Molecular Biology of Gene Lecture Notes PDF
Document Details
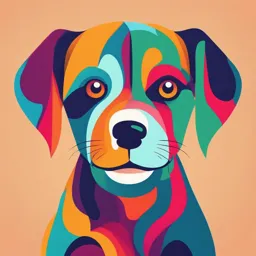
Uploaded by IdolizedLandArt
Dr.Nuha Joseph Kandala
Tags
Summary
This document is a lecture on molecular biology of gene, focusing on DNA and its properties, including the process of reversible strand separation and melting. The lecture explains how the temperature, pH, and salt concentrations affect the melting temperature.
Full Transcript
## Molecular Biology of Gene ### Lecture (3): ### DNA Can Undergo Reversible Strand Separation During replication and transcription of DNA, the strands of the double helix must separate to allow the internal edges of the bases to pair with the bases of the nucleotides being polymerized into new c...
## Molecular Biology of Gene ### Lecture (3): ### DNA Can Undergo Reversible Strand Separation During replication and transcription of DNA, the strands of the double helix must separate to allow the internal edges of the bases to pair with the bases of the nucleotides being polymerized into new complementary polynucleotide chains. ### Melting Separates DNA Strands; Cooling Anneals Them Hydrogen bonds are rather weak, but since a molecule of DNA usually contains millions of base pairs, the added effect of millions of weak bonds is strong enough to keep the two strands together (Figure. 2). When DNA is heated, the hydrogen bonds begin to break and the two strands will eventually separate if the temperature rises high enough. This is referred to as "melting" or denaturation and each DNA molecule has a melting temperature (Tm) that depends on its base composition. can be induced experimentally by increasing the temperature of a solution of DNA. As the thermal energy increases, the resulting increase in molecular motion eventually breaks the hydrogen bonds and other forces that stabilize the double helix. The strands then separate, driven apart by the electrostatic repulsion of the negatively charged deoxyribose-phosphate backbones of the two strands. Near the denaturation temperature, a small increase in temperature causes a rapid, nearly simultaneous loss of the multiple weak interactions holding the strands together along the entire length of the DNA molecules. Because the stacked base pairs in duplex DNA absorb less ultraviolet (UV) light than the unstacked bases in single-stranded DNA this change leads to an abrupt increase in the absorption of UV light. This phenomenon, known as hyperchromicity (Figure-la), is useful for monitoring DNA denaturation. The Hypochromic effect describes the decrease in the absorbance of ultraviolet light in a double stranded DNA compared to its single stranded . **melting temperature (Tm)**: The temperature at which half of the DNA is single stranded is called the melting temperature. The melting temperature of all DNA molecules is not the same.on the other meanings, the melting temperature (Tm) at which DNA strands separate depends on several factors, depends upon: 1. the length of DNA (that depends on its base composition.). 2. molecules that contain a greater proportion of G·C pairs require higher temperatures to denature because the three hydrogen bonds in G·C pairs make these base pairs more stable than AT pairs, which have only two hydrogen bonds. Indeed, the percentage of G·C base pairs in a DNA sample can be estimated from its Tm (Figure -1b). 3. The melting temperature is affected by the pH and salt concentration of the solution. So these must be standardized if comparisons are to be made. Extremes of pH disrupt hydrogen bonds. A highly alkaline pH deprotonates the bases which abolishes their ability to form hydrogen bonds and at pH > 11.3 DNA is fully denatured. Conversely, a very low pH causes excessive protonation, which also prevents hydrogen bonding. When DNA is deliberately denatured by pH, alkaline treatment is used because unlike acid, this does not affect the glycosidic bonds between bases and deoxyribose. DNA is relatively more stable at higher ionic concentrations. This is because ions suppress the electrostatic repulsion between the negatively charged phosphate groups on the backbone and hence exert a stabilizing effect. In pure water, DNA will melt even at room temperature. A spectrophotometer detects the amount absorbed when light is passed through a solution containing DNA. This is compared with the light absorbed by a solution containing no DNA to determine the amount absorbed by the DNA itself. 4. The ion concentration of the solution also influences the Tm because the negatively charged phosphate groups in the two strands are shielded by positively charged ions. When the ion concentration is low, this shielding is decreased, thus increasing the repulsive forces between the strands and reducing the Tm. Agents that destabilize hydrogen bonds, such as formamide or urea, also lower the Tm. Finally, extremes of pH denature DNA at low temperatures. At low (acid) pH, the bases become protonated and thus positively charged, repelling each other. At high (alkaline) pH, the bases lose protons and become negatively charged, again repelling each other because of their similar charges. In cells, pH and temperature are, for the most part, maintained at a constant level. These features of DNA denaturation are most useful for manipulating DNA in a laboratory setting. The single-stranded DNA molecules that result from denaturation form random coils without an organized structure. Lowering the temperature, increasing the ion concentration, or neutralizing the pH causes two complementary strands to reassociate into a perfect double helix. The extent of such renaturation is dependent on time, the DNA concentration, and the ion concentration. Two DNA strands that are not related in sequence will remain as random coils and will not renature, but they will not inhibit complementary DNA partner strands from finding each other and renaturing. Denaturation and renaturation of DNA are the basis of nucleic acid. ### Figure(1):GC content of DNA affects melting temperature. The temperature at which DNA denatures increases with the proportion of G·C pairs. (a) Melting of double stranded DNA can be monitored by its absorption of UV light at 260 nm. As regions of double-stranded DNA unpair, the absorption of light by those regions increases almost twofold. Light absorption by single-stranded DNA changes much less as the temperature increased. (b) The temperature at which half the bases in a double stranded DNA sample have denatured is denoted Tm (for "temperature of melting"). The Tm is a function of the G.C content of the DNA; The greater the G+C percentage, the higher the Tm. ### Figure (2): Melting of DNA: DNA strands separate, or “melt," with increasing temperature. This curve shows the measurement of DNA separation by ultraviolet absorption. As the temperature increases more UV is absorbed by the individual strands. The Tm or melting temperature is the point at which half of the double stranded DNA has separated. During the melting process regions rich in A=T melt first since these base pairs have only two hydrogen bonds. The melting temperature of a long DNA molecule can be calculated according to the following equation: Tm = 16.5(log [Na+]) + 0.41(%GC) + 81.5 °C The equation above breaks down, and the following estimation (sometimes called the Wallace rule) is used to determine the Tm Tm = 2(AT) + 4(GC) ### Reversible Denaturing of DNA Heating a DNA sample will break the relatively weak hydrogen bonds connecting the two strand of the helix. The separation of the two DNA strands will be effect on the physical properties of DNA. One of these changes is the way in which DNA absorbs UV light. DNA absorbs light at a wavelength of 260 nm due to the presence of alternating single and double bonds in the DNA bases. A solution of double-stranded, native DNA, with a concentration of 0.05 mg/mL, has an absorbance (or optical density, OD) of about 1.0 at the 260 nm peak. When a DNA helix is denatured to become single strands, e.g. by heating, the absorbance increases. The same 0.05 mg mL double-stranded DNA solution that we used above will have an absorbance of 1.5 when in the single-stranded form. This type of absorbance data is often expressed in the form shown below: 1.0 A260 nm double-stranded DNA = 50 µg/mL 1.5 A260 nm single-stranded DNA = 33 µg/mL ### Melting Separates DNA Strands; Cooling Anneals Them Melting is followed by measuring the absorption of ultraviolet (UV) light at a wavelength of 260 nm (the wavelength of maximum absorption), since disordered DNA absorbs more UV light than a double helix . Overall, the higher the proportion of GC base pairs, the higher the melting temperature of a DNA molecule. This is because AT base pairs are weaker, as they have only two hydrogen bonds, as opposed to GC pairs, which have three. In addition, the stacking of GC base pairs with their neighbors is also more favorable than for AT base pairs. In the early days of molecular biology, melting temperatures were used to estimate the percentage of GC versus AT in samples of DNA. DNA base compositions are often cited as the GC ratio. The GC content (% G + C) is calculated from the fractional composition of bases as follows: related sequences **GC ratio** The amount of G plus C relative to all four bases in a sample of DNA. The GC ratio is usually expressed as a percentage: G+C A+T+G+C ×100% GC contents for the DNA from different bacterial species vary from 20 percent to 80 percent, with E. coli having a ratio of 50 percent. Despite this, there is no correlation between GC content and optimum growth temperature. Apparently this is because the genomes of bacteria are circular DNA molecules with no free ends and this greatly hinders unraveling at elevated temperatures. In fact, small circular DNA molecules, like plasmids, may remain base paired up to 110-120°C. In contrast, the range of GC contents for **animals** (which have linear chromosomes) is much narrower, from approximately 35–45 percent, with **humans** having **40.3** percent GC. As a DNA molecule melts, regions with a high local concentration of AT pairs will melt earlier and GC-rich regions will stay double stranded longer. When DNA is replicated, the two strands must first be pulled apart at a region known as the origin of replication. The DNA double helix must also be opened up when genes are transcribed to make mRNA molecules. In both cases, AT-rich tracts are found where the DNA double helix will be opened up more readily. If the single strands of a melted DNA molecule are cooled, the single DNA strands will recognize their partners by base pairing and the double stranded DNA will reform. This is referred to as annealing or renaturation. For proper annealing, the DNA must be cooled slowly to allow the single strands time to find the correct partners. ### Hybrid DNA Formed by Melting and Re- Annealing The temperature should remain moderately high to disrupt random H-bond formation over regions of just one or a few bases. A temperature 20-25°C below the Tm is suitable. If DNA from two different, but related, sources is melted and reannealed, hybrid DNA molecules may be obtained (Figure-3). Two double-stranded DNA molecules with similar but not identical sequences are heated to form single-stranded DNA. The mixture is cooled slowly, allowing the sequences to anneal. Sometimes, the two original sequences will anneal into the parental molecules. Occasionally, the two will mix, forming a hybrid molecule with one green strand of DNA and one red strand. Since the sequences are not identical, there are regions that do not base pair. ### Figure (3):Annealing of DNA. When pure DNA is heated and cooled the two strands pair up again, i.e., they re-anneal. When DNA from two related sources is heated and re-annealed, hybrid strands may form. The likelihood of hybridization depends on how closely related the two sequences are. ### Hybridization of DNA and RNA Hybridization, a powerful technique used to study the relatedness of two DNA samples and to detect and isolate specific DNA molecules in a mixture containing numerous different DNA sequences. On the other hand Hybridization of DNA and RNA was originally used to estimate the relatedness of different organisms, especially bacteria where the amount of DNA is relatively small, in the days before direct sequencing of DNA became routine. Other uses for hybridization include detection of specific gene sequences and gene cloning. Several extremely useful techniques that are still current and are based on the hybridization of DNA and RNA . A wide range of methods based on hybridization is used for analysis in molecular biology. The basic idea in each case is that a known DNA sequence acts as a "probe." Generally the probe molecule is labeled by radioactivity or fluorescence for ease of detection. The probe is used to search for identical or similar sequences in the experimental sample of target molecules. Both the probe and the target DNA must be treated to give single-stranded DNA molecules that can hybridize to each other by base pairing. In the previous example, the probe DNA would be the human hemoglobin DNA since the sequence is already known. The gorilla DNA would be the sample of target molecules. The formation of hybrid DNA molecules has a wide variety of uses. Example: How closely two DNA molecules are related may be tested? To do this, 1. A sample of the first DNA molecule is heated to melt it into single stranded DNA. 2. The single strands are then attached to a suitable filter. Next, the filter is treated chemically to block any remaining sites that would bind DNA. Then, after melting, a solution of the second DNA molecule is poured through the filter (Figure-4). 3. Some of the single strands of DNA molecule No. 2 will base pair with the single strands of DNA molecule No. 1 and will stick to the filter, DNA molecule No. 2 must be labeled by radioactivity, fluorescence or some other way to enable its detection.) 4. The more closely related the two molecules are, the more hybrid molecules will be formed and the higher the proportion of molecule No. 2 that will be bound by the filter. ### Figure (4): Relatedness of DNA by Filter Hybridization (A) DNA No. 1 is denatured and attached to a filter. (B) When DNA No. 2 is added to the filter, some of the DNA strands will hybridize, provided that the sequences are similar enough. If the sequence is identical, all of the single-stranded red DNA should hybridize to strands of green DNA. If the sequences are very different, little or none of the green DNA will hybridize with the red. For example, if the DNA for a human gene, such as hemoglobin, was fully melted and bound to a filter, then DNA for the same gene but from different animals could be tested. We might expect gorilla DNA to bind strongly, frog DNA to bind weakly and mouse DNA to be intermediate. [Several variants of nucleic acid hybridization are in use. This version, involving the binding of DNA to DNA is known as Southern Blotting. Another use for hybridization is in isolating genes for cloning. Suppose we already have the human hemoglobin gene and want to isolate the corresponding gorilla gene. First the human DNA is bound to the filter as before. Then gorilla DNA is cut it into short segments with a suitable restriction enzyme The gorilla DNA is heated to melt it into single strands and poured over the filter. The DNA fragment that carries the gorilla gene for hemoglobin will bind to the human hemoglobin gene and remain stuck to the filter. Other, unrelated genes will not hybridize. A wide range of methods based on hybridization is used for analysis in molecular biology. The basic idea in each case is that a known DNA sequence acts as a "probe." Generally the probe molecule is labeled by radioactivity or fluorescence for ease of detection. The probe is used to search for identical or similar sequences in the experimental sample of target molecules. Both the probe and the target DNA must be treated to give single-stranded DNA molecules that can hybridize to each other by base pairing. In the previous example, the probe DNA would be the human hemoglobin. DNA since the sequence is already known. The gorilla DNA would be the sample of target molecules. ### Southern, Northern, and Western Blotting Isolating new genes from related species provides a wealth of information for a scientist. Many times, scientists that are studying human genes need to find similar genes ### Southern Blotting: DNA:DNA Hybridization Southern blotting requires the target DNA to be cut into smaller fragments and run on an agarose gel. The fragments are denatured chemically to give single strands, then transferred to a nylon membrane. Notice that the DNA is invisible both in the gel and on the membrane. A radioactive probe (also single-stranded) is passed over the membrane. When the probe DNA finds a related sequence, a hybrid molecule is formed. Surplus probe that has not bound is washed away. Photographic film is placed on top of the membrane. The location of radioactive hybrid molecules is revealed by black bands on the film. **TABLE 21.01 Different Types of Blotting** | Type of Blotting | Molecule on Membrane | Probe Molecule | |---|---|---| | Southern blotting | DNA | DNA | | Northern blotting | RNA | DNA | | Western blotting | Protein | Antibody | | South-Western blotting | Protein | dsDNA | in other organisms such as yeast or Drosophila. Many scientists use **Southern blotting** to identify the gene in a different organism. Southern blotting is a technique in which one DNA sample is hybridized to another DNA sample. Suppose we have a large DNA molecule, such as the yeast chromosome, and we wish to locate the particular gene whose sequence is similar to the human gene of interest. First the target or yeast DNA is cut with a restriction enzyme, and the fragments are separated by gel electrophoresis. The double-stranded fragments are melted into single-stranded fragments by soaking the gel in alkaline denaturing solution such as sodium hydroxide. Then the DNA fragments are transferred to a nylon membrane. Finally, the membrane is dipped in a solution of labeled DNA probe molecules, in this example, a radioactively labeled piece of the human gene (Fig. 21.32). The probe binds only to those fragments with corresponding DNA, the filter will be "hot" or radioactive in that area, and if a piece of photographic film is placed over the filter, a black spot corresponding to the hybrid molecule will appear. Southern blotting only refers to hybridization of DNA to DNA. Although Southern blotting was actually named after its inventor, Edward Southern, it set a geographical trend for naming other types of hybridization techniques (Table 21.01). **Northern blotting** refers to hybridization that uses **RNA** as the target molecule and DNA as a probe. For example, DNA probes may be used to locate messenger RNA molecules that correspond to the same gene. The mixture of RNA is run on the gel and transferred to the filter. The filter is then probed just as above. **Western blotting** does not even involve nucleic acid hybridization. Proteins are separated on a gel, transferred to a membrane and detected by antibodies or other methods. Since Western blotting applies to proteins. In **South-Western blotting**, proteins suspected of binding to DNA are stuck to the membrane for testing. The probe is a DNA fragment, in this case double stranded, since DNA binding proteins normally bind to the DNA double helix. ### Zoo Blotting Zoo blotting is not a distinct method but a neat trick using Southern blotting. One problem encountered when cloning human genes is that most DNA in higher animals is non-coding DNA, yet most scientists want the coding regions. The question is how to identify coding regions in the large amount of non-coding DNA. During evolution, the base sequence of non-coding DNA mutates and changes rapidly, whereas coding sequences change much more slowly and can still be recognized after millions of years of divergence between two species Therefore DNA is extracted from a series of related animals, such as a human, monkey, mouse, hamster, cow, etc. Samples in this DNA "zoo" are each cut up with a suitable restriction enzyme and the fragments are run on a gel and transferred to a nylon membrane. They are probed using DNA that is suspected of being human coding DNA. If a DNA sample really does include a coding sequence, it will probably hybridize with some fragment of DNA from most other closely related animals (Figure. 5). ### Zoo Blotting Reveals Coding DNA A specialized form of Southern blotting, called zoo blotting, is used to distinguish coding DNA from non-coding regions. The target DNA includes several samples of genomic DNA from different animals, hence the term "zoo". The probe is a segment of human DNA that may or may not be from a coding region. Blotting is carried out as usual. On the left, the only hybrid seen was between the probe and the human DNA. Therefore, related sequences were not found in other species and the probe is probably non-coding DNA. In the example on the right, the probe binds to related sequences in other animals; therefore, this piece of DNA is probably from a coding region. ### Fluorescence in Situ Hybridization (FISH) All the previous techniques require the scientist to isolate the DNA, RNA or protein from its cellular environment. In contrast, Fluorescence in Situ Hybridization, better known as FISH, is used to detect the presence of a gene, or the corresponding messenger RNA, within the actual cell (Figure. 6). DNA sequences from the gene of interest must first be generated for use as a probe. These may be obtained by cloning the gene . practice, it is rarely necessary to use the whole gene sequence as a probe, unless distinguishing or, more usually nowadays, amplified by PCR between closely related genes is essential. As the name indicates, the DNA probe is labeled with a fluorescent dye whose localization will eventually be observed under a fluorescence microscope. The tissue or cell must also be treated to denature the chromosomal DNA, but this is done on the actual tissue section, leaving the DNA within the nuclei. A thin section of tissue from a particular animal, a mouse for example, may be treated with a DNA probe for a known mouse gene. In this case, the mouse probe will hybridize to the mouse DNA in the nucleus of all the cells. This tells us that the genes are in the nucleus, which we knew anyway. Some more useful applications are as follows: 1. Using a virus gene as a probe reveals which cells contain virus genes, and whether the virus genes are in the cytoplasm or have penetrated the nucleus. 2. Besides DNA within the nucleus, FISH can be used to identify where a particular gene is in the metaphase chromosome. First, a chromosome smear can be made on a microscope slide, and then probed with a fluorescently labeled gene of interest. The place where the probe binds reveals which chromosome carries the gene corresponding to the probe. (Figure. 7). 3. A DNA probe can be used to detect mRNA within the target tissue since one of the two strands of the denatured DNA will bind to the RNA. Since mRNA is already single-stranded, the cells do not have to be treated with high heat or chemical denaturants. Cells actively transcribing the gene of interest will have high levels of the corresponding mRNA, which will bind the probe and light up (Fig. 8). The greater the gene expression, the brighter the cell will fluoresce. Identifying the location of a particular mRNA can be real helpful when comparing tissues. For example, comparing the amount of mRNA for a particular gene in liver cells versus heart cells can help determine the function of the gene of interest. Levels of many mRNA molecules are low and hence would give only a weak signal by FISH. In practice, such mRNA is often amplified by RT-PCR before detection .Alternatively, more modern and more sensitive techniques, such as microarrays are used for mRNA detectio ### Figure -6: Fluorescence in Situ Hybridization-Principle A cell with intact DNA in its nucleus is treated to denature the DNA, forming single-stranded regions. The fluorescently labeled DNA probe is added, and the single-stranded probe can anneal with the corresponding sequence inside the nucleus. The hybrid molecule will fluoresce when the light from a fluorescence microscope excites the tag on the probe. This technique can localize the gene of interest to different areas of the nucleus or to individual chromosomes. ### Figure -7: FISH Το Localize Genes on Chromosomes FISH can localize a gene to a specific place on a chromosome. First, metaphase chromosomes are isolated and attached to a microscope slide. The chromosomal DNA is denatured into single-stranded pieces that remain attached to the slide. The fluorescent probe hybridizes to the corresponding gene. When the slide is illuminated, the hybrid molecules fluoresce and reveal the location of the gene of interest. ### Figure -8: FISH To Detect and Measure mRNA Levels A variety of different mRNA species are expressed at any one time within a tissue. Not all cells express the same genes or express them at the same level. If the target cells are probed with fluorescently labeled DNA (red), this will bind to the corresponding mRNA (blue). Notice that the probe DNA does not bind to the nuclear DNA because in this procedure the cells were not treated to denature the chromosomal DNA. The target gene in this example was only expressed in two of the cells **Denaturation** : When used of proteins or other biological polymers, refers to the loss of correct 3-D structure **melting**: When used of DNA, refers to its separation into two strands as a result of heating **melting temperature (Tm)** : The temperature at which the two strands of a DNA molecule are half unpaired. **annealing**: The re-pairing of separated single strands of DNA to form a double helix **hybrid DNA**: Artificial double stranded DNA molecule made by pairing two single strands from two different sources **hybridization**: Pairing of single strands of DNA or RNA from two different (but related) sources to give a hybrid double helix **renaturation**: Re-annealing of single-stranded DNA or refolding of a denatured protein to give the original natural 3-D structure.