosphate synthetase II Carbamoyl Glutamate 2 ADP + Pi phosphate ADP + Pi CTP synthetase Glutamate ATP Glutamine Aspartic acid Aspartic acid Aspartate transcarbamoylase Pi UTP N-Carbamoyl aspartic acid Kinase Dihydroorotase UDP Dihydroorotic acid Kinase Dehydrogenase (mitochondria) Uridine Orotidine monophosphate Orotic acid monophosphate Decarboxylase OPRTase ❖ dUMP is converted to dTMP by thymidylate synthase which uses N5,N10-methylene tetrahydrofolate as the source of the methyl group. ❖ Re-formation of THF requires the enzyme dihydrofolate reductase, which is inhibited by methotrexate. ❖ This explains the inhibitory effect of this drug on the biosynthesis of purines and thymine, inhibiting nucleic acid synthesis and cell division. Thus, methotrexate is used in the treatment of leukemia and cancers. O II CH2-THF DHF CH3 HN d-UMP Thymidylate synthase O N I R 5-P dTMP O HN O N 2-O POH C 3 2 O H2C NR dUMP O I HO N HN H N5, N10-Methylene- H2N N N tetrahydrofolate H 5-Fluorouracil Thymidylate - synthase 5-FdUMP Dihydrofolate Metho- NADPH + H+ dTMP Glycine trexate O Dihydrofolate CH3 - reductase HN O NADP+ 2-O N 3POH2C O Serine Tetrahydrofolate HO Pyrimidine salvage ❖Free pyrimidines are not salvaged. Pyrimidine nucleosides can be salvaged ATP ADP Uridine Uridine-cytidine UMP Cytidine kinase CMP ATP ADP Deoxycaytidine Deoxycytidine dCMP kinase Regulation of pyrimidine nucleotides biosynthesis Glutamine + CO2 Carbamoyl phosphate synthetase II + PRPP – ATP Carbamoyl phosphate Aspartate transcarbamoylase Mammalian cells Carbamoyl aspartate UTP CTP synthetase CTP Catabolism of pyrimidines ❖In the liver, free pyrimidines undergo further catabolism; ❖Cytosine undergoes hydrolytic deamination, forming uracil. ❖Uracil and thymine are first reduced to the dihydro-derivatives. ❖They are then hydrolyzed, liberating carbon dioxide and ammonia, forming -alanine and -aminoisobutyrate, respectively. O O NH2 HN CH3 H2O NH3 HN HN O O O N N N H H H Uracil Thymine Cytosine H2O H2O NH3 NH3 CO2 CO2 Urea -Aminoisobutyric -Alanine acid CH2.CH2.COOH CH2.CH.COOH I I I NH2 NH2 CH3 Disorders of pyrimidine metabolism Hereditary orotic aciduria 1. Type I hereditary orotic aciduria ❖ This disease is caused by deficiency of UMP synthase (orotate phosphoribosyltransferase + OMP decarboxylase). 1. Type II hereditary orotic aciduria ❖ It is caused by deficiency of OMP decarboxylase. ✓ Both types are associated with the excretion of excessive quantities of orotic acid in the urine, severe anemia, and growth retardation. ✓ Both types can be treated by uridine, which becomes converted to UMP by the enzyme kinase.