Physiology II Past Paper (2024) PDF
Document Details
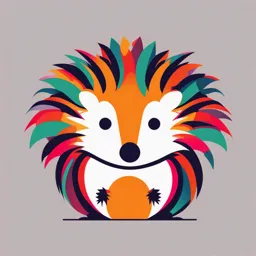
Uploaded by UnfetteredImagery
2024
Tags
Summary
This document covers basic concepts of endocrine regulation. It discusses the different types of hormones, their actions on target cells, synthesis, secretion, and transport in the blood. The material explores how hormones maintain homeostasis in various aspects of the body.
Full Transcript
ﻟﺠﻨﺔ ﻋﻤﺪاء +ﻠ-ﺎت اﻟﺼ-ﺪﻟﺔ ﻟﺠﻨﺔ ﺗﻮﺣ-ﺪ ﻣﻨﻬﺎج ﻣﺎدة )(Physiology II Physiology II اﻟﻤﺮﺣﻠﺔ اﻟﺜﺎﻧ,ﺔ 2024 SﻟUﻠ-ﺎت اﻟﺼ-ﺪﻟﺔ ﻟﻠﻌﺎمﺗﻢ اﻋﺪاد وﻣﺮاﺟﻌﺔ ﻫﺬا اﻟﻤﻨﻬﺞ اﻟﻤﻮﺣﺪ ﻟﻼﻣﺘﺤﺎن اﻟﺘﻘT RQ f اﻟﺪرا 2024-2023...
ﻟﺠﻨﺔ ﻋﻤﺪاء +ﻠ-ﺎت اﻟﺼ-ﺪﻟﺔ ﻟﺠﻨﺔ ﺗﻮﺣ-ﺪ ﻣﻨﻬﺎج ﻣﺎدة )(Physiology II Physiology II اﻟﻤﺮﺣﻠﺔ اﻟﺜﺎﻧ,ﺔ 2024 SﻟUﻠ-ﺎت اﻟﺼ-ﺪﻟﺔ ﻟﻠﻌﺎمﺗﻢ اﻋﺪاد وﻣﺮاﺟﻌﺔ ﻫﺬا اﻟﻤﻨﻬﺞ اﻟﻤﻮﺣﺪ ﻟﻼﻣﺘﺤﺎن اﻟﺘﻘT RQ f اﻟﺪرا 2024-2023 Xﻣﻦ ﻗ`ﻞ اﺳﺎﺗﺬة ﻣﺘﺨﺼﺼ f e dﻟﺪﻳﻬﻢ ﺧijة ﻛﺒieة mTاﻟﺘﺪرnﺲ T f . Sﻟﻘﺪ tﺬل اﻻﺳﺎﺗﺬة ﻗﺼﺎرى ﺟﻬﻮدﻫﻢ mTﺟﻤﻊ اﻟﻤﻌﻠﻮﻣﺎت وﺣﺮﺻﻮا واﻟﻌﻤﻞ اﻻqﺎدT r ﻋ yﺗﺮﺗﺒﻬﺎ وﺗﻨﻈ-ﻤﻬﺎ ﻟﺘﻜﻮن واﺿﺤﺔ }ﺴieة ﻋ yﻃﻠﺒ€ﻨﺎ اﻻﻋﺰاء .ﻧﺄﻣﻞ ﻣﻦ ﻃﻠﺒ€ﻨﺎ f اﻻﻋﺰاء اﻻﺳﺘﻔﺎدة ﻣﻨﻪ mTﻃ†Rﻘﻬﻢ ا‡ اﻟﻨﺠﺎح واﻟﺘﻔﻮق ،و‹ اﻟﻤﻮﻓﻖ Chapter 16 Basic Concepts of Endocrine Regulation In general, endocrine physiology is concerned with maintaining various aspects of homeostasis. The mediators of such control mechanisms are soluble factors known as hormones. The word hormone was derived from the Greek horman, meaning to set in motion. The endocrine system differs from other physiological systems in that it cannot be distinctly defined based on anatomical boundaries. It operates as a distributed network comprising glands and circulating messengers, often under the influence of the central nervous system, the autonomic nervous system, or both. Evolution of Hormones &Their Actions on Target Cells Hormones comprise steroids, amines, and peptides. Peptide hormones are by far the most numerous. Many hormones can be grouped into families reflecting their structural similarities as well as the similarities of the receptors they activate. However, the number of hormones and their diversity increases as one moves from simple to higher life forms, reflecting the added challenges in providing for homeostasis in more complex organisms. For example, among the peptide hormones, several are heterodimers that share a common α chain, with specificity being conferred by the β-chain. In the specific case of thyroid-stimulating hormone (TSH), follicle-stimulating hormone (FSH), and luteinizing hormone (LH), there is evidence that the distinctive β-chains arose from a series of duplications of a common ancestral gene. For these and other hormones, moreover, this molecular evolution implies that hormone receptors also need to evolve to allow for the spreading of hormone actions/specificity. This was accomplished by co-evolution of the basic G-protein–coupled receptors (GPCR) and receptor tyrosine kinases that mediate the effects of peptide and amine hormones that act at the cell surface. Steroids and thyroid hormones are distinguished by their predominantly intracellular sites of action, since they can diffuse freely through the cell membrane. They bind to a family of largely cytoplasmic proteins known as nuclear receptors. Upon ligand binding, the receptor–ligand complex translocates to the nucleus where it either homodimerizes, or associates with a distinct liganded nuclear receptor to form a heterodimer. In either case, the dimer binds to DNA to either increase or decrease gene transcription in the target tissue. HORMONE SECRETION SYNTHESIS & PROCESSING The regulation of hormone synthesis depends on their chemical nature. For peptide hormones as well as hormone receptors, synthesis is controlled predominantly at the level of transcription. For amine and steroid hormones, synthesis is controlled indirectly by regulating the production of key synthetic enzymes as well as by substrate availability. Interestingly, the majority of peptide hormones are synthesized initially as much larger polypeptide chains, and then processed intracellularly by specific proteases to yield the final hormone molecule. In some cases, multiple hormones may be derived from the same initial precursor, depending on the specific processing steps present in a given cell type. Presumably, this provides for a level of genetic “economy.” It is also notable that the hormone precursors themselves are typically inactive. This may be a mechanism that provides for an additional measure of regulatory control, or, in the case of thyroid hormones, may dictate the site of highest hormone availability. The synthesis of all of the proteins/peptides discussed above is subject to the normal mechanisms of transcriptional control in the cell. In addition, there is provision for exquisitely specific regulation by other hormones, since the regulatory regions of many peptide hormone genes contain binding motifs for the nuclear receptors discussed above. For example, thyroid hormone directly suppresses TSH expression via the thyroid hormone receptor. These specific mechanisms to regulate hormone transcription are essential to the function of feedback loops, as will be addressed in greater detail below. In some cases, the abundance of selected hormones may also be regulated via effects on translation. For example, elevated levels of circulating glucose stimulate the translation of insulin mRNA. These effects are mediated by the ability of glucose to increase the interaction of the insulin mRNA with specific RNA-binding proteins, which increase its stability and enhance its translation. The net effect is a more precise and timely regulation of insulin levels, and thus energy metabolism, than could be accomplished with transcriptional regulation alone. The precursors for peptide hormones are processed through the cellular machinery that handles proteins destined for export, including trafficking through specific vesicles where the propeptide form can be cleaved to the final active hormones. Mature hormones are also subjected to a variety of posttranslational processing steps, such as glycosylation, which can influence their ultimate biological activity and/or stability in circulation. Ultimately, all hormones enter either the constitutive or regulated secretory pathway. SECRETION The secretion of many hormones is via a process of exocytosis of stored granules. The exocytotic machinery is activated when the cell type that synthesizes and stores the hormone in question is activated by a specific signal, such as a neurotransmitter or peptide-releasing factor. One should, however, contrast the secretion of stored hormones with that of those that are continually released by diffusion (eg, steroids). Control of the secretion of the latter molecules occurs via kinetic influences on the synthetic enzymes or carrier proteins involved in hormone production. For example, the steroidogenic acute regulatory protein (StAR) is a labile protein whose expression, activation, and deactivation are regulated by intracellular signaling cascades and their electors, including a variety of protein kinases and phosphatases. StAR traffics cholesterol from the outer to the inner membrane leaflet of the mitochondrion. Because this is a rate-limiting first step in the synthesis of the steroid precursor, pregnenolone, this arrangement permits changes in the rate of steroid synthesis, and thus secretion, in response to homeostatic cues such as trophic hormones, cytokines, and stress (Figure 16–1). An additional complexity related to hormone secretion relates to the fact that some hormones are secreted in a pulsatile manner. Secretion rates may peak and ebb relative to circadian rhythms, in response to the timing of meals, or as regulated by other pattern generators whose periodicity may range from milliseconds to years. Pulsatile secretion is often related to the activity of oscillators in the hypothalamus that regulate the membrane potential of neurons, in turn secreting bursts of hormone releasing factors into the hypophysial blood flow that then cause the release of pituitary and other downstream hormones in a similar pulsatile manner. There is evidence that these hormone pulses convey different information to the target tissues that they act upon compared to a steady exposure to a single concentration of the hormone. Therapeutically, pulsatile secretion may pose challenges if, due to deficiency, it proves necessary to replace a particular hormone that is normally secreted in this way. FIGURE 16–1 Regulation of steroid biosynthesis by the steroidogenic acute regulatory protein (StAR). Extracellular signals activate intracellular kinases that, in turn, phosphorylate transcription factors that upregulate StAR expression. StAR is activated by phosphorylation, and facilitates transfer of cholesterol from the outer to inner mitochondrial membrane leaflet. This then allows conversion of cholesterol into pregnenolone, which is the first intermediate in the steroid biosynthetic pathway. HORMONE TRANSPORT IN THE BLOOD In addition to the rate of secretion and its nature (steady vs. pulsatile), several factors influence the circulating levels of hormones. These include the rates of hormone degradation and/or uptake, receptor binding and availability of receptors, and the affinity of a given hormone for plasma carriers (Figure 16–2). Stability influences the circulating half-life of a given hormone and has therapeutic implications for hormone replacement therapy, in addition to those posed by pulsatile secretion as discussed above. Plasma carriers for specific hormones have several important physiologic functions. First, they serve as a reservoir of inactive hormones and thus provide a hormonal reserve. Bound hormones are typically prevented from degradation or uptake. Thus, the bound hormone reservoir can allow fluctuations in hormonal levels to be smoothed over time. Plasma carriers also restrict the access of the hormone to some sites. Ultimately, plasma carriers may be vital in modulating levels of the free hormone in question. Typically, it is only the free hormone that is biologically active in target tissues or can mediate feedback regulation (see below) since it is the only form able to access the extravascular compartment. Catecholamine and most peptide hormones are soluble in plasma and are transported as such. In contrast, steroid hormones are hydrophobic and are mostly bound to large proteins called steroid binding proteins (SBP), which are synthesized in the liver. As a result, only small amounts of the free hormone are dissolved in the plasma. Specifically, sex hormone–binding globulin (SHBG) is a glycoprotein that binds to the sex hormones, testosterone and 17β-estradiol. Progesterone, cortisol, and other corticosteroids are bound by transcortin. The SBP-hormone complex and the free hormone are in equilibrium in the plasma, and only the free hormone can diffuse across cell membranes. SBP have three main functions: they increase the solubility of lipid-based hormones in the blood; they reduce the rate of hormone loss in the urine by preventing the hormones from being altered in the kidney; and as mentioned above, they provide a source of hormone in the bloodstream that can release free hormone as the equilibrium changes. It follows that an additional way to regulate the availability of hormones that bind to carrier proteins, such as steroids, is to regulate the expression and secretion of the carrier proteins themselves. This is a critical mechanism that regulates the bioavailability of thyroid hormones, for example. In a pathophysiologic setting, some medications can alter levels of binding proteins or displace hormones that are bound to them. In addition, some binding proteins are promiscuous and bind multiple hormones (eg, SHBG). These observations may have clinical implications for endocrine homeostasis, since free hormones are needed to feedback and control their rates of synthesis and secretion (see below). Finally, the anatomic relationship of sites of release and action of hormones may play a key role in their regulation. For example, several hormones are destroyed by passage through the pulmonary circulation or the liver. This may markedly curtail the temporal window within which a given hormone can act. FIGURE 16–2 Summary of factors that determine the level of free hormones circulating in the bloodstream. Factors that increase (green upward arrow) or decrease (red downward arrow) hormone levels are shown. Free hormones also equilibrate with the forms bound to either receptors or plasma carrier proteins. HORMONE ACTION Hormones exert a wide range of distinctive actions on a huge number of target cells to effect changes in metabolism, the release of other hormones and regulatory substances, changes in ion channel activity, and cell growth, among others. Ultimately, the concerted action of the hormones of the body ensures the maintenance of homeostasis. Indeed, all hormones affect homeostasis to some degree. However, a subset of the hormones, including thyroid hormone, cortisol, parathyroid hormone, vasopressin, mineralocorticoids, and insulin, are the key contributors to homeostasis (Table 16–1). Hydrophilic hormones, including peptides and catecholamines, exert their acute effects by binding to cell surface receptors. Most of these are from the GPCR family. Hydrophobic hormones, on the other hand, predominantly exert their actions via nuclear receptors. Two classes of nuclear receptors are important in endocrine physiology. TABLE 16–1 Major hormonal contributors to homeostasis. The first class provides direct stimulation of transcription via induction of the binding of a transcriptional co-activator when the hormonal ligand is bound. In the second class, hormone binding triggers simultaneous dislodging of a transcriptional co-repressor and recruitment of a co-activator. The latter class of receptor allows for a wider dynamic range of regulation of the genes targeted by the hormone in question. PRINCIPLES OF FEEDBACK CONTROL A final general principle that is critical for endocrine physiology is that of feedback regulation. This holds that the responsiveness of target cells to hormonal action subsequently “feeds back” to control the inciting endocrine organ. Feedback can regulate the further release of the hormone in either a negative feedback or (more rarely) a positive feedback loop. Positive feedback relates to the enhancement or continued stimulation of the original release mechanism/stimulus. Such mechanisms are only seen in settings that need to gather momentum for an eventual outcome, such as parturition. Negative feedback is a far more common control mechanism and involves the inhibition or dampening of the initial hormone release mechanism/stimulus. A general scheme for feedback inhibition of endocrine axes is depicted in Figure 16–3. FIGURE 16–3 Summary of feedback loops regulating endocrine axes. CNS, central nervous system. In general, the endocrine system uses a network of feedback responses to maintain a steady state. Negative feedback control systems such as those described are the most common feedback/homeostatic systems in the body. Feedback control loops also provide for diagnostic strategies in evaluating patients with suspected endocrine disorders. For example, in a patient being evaluated for hypothyroidism, normal levels of TSH tend to rule out a primary defect at the level of the thyroid gland itself, and rather suggest that a defect at the level of the anterior pituitary should be sought. Conversely, if TSH is elevated, it suggests that the normal ability of circulating thyroid hormone to suppress TSH synthesis has been lost, likely due to a reduction in the ability of the thyroid gland to synthesize the hormone. Chapter 17 Hypothalamic Regulation of Hormonal Function Introduction Many of the complex autonomic mechanisms that maintain the chemical constancy and temperature of the internal environment are integrated in the hypothalamus. The hypothalamus also functions with the limbic system as a unit that regulates emotional and instinctual behavior Relation to the Pituitary Gland There are neural connections between the hypothalamus and the posterior lobe of the pituitary gland and vascular connections between the hypothalamus and the anterior lobe. Embryologically, the posterior pituitary arises as an evagination of the floor of the third ventricle. It is made up in large part of the endings of axons that arise from cell bodies in the supraoptic and paraventricular nuclei and pass to the posterior pituitary via the hypothalamohypophysial tract. Most of the supraoptic fibers end in the posterior lobe itself, whereas some of the paraventricular fibers end in the median eminence. The anterior and intermediate lobes of the pituitary arise in the embryo from the Rathke pouch, an evagination from the roof of the pharynx. Sympathetic nerve fiber. reach the anterior lobe from its capsule, and parasympathetic fibers reach it from the petrosal nerves, but few, if any, nerve fibers pass to it from the hypothalamus. However, the portal hypophysial vessels form a direct vascular link between the hypothalamus and the anterior pituitary. Arterial twigs from the carotid arteries and circle of Willis form a network of fenestrated capillaries called the primary plexus on the ventral surface of the hypothalamus (Figure 17–1). Capillary loops also penetrate the median eminence. The capillaries drain into the sinusoidal portal hypophysial vessels that carry blood down the pituitary stalk to the capillaries of the anterior pituitary. This system begins and ends in capillaries without going through the heart and is therefore a true portal system. In birds and some mammals, including humans, there is no other anterior hypophysial arterial supply other than capsular vessels and anastomotic connections from the capillaries of the posterior pituitary. The median eminence is generally defined as the portion of the ventral hypothalamus from which the portal vessels arise. This region is outside the blood–brain barrier THIRST Decreases in ECF volume also stimulate thirst by a pathway independent of that mediating thirst in response to increased plasma osmolality (Figure 17–4). Thus, hemorrhage causes increased drinking even if there is no change in the osmolality of the plasma. The effect of ECF volume depletion on thirst is mediated in part via the renin–angiotensin system (see Chapter 38). Renin secretion is increased by hypovolemia and results in an increase in circulating angiotensin II. The angiotensin II acts on the subfornical organ, a specialized receptor area in the diencephalon (see Figure 33–7), to stimulate the neural areas concerned with thirst. Some evidences suggest that it acts on the organum vasculosum of the lamina terminalis (OVLT) as well. These areas are highly permeable and are two of the circumventricular organs located outside the blood–brain barrier (see Chapter 33). However, drugs that block the action of angiotensin II do not completely block the thirst response to hypovolemia, and it appears that the baroreceptors in the heart and blood vessels are also involved. The intake of liquids is increased during eating (prandial drinking). The increase has been called a learned or habit response, but it has not been investigated in detail. One factor is an increase in plasma osmolality that occurs as food is absorbed. Another may be an action of one or more gastrointestinal hormones on the hypothalamus When the sensation of thirst is obtunded, either by direct damage to the diencephalon or by depressed or altered states of consciousness, patients stop drinking adequate amounts of fluid. Dehydration results if appropriate measures are not instituted to maintain water balance. If the protein intake is high, the products of protein metabolism cause an osmotic diuresis, and the amounts of water required to maintain hydration are large. Most cases of hypernatremia are actually due to simple dehydration in patients with psychoses or hypothalamic disease who do not or cannot increase their water intake when their thirst mechanism is stimulated. Lesions of the anterior communicating artery can also obtund thirst because branches of this artery supply the hypothalamic areas concerned with thirst. Control of posterior pituitary secretions vasopressin & oxytocin In most mammals, the hormones secreted by the posterior pituitary gland are arginine vasopressin (AVP) and oxytocin. In hippopotami and most pigs, arginine in the vasopressin molecule is replaced by lysine to form lysine vasopressin. The posterior pituitaries of some species of pigs and marsupials contain a mixture of arginine and lysine vasopressin. The posterior lobe nano peptides with a disulfide ring at one end BIOSYNTHESIS, INTRANEURONAL TRANSPORT, & SECRETION The hormones of the posterior pituitary gland are synthesized in the cell bodies of the magnocellular neurons in the supraoptic and paraventricular nuclei and transported down the axons of these neurons to their endings in the posterior lobe, where they are secreted in response to electrical activity in the endings Oxytocin and vasopressin are typical neural hormones, that is, hormones secreted into the circulation by nerve cells. vasopressin & oxytocin in other locations Vasopressin-secreting neurons are found in the suprachiasmatic nuclei, and vasopressin and oxytocin are also found in the endings of neurons that project from the paraventricular nuclei to the brainstem and spinal cord. These neurons appear to be involved in cardiovascular control. In addition, vasopressin and oxytocin are synthesized in the gonads and the adrenal cortex, and oxytocin is present in the thymus. The functions of the peptides in these organs are unsettled Vasopressin Receptors There are at least three kinds of vasopressin receptors: V1A, V1B, and V2. All are G-protein–coupled. The V1A and V1B receptors act through phosphatidylinositol hydrolysis to increase intracellular Ca2+ concentrations. The V2 receptors act through Gs to increase cyclic adenosine monophosphate levels. Effects of Vasopressin Because one of its principal physiologic effects is the retention of water by the kidney, vasopressin is often called the antidiuretic hormone (ADH). It increases the permeability of the collecting ducts of the kidney so that water enters the hypertonic interstitium of the renal pyramids (see Chapter 37). The urine becomes concentrated and its volume decreases. The overall effect is therefore retention of water in excess of solute; consequently, the effective osmotic pressure of the body fluids is decreased. In the absence of vasopressin, the urine is hypotonic to plasma, urine volume is increased, and there is a net water loss. Consequently, the osmolality of the body fluid rises Effects of Oxytocin In humans, oxytocin acts primarily on the breasts and uterus, though it appears to be involved in luteolysis as well. A G-protein–coupled oxytocin receptor has been identified in human myometrium, and a similar or identical receptor is found in mammary tissue and the ovary. It triggers increases in intracellular Ca2+ levels. Anterior Pituitary Hormones The anterior pituitary secretes six hormones: adrenocorticotropic hormone (corticotropin, ACTH), thyroid-stimulating hormone (thyrotropin, TSH), growth hormone, follicle-stimulating hormone (FSH), luteinizing hormone (LH), and prolactin (PRL). An additional polypeptide, β-lipotropin (β-LPH), is secreted with ACTH, but its physiologic role is unknown Nature of Hypothalamic Control Anterior pituitary secretion is controlled by chemical agents carried in the portal hypophysial vessels from the hypothalamus to the pituitary. These substances used to be called releasing and inhibiting factors, but now they are commonly called hypophysiotropic hormones. The latter term seems appropriate since they are secreted into the bloodstream and act at a distance from their site of origin. Small amounts escape into the general circulation, but they are at their highest concentration in portal hypophysial blood. Hypophysiotropic Hormones There are six established hypothalamic releasing and inhibiting hormones (Figure 17–10): corticotropin-releasing hormone (CRH); thyrotropin-releasing hormone (TRH); growth hormone–releasing hormone (GRH); growth hormone–inhibiting hormone (GIH, now generally called somatostatin); luteinizing hormone–releasing hormone (LHRH, now generally known as gonadotropin-releasing hormone [GnRH]); and prolactin-inhibiting hormone (PIH). In addition, hypothalamic extracts contain prolactin-releasing activity, and a prolactin-releasing hormone (PRH) has been postulated to exist. TRH, VIP, and several other Receptors for most of the hypophysiotropic hormones are coupled to G-proteins. There are two human CRH receptors: hCRH-RI and hCRH-RII. The physiologic role of hCRH-RII is unsettled, though it is found in many parts of the brain. In addition, a CRH-binding protein in the peripheral circulation inactivates CRH. It is also found in the cytoplasm of corticotropes in the anterior pituitary, and in this location it might play a role in receptor internalization. However, the exact physiologic role of this protein is unknown. Other hypophysiotropic hormones do not have known binding proteins Chapter 18 The Pituitary Gland Introduction The pituitary gland, or hypophysis, lies in a pocket of the sphenoid bone at the base of the brain. It is a coordinating center for control of many downstream endocrine glands, some of which are discussed in subsequent chapters. In many ways, it can be considered to consist of at least two (and in some species, three) separate endocrine organs that contain a plethora of hormonally active substances. The anterior pituitary secretes thyroid-stimulating hormone (TSH, thyrotropin), adrenocorticotropic hormone (ACTH), luteinizing hormone (LH), follicle- stimulating hormone (FSH), prolactin, and growth hormone (see Figure 17–9), and receives almost all of its blood supply from the portal hypophysial vessels that pass initially through the median eminence, a structure immediately below the hypothalamus. This vascular arrangement positions the cells of the anterior pituitary to respond efficiently to regulatory factors released from the hypothalamus. Of the listed hormones, prolactin acts on the breast. The remaining five are, at least in part, tropichormones; that is, they stimulate secretion of hormonally active substances by other endocrine glands or, in the case of growth hormone, the liver and other tissues. The tropic hormones for some endocrine glands are discussed in the chapter on that gland: TSH ; and ACTH. However, the gonadotropins FSH and LH, along with prolactin. The posterior pituitary in mammals consists predominantly of nerves that have their cell bodies in the hypothalamus, and stores oxytocin and vasopressin in the termini of these neurons, to be released into the bloodstream. The secretion of these hormones, as well as a discussion of the overall role of the hypothalamus and median eminence in regulating both the anterior and posterior pituitary. In some species, there is also a well-developed intermediate lobe of the pituitary, whereas in humans it is rudimentary. Nevertheless, the intermediate lobe, as well as the anterior pituitary, contains hormonally active of the proopiomelanocortin (POMC) molecule that regulate skin pigmentation, among other functions (see below). To avoid redundancy, this chapter will focus predominantly on growth hormone and its role in growth and facilitating the activity of other hormones, along with a number of general considerations about the pituitary. The melanocyte-stimulating hormones (MSHs) of the intermediate lobe of the pituitary, α-MSH and β-MSH, will also be touched upon. Cell Types in the Anterior Pituitary Five types of secretory cells have been identified in the anterior pituitary by immunocytochemistry and electron microscopy. The cell types are the somatotropes, which secrete growth hormone; the lactotropes (also called mammotropes), which secrete prolactin; the corticotropes, which secrete ACTH; the thyrotropes, which secrete TSH; and the gonadotropes, which secrete FSH and LH. The characteristics of these cells are summarized in Table 18–1. Some cells may contain two or more hormones. It is also notable that the three pituitary glycoprotein hormones, FSH, LH, and TSH human chorionic gonadotropin (hCG) has α and β subunits Growth Hormone Biosynthesis & Chemistry The long arm of human chromosome 17 contains the growth hormone-hCS cluster that contains five genes: one, hGH-N, codes for the most abundant (“normal”) form of growth hormone; a second, hGH-V, codes for the variant form of growth hormone; two code for human chorionic somatomammotropin (hCS) and the fifth is probably an hCS pseudogene. Growth hormone that is secreted into the circulation by the pituitary gland consists of a complex mixture of hGH-N, peptides derived from this molecule with varying degrees of posttranslational modifications, such as glycosylation, and a splice variant of hGH-N that lacks amino acids 32–46. The physiologic significance of this complex array of hormones has yet to be fully understood, particularly since their structural similarities make it difficult to assay for each species separately. Nevertheless, there is emerging evidence that, while the various peptides share a broad range of functions, they may occasionally exert actions in opposition to one another. hGH-V and hCS, on the other hand, are primarily products of the placenta, and as a consequence are only found in appreciable quantities in the circulation during pregnancy Plasma levels, Binding, & Metabolism A portion of circulating growth hormone is bound to a plasma protein that is a large fragment of the extracellular domain of the growth hormone receptor. It appears to be produced by cleavage of receptors in humans, and its concentration is an index of the number of growth hormone receptors in the tissues. Approximately 50% of the circulating pool of growth hormone activity is in the bound form, providing a reservoir of the hormone to compensate for the wide fluctuations that occur in secretion The basal plasma growth hormone level measured by radioimmunoassay in adult humans is normally less than 3 ng/mL. This represents both the protein-bound and free forms. Growth hormone is metabolized rapidly, at least in part in the liver. The half-life of circulating growth hormone in humans is 6–20 min, and the daily growth hormone output has been calculated to be 0.2–1.0 mg/d in adults Growth Hormone Receptors The growth hormone receptor is a 620-amino-acid protein with a large extracellular portion, a transmembrane domain, and a large cytoplasmic portion. It is a member of the cytokine receptor superfamily, which is discussed in Chapter 3. Growth hormone has two domains that can bind to its receptor, and when it binds to one receptor, the second binding site attracts another, producing a homodimer (Figure 18–3). Dimerization is essential for receptor activation. Growth hormone has widespread effects in the body (see below), so even though it is not yet possible precisely to correlate intracellular and whole-body effects, it is not surprising that, like insulin, growth hormone activates many different intracellular signaling cascades (Figure 18–3). Of particular note is its activation of the JAK2–STAT pathway. JAK2 is a member of the Janus family of cytoplasmic tyrosine kinases. STATs (for signal transducers and activators of transcription) are a family of cytoplasmic transcription factors that, upon phosphorylation by JAK kinases, migrate to the nucleus where they activate various genes. JAK–STAT pathways are known also to mediate the effects of prolactin and various other growth factors Effects on Growth In young animals in which the epiphyses have not yet fused to the long bones, growth is inhibited by hypophysectomy and stimulated by growth hormone. Chondrogenesis is accelerated, and as the cartilaginous epiphysial plates widen, they lay down more bone matrix at the ends of long bones. In this way, stature is increased. Prolonged treatment of animals with growth hormone leads to gigantism. When the epiphyses are closed, linear growth is no longer possible. In this case, an overabundance of growth hormone produces the pattern of bone and soft tissue deformities known in humans as acromegaly. The sizes of most of the viscera are increased. The protein content of the body is increased, and the fat content is decreased Effect on Protein & Electrolyte Homeostasis Growth hormone is a protein anabolic hormone and produces a positive nitrogen and phosphorus balance, a rise in plasma phosphorus, and a fall in blood urea nitrogen and amino acid levels. In adults with growth hormone deficiency, recombinant human growth hormone produces an increase in lean body mass and a decrease in body fat, along with an increase in metabolic rate and a fall in plasma cholesterol. Gastrointestinal absorption of Ca2+ is increased. Na+ and K+ excretion is reduced by an action independent of the adrenal glands, probably because these electrolytes are diverted from the kidneys to the growing tissues. On the other hand, excretion of the amino acid 4-hydroxyproline is increased during this growth, reflective of the ability of growth hormone to stimulate the synthesis of soluble collagen Effects on Carbohydrate & Fat metabolism The actions of growth hormone on carbohydrate metabolism are discussed in Chapter 24. At least some forms of growth hormone are diabetogenic because they increase hepatic glucose output and exert an anti-insulin effect in muscle. Growth hormone is also ketogenic and increases circulating free fatty acid (FFA) levels. The increase in plasma FFA, which takes several hours to develop, provides a ready source of energy for the tissues during hypoglycemia, fasting, and stressful stimuli. Growth hormone does not stimulate β cells of the pancreas directly, but it increases the ability of the pancreas to respond to insulinogenic stimuli such as arginine and glucose. This is an additional way growth hormone promotes growth, since insulin has a protein anabolic effect Somatomedins The effects of growth hormone on growth, cartilage, and protein metabolism depend on an interaction between growth hormone and somatomedins, which are polypeptide growth factors secreted by the liver and other tissues. The first of these factors isolated was called sulfation factor because it stimulated the incorporation of sulfate into cartilage. However, it also stimulated collagen formation, and its name was changed to somatomedin. It then became clear that there are a variety of different somatomedins and that they are members of an increasingly large family of growth factors that affect many different tissues and organs. The principal (and in humans probably the only) circulating somatomedins are insulin-like growth factor I (IGF-I, somatomedin C) and IGF-II. These factors are closely related to insulin, except that their C chains are not separated and they have an extension of the A chain called the D domain. The hormone relaxin is also a member of this family. Humans have two related relaxin isoforms, and both resemble IGF-II humans a variant form of IGF-I lacking three amino terminal amino acid residues has been found in the brain, and there are several variant forms of human IGF-II. The mRNAs for IGF-I and IGF-II are found in the liver, other proteins to intracellular organelles. Secretion of IGF-I is independent of growth hormone before birth but is stimulated by growth hormone after birth, and it has pronounced growth- stimulating activity. Its concentration in plasma rises during childhood and peaks at the time of puberty, then declines to low levels in old age. IGF-II is largely independent of growth hormone and plays a role in the growth of the fetus before birth. In human fetuses in which it is overexpressed, several organs, especially the tongue, other muscles, kidneys, heart, and liver, develop out of proportion to the rest of the body. In adults, the gene for IGF-II is expressed only in the choroid plexus and meninges Chapter 19 Thyroid Gland Introduction The thyroid gland is one of the larger endocrine glands of the body. The gland has two primary functions. The first is to secrete the thyroid hormones, which maintain the level of metabolism in the tissues that is optimal for their normal function. Thyroid hormones stimulate O2 consumption by most of the cells in the body, help regulate lipid and carbohydrate metabolism, and thereby influence body mass and mentation. Consequences of thyroid gland dysfunction depend on the life stage at which they occur. The thyroid is not essential for life, but its absence or hypofunction during fetal and neonatal life results in severe mental retardation and dwarfism. In adults, hypothyroidism is accompanied by mental and physical slowing and poor resistance to cold. Conversely, excess thyroid secretion leads to body wasting, nervousness, tachycardia, tremor, and excess heat production. Thyroid function is controlled by the thyroid-stimulating hormone (TSH, thyrotropin) of the anterior pituitary. The secretion of this hormone is in turn increased by thyrotropin-releasing hormone (TRH) from the hypothalamus and is also subject to negative feedback control by high circulating levels of thyroid hormones acting on the anterior pituitary and the hypothalamus. The second function of the thyroid gland is to secrete calcitonin, a hormone that regulates circulating levels of calcium. FORMATION & SECRETION OF THYROID HORMONE Thyroglobulin is a glycoprotein made up of two subunits and has a molecular weight of 660 kDa. It contains 10% carbohydrate by weight. It also contains 123 tyrosine residues, but only 4–8 of these are normally incorporated into thyroid hormones. Thyroglobulin is synthesized in the thyroid cells and secreted into the colloid by exocytosis of granules. The oxidation and reaction of iodide with the secreted thyroglobulin is mediated by thyroid peroxidase, a membrane-bound enzyme found in the thyrocyte apical membrane. The thyroid hormones so produced remain part of the thyroglobulin molecule until needed. As such, colloid represents a reservoir of thyroid hormones, and humans can ingest a diet completely devoid of iodide for up to 2 months before a decline in circulating thyroid hormone levels is seen. When there is a need for thyroid hormone secretion, colloid is internalized by the thyrocytes by endocytosis, and directed toward lysosomal degradation Thyroid hormone synthesis is a multistep process. Thyroid peroxidase generates reactive iodine species that can attack thyroglobulin. The first product is monoiodotyrosine (MIT). MIT is next iodinated on the carbon 5 position to form diiodotyrosine (DIT). Two DIT molecules then undergo an oxidative condensation to form T4 with the elimination of the alanine side chain from the molecule that forms the outer ring. There are two theories of how this coupling reaction occurs. One holds that the coupling occurs with both DIT molecules attached to thyroglobulin (intramolecular coupling). The other holds that the DIT that forms the outer ring is first detached from thyroglobulin (intermolecular coupling). In either case, thyroid peroxidase is involved in coupling as well as iodination. T3 is formed by condensation of MIT with DIT. A small amount of RT3 is also formed, probably by condensation of DIT with MIT. In the normal human thyroid, the average distribution of iodinated compounds is 3% MIT, 33% DIT, 35% T4, and 7% T3. Only traces of RT3 and other components are present. The human thyroid secretes about 80 μg (103 nmol) of T4, 4 μg (7 nmol) of T3, and 2 μg (3.5 nmol) of RT3 per day TRANSPORT & METABOLISM OF THYROID HORMONES protein binding The normal total plasma T4 level in adults is approximately 8 μg/dL (103 nmol/L), and the plasma T3 level is approximately 0.15 μg/dL (2.3 nmol/L). T4 and T3 are relatively lipophilic; thus, their free forms in plasma are in equilibrium with a much larger pool of protein-bound thyroid hormones in plasma and in tissues. Free thyroid hormones are added to the circulating pool by the thyroid. It is the free thyroid hormones in plasma that are physiologically active and that feed back to inhibit pituitary secretion of TSH (Figure 19–8). The plasma proteins that bind thyroid hormones are albumin, a prealbumin called transthyretin (formerly called thyroxine-binding prealbumin), and a globulin known as thyroxine-binding globulin (TBG). Of the three proteins, albumin has the largest capacity to bind T4 (ie, it can bind the most T4 before becoming saturated) and TBG has the smallest capacity. However, the affinities of the proteins for T4 (ie, the avidity with which they bind T4 under physiologic conditions) are such that most of the circulating T4 is bound to TBG (Table 19–1), with over a third of the binding sites on the protein occupied. Smaller amounts of T4 are bound to transthyretin and albumin. The half-life of transthyretin is 2 days, that of TBG is 5 days, and that of albumin is 13 days. Metabolism of Thyroid Hormones T4 and T3 are deiodinated in the liver, the kidneys, and many other tissues. These deiodination reactions serve not only to catabolize the hormones, but also to provide a local supply specifically of T3, which is believed to be the primary mediator of the physiologic effects of thyroid secretion. One third of the circulating T4 is normally converted to T3 in adult humans, and 45% is converted to RT3. As shown in Figure 19–7, only about 13% of the circulating T3 is secreted by the thyroid while 87% is formed by deiodination of T4; similarly, only 5% of the circulating RT3 is secreted by the thyroid and 95% is formed by deiodination of T4 Regulation of Thyroid Secretion Thyroid function is regulated primarily by variations in the circulating level of pituitary TSH (Figure 19–8). TSH secretion is increased by the hypothalamic hormone TRH and inhibited in a negative feedback manner by circulating free T4 and T3. The effect of T4 is enhanced by production Effects of TSH on the Thyroid When the pituitary is removed, thyroid function is depressed and the gland atrophies; when TSH is administered, thyroid function is stimulated. Within a few minutes after the injection of TSH, there are increases in iodide binding, synthesis of T3, T4, and iodotyrosines, secretion of thyroglobulin into the colloid, and endocytosis of colloid. Iodide trapping is increased in a few hours; blood flow increases; and, with long- term TSH treatment, the cells hypertrophy and the weight of the gland increases. Whenever TSH stimulation is prolonged, the thyroid becomes detectably enlarged. Enlargement of the thyroid is called a goit Effects of Thyroid Hormones Some of the widespread effects of thyroid hormones in the body are secondary to stimulation of O2 consumption (calorigenic action), although the hormones also affect growth and development in mammals, help regulate lipid metabolism, and increase the absorption of carbohydrates from the intestine (Table 19–5). They also increase the dissociation of oxygen from hemoglobin by increasing red cell 2,3- diphosphoglycerate (DPG) Mechanism of action Thyroid hormones enter cells and T3 binds to TR in the nuclei. T4 can also bind, but not as avidly. The hormone–receptor complex then binds to DNA via zinc fingers and increases (or in some cases, decreases) the expression of a variety of different genes that code for proteins that regulate cell function. Thus, the nuclear receptors for thyroid hormones are members of the superfamily of hormone-sensitive nuclear transcription factors. Chapter 20 The Adrenal Medulla & Adrenal Cortex: There are two endocrine organs in the adrenal gland, one surrounding the other. The main secretions of the inner adrenal medulla are the catecholamines epinephrine, norepinephrine, and dopamine; the outer adrenal cortex secretes steroid hormones. The adrenal cortex secretes glucocorticoids, steroids with widespread effects on the metabolism of carbohydrate and protein; and a mineralocorticoid essential to the maintenance of Na+ balance and extracellular fluid (ECF) volume. It is also a secondary site of androgen synthesis, secreting sex hormones such as testosterone, which can exert effects on reproductive function. The adrenal medulla, which constitutes 28% of the mass of the adrenal gland, two cell types can be distinguished morphologically: an epinephrine-secreting type and a norepinephrine-secreting type. In humans, 90% of the cells are the epinephrine- secreting type and 10% are the norepinephrine-secreting type. In adult mammals, the adrenal cortex is divided into three zones ,the outer zona glomerulosa , then zona fasciculate and zona reticularis. All three cortical zones secrete corticosterone, but the active enzymatic mechanism for aldosterone biosynthesis is limited to the zona glomerulosa, whereas the enzymatic mechanisms for forming cortisol and sex hormones are found in the two inner zones. FIGURE 1: Section through an adrenal gland showing both the medulla and the zones of the cortex, as well as the hormones they secrete. ADRENAL MEDULLA: STRUCTURE & FUNCTION OF MEDULLARY HORMONES: CATECHOLAMINES Norepinephrine, epinephrine, and small amounts of dopamine are synthesized by the adrenal medulla. Norepinephrine is formed by hydroxylation and decarboxylation of tyrosine, and epinephrine by methylation of norepinephrine. In plasma, about 95% of the dopamine and 70% of the norepinephrine and epinephrine are conjugated to sulfate. Sulfate conjugates are inactive and their function is unsettled. The catecholamines have a half-life of about 2 min in the circulation. EFFECTS OF EPINEPHRINE & NOREPINEPHRINE: The norepinephrine and epinephrine exert metabolic effects that include glycogenolysis in liver and skeletal muscle, mobilization of free fatty acids (FFA), increased plasma lactate, and stimulation of the metabolic rate. The effects of norepinephrine and epinephrine are brought about by actions on two classes of receptors: α- and β-adrenergic receptors. In addition, the catecholamines increase the secretion of insulin and glucagon via β-adrenergic mechanisms and inhibit the secretion of these hormones via α-adrenergic mechanisms. Norepinephrine and epinephrine both increase the force and rate of contraction of the isolated heart. These responses are mediated by β1-receptors. Norepinephrine produces vasoconstriction in most if not all organs via α1-receptors, but epinephrine dilates the blood vessels in skeletal muscle and the liver via β2-receptors. EFFECTS OF DOPAMINE: The physiologic function of the dopamine in the circulation is unknown. However, injected dopamine produces renal vasodilation, probably by acting on a specific dopaminergic receptor. It produces vasoconstriction, probably by releasing norepinephrine, and it has a positive inotropic effect on the heart by an action on β1- adrenergic receptors. Dopamine is made in the renal cortex. It causes natriuresis and may exert this effect by inhibiting renal Na, K, ATPase. REGULATION OF ADRENAL MEDULLARY SECRETION Certain drugs act directly on the adrenal medulla, but physiologic stimuli affect medullary secretion through the nervous system. Catecholamine secretion is low in basal states, but the secretion of epinephrine and, to a lesser extent, that of norepi- nephrine is reduced even further during sleep. Increased adrenal medullary secretion is part of the diffuse sympathetic discharge provoked in emergency situations, which Cannon called the “emergency function of the sympathoadrenal system.” The ways in which this discharge prepares the individual for flight or fight and the increases in plasma catecholamines under various conditions. ADRENAL CORTEX: STRUCTURE & BIOSYNTHESIS OF ADRENOCORTICAL HORMONES CLASSIFICATION & STRUCTURE The hormones of the adrenal cortex are derivatives of cholesterol. Like cholesterol, bile acids, vitamin D, and ovarian and testicular steroids, they contain the cyclopentanoperhydrophenanthrene nucleus. SECRETED STEROIDS: Innumerable steroids have been isolated from adrenal tissue, but the only steroids normally secreted in physiologically significant amounts are the mineralocorticoid aldosterone, the glucocorticoids cortisol and corticosterone, and the androgens dehydroepiandrosterone (DHEA) and androstenedione. Deoxycorticosterone is a mineralocorticoid that is normally secreted in about the same amount as aldosterone but has only 3% of the mineralocorticoid activity of aldosterone. STEROID BIOSYNTHESIS: The precursor of all steroids is cholesterol. Some of the cholesterol is synthesized from acetate, but most of it is taken up from LDL in the circulation. Cholesterol ester hydrolase catalyzes the formation of free choles- terol in the lipid droplets. The cholesterol is transported to mitochondria by a sterol carrier protein. In the mitochondria, it is converted to pregnenolone. Pregnenolone moves to the smooth endoplasmic reticulum, where some of it is dehydrogenated to form progesterone. ACTH binds to high-affinity receptors on the plasma membrane of adrenocortical cells. This activates adenylyl cyclase via Gs. The resulting reactions lead to a prompt increase in the formation of pregnenolone and its derivatives. Over longer periods, ACTH also increases the synthesis of the P450s involved in the synthesis of glucocorticoids. TRANSPORT, METABOLISM, & EXCRETION OF ADRENOCORTICAL HORMONES: GLUCOCORTICOID BINDING Cortisol is bound in the circulation to an α globulin called transcortin or corticosteroid-binding globulin (CBG). CBG is synthesized in the liver and its production is increased by estrogen. CBG levels are elevated during pregnancy and depressed in cirrhosis, nephrosis, and multiple myeloma. When the CBG level rises, more cortisol is bound, and initially the free cortisol level drops. This stimulates ACTH secretion, and more cortisol is secreted. Changes in the opposite direction occur when the CBG level falls. A minor degree of binding to albumin also takes place. The half-life of cortisol in the circulation is therefore longer (about 60–90 min). Bound steroids are physiologically inactive. In addition, relatively little free cortisol & corticosterone are found in the urine because of protein binding. The bound cortisol functions as a circulating reservoir of hormone that keeps a supply of free cortisol available to the tissue. METABOLISM & EXCRETION OF GLUCOCORTICOIDS Cortisol is metabolized in the liver, which is the principal site of glucocorticoid catabolism. Most of the cortisol is reduced to dihydrocortisol and then to tetrahydrocortisol, which is conjugated to glucuronic acid. Cortisone is an active glucocorticoid because it is converted to cortisol, and it is well known because of its extensive use in medicine. It is not secreted in appreciable quantities by the adrenal glands. Little, if any, of the cortisone formed in the liver enters the circulation, because it is promptly reduced and conjugated to form tetrahydrocortisone glucuronides. ALDOSTERONE Aldosterone is bound to protein to only a slight extent, and its half-life is short (about 20 min). The amount secreted is small when compared with a cortisol level (bound and free). Much of the aldosterone is converted in the liver to the tetrahy- droglucuronide derivative, but some is changed in the liver and in the kidneys to an 18-glucuronide. 17-KETOSTEROIDS The major adrenal androgen is the 17-ketosteroid dehydroepiandrosterone, although androstenedione is also secreted. The 11-hydroxy derivative of androstenedione and the 17-ketosteroids formed from cortisol and cortisone by side chain cleavage in the liver. Testosterone is also converted to a 17-ketosteroid. Because the daily 17- ketosteroid excretion in normal adults is 15 mg in men and 10 mg in women, about two-thirds of the urinary ketosteroids in men are secreted by the adrenal or formed from cortisol in the liver and about one-third are of testicular origin. EFFECTS OF ADRENAL ANDROGENS & ESTROGENS ANDROGENS Androgens are the hormones that exert masculinizing effects and they promote protein anabolism and growth. Testosterone from the testes is the most active androgen and the adrenal androgens have less than 20% of its activity. Secretion of the adrenal androgens is controlled acutely by ACTH and not by gonadotropins. In normal males, so it is clear that these hormones exert very little masculinizing effect when secreted in normal amounts. However, they can produce appreciable masculinization when secreted in excessive amounts. In adult males, excess adrenal androgens merely accentuate existing. ESTROGENS The adrenal androgen androstenedione is converted to testosterone and to estrogens (aromatized) in fat and other peripheral tissues. This is an important source of estrogens in men and postmenopausal women. PHYSIOLOGIC EFFECTS OF GLUCOCORTICOIDS MECHANISM OF ACTION The multiple effects of glucocorticoids are triggered by binding to glucocorticoid receptors, and the steroid–receptor complexes act as transcription factors that promote the transcription of certain segments of DNA. EFFECTS ON INTERMEDIARY METABOLISM:.They include increased protein catabolism and increased hepatic glycogenesis and gluconeogenesis. Glucose-6-phosphatase activity is increased, and the plasma glucose level rises. Glucocorticoids exert an anti-insulin action in peripheral tissues and make diabetes worse. However, the brain and the heart are spared, so the increase in plasma glucose provides extra glucose to these vital organs. In diabetics, glucocorticoids raise plasma lipid levels and increase ketone body formation. EFFECTS ON ACTH SECRETION Glucocorticoids inhibit ACTH secretion, which represents a negative feedback response on the pituitary. EFFECTS ON THE NERVOUS SYSTEM Changes in the nervous system in adrenal insufficiency that are reversed only by glucocorticoids include the appearance of electroencephalographic waves slower than the normal β rhythm, and personality changes. The latter, which are mild, include irritability, apprehension, and inability to concentrate. EFFECTS ON WATER METABOLISM Adrenal insufficiency is characterized by an inability to excrete a water load, causing the possibility of water intoxication. Only glucocorticoids repair this deficit. In patients with adrenal insufficiency who have not received glucocorticoids, glu- cose infusion may cause high fever (“glucose fever”) followed by collapse and death. EFFECTS ON THE BLOOD CELLS & LYMPHATIC ORGANS: Glucocorticoids decrease the number of circulating eosinophils by increasing their sequestration in the spleen and lungs. Glucocorticoids also lower the number of basophils in the circulation and increase the number of neutrophils, platelets, and red blood cells. RESISTANCE TO STRESS The term stress as used in biology has been defined as any change in the environment that changes or threatens to change an existing optimal steady state. Most, if not all, of these stresses activate processes at the molecular, cellular, or systemic level that tend to restore the previous state, that is, they are homeostatic reactions. Some, but not all, of the stresses stimulate ACTH secretion. The increase in ACTH secretion is essential for survival when the stress is severe. Most of the stressful stimuli that increase ACTH secretion also activate the sympathetic nervous system, and part of the function of circulating glucocorticoids may be maintenance of vascular reactivity to catecholamines. It should also be noted that the increase in ACTH, which is beneficial in the short term, becomes harmful and disruptive in the long term, causing among other things, the abnormalities of Cushing syndrome PHARMACOLOGIC & PATHOLOGIC EFFECTS OF GLUCOCORTICOIDS CUSHING SYNDROME The clinical picture produced by prolonged increases in plasma glucocorticoids was described by Harvey Cushing and is called Cushing syndrome. Patients with Cushing syndrome are protein-depleted as a result of excess protein catabolism. The skin and subcutaneous tissues are therefore thin and the muscles are poorly devel- oped. The hair is thin and scraggly. Many patients with the disease have some increase in facial hair and acne, but this is caused by the increased secretion of adrenal androgens and often accompanies the increase in glucocorticoid secretion. Body fat is redistributed in a characteristic way. The extremities are thin, but fat collects in the abdominal wall, face, and upper back, where it produces a “buffalo hump.” The salt and water retention plus the facial obesity cause the characteristic plethoric, rounded “moon-faced” appearance, and there may be significant K+ depletion and weakness. About 85% of patients with Cushing syndrome are hypertensive. The hypertension may be due to increased deoxycorticosterone secretion, increased angiotensinogen secretion, or a direct glucocorticoid effect on blood vessels. ANTI-INFLAMMATORY & ANTI-ALLERGIC EFFECTS OF GLUCOCORTICOIDS Glucocorticoids inhibit the inflammatory response to tissue injury. The glucocorticoids also suppress manifestations of allergic disease that are due to the release of histamine from mast cells and basophils. Both of these effects require high levels of circulating glucocorticoids and cannot be produced by administering steroids without producing the other manifestations of glucocorticoid excess. REGULATION OF GLUCOCORTICOID SECRETION ROLE OF ACTH Both basal secretion of glucocorticoids and the increased secretion provoked by stress depend on ACTH from the anterior pituitary, its half-life in the circulation in humans is about 10 min. ACTH not only produces prompt increases in glucocorticoid secretion but also increases the sensitivity of the adrenal to subsequent doses of ACTH. ACTH are necessary to restore normal adrenal responses to ACTH. CIRCADIAN RHYTHM ACTH is secreted in irregular bursts throughout the day and plasma cortisol tends to rise and fall in response to these bursts. In humans, the bursts are most frequent in the early morning, and about 75% of the daily production of cortisol occurs between 4:00 AM and 10:00 AM. The bursts are least frequent in the evening. This diurnal (circadian) rhythm in ACTH secretion is present in patients with adrenal insufficiency receiving constant doses of glucocorticoids GLUCOCORTICOID FEEDBACK Free glucocorticoids inhibit ACTH secretion, and the degree of pituitary inhibition is proportional to the circulating glucocorticoid level. The inhibitory effect is exerted at both the pituitary and the hypothalamic levels. A drop in resting corticoid levels stimulates ACTH secretion, and in chronic adrenal insufficiency the rate of ACTH synthesis and secretion is markedly increased. EFFECTS OF MINERALOCORTICOIDS: ACTIONS Aldosterone and other steroids with mineralocorticoid activity increase the reabsorption of Na+ from the urine, sweat, saliva, and the contents of the colon. Thus, mineralocorticoids cause retention of Na+ in the ECF. This expands ECF volume. Under the influence of aldosterone, increased amounts of Na+ are in effect exchanged for K+ and H+ in the renal tubules, producing a K+ diuresis and an increase in urine acidity. MECHANISM OF ACTION Like many other steroids, aldosterone binds to a cytoplasmic receptor, and the receptor–hormone complex moves to the nucleus where it alters the transcription of mRNAs. The aldosterone-stimulated proteins have two effects—a rapid effect, to increase the activity of epithelial sodium channels (ENaCs) by increasing the insertion of these channels into the cell membrane from a cytoplasmic pool; and a slower effect to increase the synthesis of ENaCs. REGULATION OF ALDOSTERONE SECRETION STIMULI The principal conditions that increase aldosterone secretion are summarized in Table 20–6. Some of them also increase glucocorticoid secretion; others selectively affect the output of aldosterone. The primary regulatory factors involved are ACTH from the pituitary, renin from the kidney via angiotensin II, and a direct stimulatory effect on the adrenal cortex of a rise in plasma K+ concentration. EFFECT OF ACTH When first administered, ACTH stimulates the output of aldosterone as well as that of glucocorticoids and sex hormones. Although the amount of ACTH required to increase aldosterone output is somewhat greater than the amount that stimulates maximal glucocorticoid secretion. EFFECTS OF ANGIOTENSIN II & RENIN The angiotensin II is formed in the body from angiotensin I, which is liberated by the action of renin on circulating angiotensinogen. Injections of angiotensin II stimulate adrenocortical secretion and, in small doses, affect primarily the secretion of aldosterone. Hemorrhage stimulates ACTH and renin secretion. Like hemorrhage, standing and constriction of the thoracic inferior vena cava decrease intrarenal arterial pressure. Dietary sodium restriction also increases aldosterone secretion via the renin–angiotensin system.Such restriction reduces ECF volume, but aldosterone and renin secretion are increased before any consistent decrease in blood pressure takes place. ROLE OF MINERALOCORTICOIDS IN THE REGULATION OF SALT BALANCE Variations in aldosterone secretion is only one of many factors affecting Na+ excretion. Other major factors include the glomerular filtration rate, ANP, the presence or absence of osmotic diuresis, and changes in tubular reabsorption of Na+ independent of aldosterone. It takes some time for aldosterone to act. When one rises from the supine to the standing position, aldosterone secretion increases and Na+ is retained from the urine. However, the decrease in Na+ excretion develops too rapidly to be explained solely by increased aldosterone secretion. The primary function of the aldosterone-secreting mechanism is the defense of intravascular volume, but it is only one of the homeostatic mechanisms involved in this regulation. Chapter 21 Hormonal Control of Calcium & Phosphate Metabolism & the Physiology of Bone Introduction Calcium is an essential intracellular signaling molecule and also plays a variety of extracellular functions, thus the control of body calcium concentrations is vitally important. The components of the system that maintains calcium homeostasis include cell types that sense changes in extracellular calcium and release calcium- regulating hormones, and the targets of these hormones, including the kidneys, bones, and intestine, that respond with changes in calcium mobilization, excretion, or uptake. Three hormones are primarily concerned with the regulation of calcium homeostasis. Parathyroid hormone (PTH) is secreted by the parathyroid glands. Its main action is to mobilize calcium from bone and increase urinary phosphate excretion. 1,25-Dihydroxycholecalciferol is a steroid hormone formed from vitamin D by successive hydroxylations in the liver and kidneys. Its primary action is to increase calcium absorption from the intestine. Calcitonin, a calcium-lowering hormone that in mammals is secreted primarily by cells in the thyroid gland, inhibits bone resorption. Although the role of calcitonin seems to be relatively minor, all three hormones probably operate in concert to maintain the constancy of the calcium level in the body fluids. Phosphate homeostasis is likewise critical to normal body function, particularly given its inclusion in adenosine triphosphate (ATP), its role as a biological buffer, and its role as a modifier of proteins, thereby altering their functions. Many of the systems that regulate calcium homeostasis also contribute to that of phosphate, albeit sometimes in a reciprocal manner. CALCIUM & PHOSPHORUS METABOLISM Calcium The body of a young adult human contains about 1100 g (27.5 moles) of calcium. Ninety-nine percent of the calcium is in the skeleton. Plasma calcium, normally at a concentration of around 10 mg/dL (5 mEq/L, 2.5 mmol/L), is partly bound to protein and partly diffusible (Table 21–1). It is the free, ionized calcium (Ca2+) in the body fluids that is a vital second messenger and is necessary for blood coagulation, muscle contraction, and nerve function. A decrease in extracellular Ca2+ exerts a net excitatory effect on nerve and muscle cells in vivo. The result is hypocalcemic tetany, which is characterized by extensive spasms of skeletal muscle, involving especially the muscles of the extremities and the larynx. Laryngospasm can become so severe that the airway is obstructed and fatal asphyxia is produced. Ca2+ also plays an important role in blood clotting, but in vivo, fatal tetany would occur before compromising the clotting reaction. Because the extent of Ca2+ binding by plasma proteins is proportional to the plasma protein level, it is important to know the plasma protein level when evaluating the total plasma calcium. Other electrolytes and pH also affect the free Ca2+ level. Thus, for example, symptoms of tetany appear at higher total calcium levels if the patient hyperventilates, thereby increasing plasma pH. Plasma proteins are more ionized when the pH is high, providing more protein anions to bind with Ca2+. The calcium in bone is of two types: a readily exchangeable reservoir and a much larger pool of stable calcium that is only slowly exchangeable. Two independent but interacting homeostatic systems affect the calcium in bone. One is the system that regulates plasma Ca2+, providing for the movement of about 500 mmol of Ca2+ per day into and out of the readily exchangeable pool in the bone (Figure 21–1). The other system involves bone remodeling by the constant interplay of bone resorption and deposition. However, the Ca2+ interchange between plasma and this stable pool of bone calcium is only about 7.5 mmol/d. The overall transportation process of Ca2+ across the brush border of intestinal epithelial cells to the bloodstream is regulated by 1,25-dihydroxycholecalciferol. Plasma Ca2+ is filtered in the kidneys, but 98–99% of the filtered Ca2+ is reabsorbed. About 60% of the reabsorption occurs in the proximal tubules and the remainder in the ascending limb of the loop of Henle and the distal tubule. Distal tubular reabsorption is regulated by PTH. PHOSPHORUS Phosphate is found in ATP, cyclic adenosine monophosphate (cAMP), 2,3- diphosphoglycerate, many proteins, and other vital compounds in the body. Phosphorylation and dephosphorylation of proteins are involved in the regulation of cell function. Therefore, it is not surprising that, like calcium, phosphate metabolism is closely regulated. Total body phosphorus is 500–800 g (16.1–25.8 moles), 85– 90% of which is in the skeleton. Total plasma phosphorus is about 12 mg/dL, with two-thirds of this total in organic compounds and the remaining inorganic phosphorus (Pi) mostly in PO43–, HPO42–, and H2PO4–. The amount of phosphorus normally entering bone is about 3 mg (97 μmol)/kg/d, with an equal amount leaving via reabsorption. Pi in the plasma is filtered in the glomeruli, and 85–90% of the filtered Pi is reabsorbed. Active transport in the proximal tubule accounts for most of the reabsorption and involves two related sodium-dependent Pi cotransporters, NaPi-IIa and NaPi-IIc. NaPi-IIa is powerfully inhibited by PTH, which causes its internalization and degradation and thus a reduction in renal Pi reabsorption. Pi is absorbed in the duodenum and small intestine. Many stimuli that increase Ca2+ absorption, including 1,25-dihydroxycholecalciferol, also increase Pi absorption. VITAMIN D & THE HYDROXYCHOLECALCIFEROLS CHEMISTRY The active transport of Ca2+ and PO43- from the intestine is increased by a metabolite of vitamin D. The term “vitamin D” is used to refer to a group of closely related sterols produced by the action of ultraviolet light on certain provitamins (Figure 21–2). Vitamin D3, which is also called cholecalciferol, is produced in the skin of mammals from 7-dehydrocholesterol by the action of sunlight. The reaction involves the rapid formation of previtamin D3, which is then converted more slowly to vitamin D3. Vitamin D3 and its hydroxylated derivatives are transported in the plasma bound to a globulin, vitamin D-binding protein (DBP). Vitamin D3 is also ingested in the diet. Vitamin D3 is metabolized by enzymes that are members of the cytochrome P450 (CYP) superfamily. In the liver, vitamin D3 is converted to 25- hydroxycholecalciferol (calcidiol, 25-OHD3). The 25-hydroxycholecalciferol is converted in the cells of the proximal tubules of the kidneys to the more active metabolite 1,25-dihydroxycholecalciferol, which is also called calcitriol or 1,25- (OH)2D3. 1,25-Dihydroxycholecalciferol is also made in the placenta, in keratinocytes in the skin, and in macrophages. The normal plasma level of 25- hydroxycholecalciferol is about 30 ng/mL, and that of 1,25- dihydroxycholecalciferol is about 0.03 ng/mL (approximately 100 pmol/L). The less active metabolite 24,25-dihydroxycholecalciferol is also formed in the kidneys (Figure 21–2). In addition to increasing Ca2+ absorption from the intestine, 1,25- dihydroxycholecalciferol facilitates Ca2+ reabsorption in the kidneys (proximal tubules), increases the synthetic activity of osteoblasts, and is necessary for normal calcification of matrix. The stimulation of osteoblasts brings about a secondary increase in the activity of osteoclasts. REGULATION OF SYNTHESIS The formation of 25-hydroxycholecalciferol does not appear to be stringently regulated. However, the formation of 1,25-dihydroxycholecalciferol in the kidneys, which is catalyzed by the renal 1α-hydroxylase, is regulated in a feedback manner by plasma Ca2+ and PO43+ (Figure 21–3). When the plasma Ca2+ level is high, little 1,25-dihydroxycholecalciferol is produced, and the kidneys produce the relatively inactive metabolite 24,25-dihydroxycholecalciferol instead. This effect of Ca2+ on production of 1,25-dihydroxycholecalciferol is the mechanism that brings about adaptation of Ca2+ absorption from the intestine. Conversely, expression of 1α- hydroxylase is stimulated by PTH, and when the plasma Ca2+ level is low, PTH secretion is increased. The production of 1,25-dihydroxycholecalciferol is also increased by low plasma PO43– levels and inhibited by high plasma PO43– levels, by a direct inhibitory effect of PO43– on the 1α-hydroxylase. Additional control of 1,25- dihydroxycholecalciferol formation results from a direct negative feedback effect of the metabolite on 1α-hydroxylase, a positive feedback action on the formation of 24,25-dihydroxycholecalciferol, and a direct action on the parathyroid gland to inhibit PTH expression. THE PARATHYROID GLANDS ANATOMY Humans usually have four parathyroid glands: two embedded in the superior poles of the thyroid and two in its inferior poles (Figure 21–4). Each parathyroid gland is a richly vascularized disk, about 3 × 6 × 2 mm, containing two distinct types of cells (Figure 21–5). The abundant chief cells, which contain a prominent Golgi apparatus plus endoplasmic reticulum and secretory granules, synthesize and secrete PTH. The less abundant and larger oxyphil cells contain oxyphil granules and large numbers of mitochondria in their cytoplasm. In humans, few oxyphil cells are seen before puberty, and thereafter they increase in number with age. SYNTHESIS & METABOLISM OF PTH Human PTH is a linear polypeptide with a molecular weight of 9500 that contains 84 amino acid residues. It is synthesized as part of a larger molecule containing 115 amino acid residues (preproPTH). On entry of preproPTH into the endoplasmic reticulum, a leader sequence is removed from the amino terminal to form the 90- amino-acid polypeptide proPTH. Six additional amino acid residues are removed from the amino terminal of proPTH in the Golgi apparatus, and the 84-amino-acid polypeptide PTH is packaged in secretory granules and released as the main secretory product of the chief cells. The normal plasma level of intact PTH is 10–55 pg/mL. The half-life of PTH is approximately 10 min, and the secreted polypeptide is rapidly cleaved by the Kupfer cells in the liver into fragments that are probably biologically inactive. PTH and these fragments are then cleared by the kidneys. ACTIONS PTH acts directly on bone to increase bone resorption and mobilize Ca2+. In addition to increasing plasma Ca2+, PTH increases phosphate excretion in the urine and thereby depresses plasma phosphate levels. This phosphaturic action is due to a decrease in reabsorption of phosphate via effects on NaPi-IIa in the proximal tubules. PTH also increases reabsorption of Ca2+ in the distal tubules, although Ca2+ excretion in the urine is often increased in hyperparathyroidism because the increase in the load of filtered calcium overwhelms the effect on reabsorption. PTH also increases the formation of 1,25-dihydroxycholecalciferol, and this increases Ca2+ absorption from the intestine. On a longer time scale, PTH stimulates both osteoblasts and osteoclasts. REGULATION OF SECRETION Circulating Ca2+ acts directly on the parathyroid glands in a negative feedback manner to regulate the secretion of PTH. When the plasma Ca2+ level is high, PTH secretion is inhibited and Ca2+ is deposited in the bones. When it is low, secretion is increased and Ca2+ is mobilized from the bones. 1,25-Dihydroxycholecalciferol acts directly on the parathyroid glands to decrease preproPTH mRNA. Increased plasma phosphate stimulates PTH secretion by lowering plasma levels of free Ca2+ and inhibiting the formation of 1,25- dihydroxycholecalciferol. Magnesium is required to maintain normal parathyroid secretory responses. Impaired PTH release along with diminished target organ responses to PTH account for the hypocalcemia that occasionally occurs in magnesium deficiency. CALCITONIN ORIGIN, SECRETION & METABOLISM Calcitonin is a Ca2+-lowering hormone. In mammals, calcitonin is produced by the parafollicular cells of the thyroid gland, which are also known as the clear or C cells. Calcitonin secretion is increased when the thyroid gland is exposed to a plasma calcium level of approximately 9.5 mg/dL. Above this level, plasma calcitonin is directly proportional to plasma calcium. β-Adrenergic agonists, dopamine, and estrogens also stimulate calcitonin secretion. Gastrin, cholecystokinin (CCK), glucagon, and secretin have also been reported to stimulate calcitonin secretion, with gastrin being the most potent stimulus. Thus, the plasma calcitonin level is elevated in Zollinger–Ellison syndrome and in pernicious anemia. However, the dose of gastrin needed to stimulate calcitonin secretion is supraphysiologic and not seen after eating in normal individuals, so dietary calcium in the intestine probably does not induce secretion of a calcium-lowering hormone prior to the calcium being absorbed. In any event, the actions of calcitonin are short-lived because it has a half- life of less than 10 min in humans. ACTIONS Receptors for calcitonin are found in bones and the kidneys. Calcitonin lowers circulating calcium and phosphate levels. It exerts its calcium-lowering effect by inhibiting bone resorption. This action is direct, and calcitonin inhibits the activity of osteoclasts in vitro. It also increases Ca2+ excretion in the urine. The exact physiologic role of calcitonin is uncertain. The calcitonin content of the human thyroid is low, and after thyroidectomy, bone density and plasma Ca2+ level are normal as long as the parathyroid glands are intact. In addition, after thyroidectomy, there are only transient abnormalities of Ca2+ homeostasis when a Ca2+ load is injected. This may be explained in part by secretion of calcitonin from tissues other than the thyroid. However, there is general agreement that the hormone has little long-term effect on the plasma Ca2+ level in adult animals and humans. Further, unlike PTH and 1,25-dihydroxycholecalciferol, calcitonin does not appear to be involved in phosphate homeostasis. Moreover, patients with medullary carcinoma of the thyroid have a very high circulating calcitonin level but no symptoms directly attributable to the hormone, and their bones are essentially normal. No syndrome due to calcitonin deficiency has been described. More hormone is secreted in young individuals, and it may play a role in skeletal development. In addition, it may protect the bones of the mother from excess calcium loss during pregnancy. Bone formation in the infant and lactation are major drains on Ca2+ stores, and plasma concentrations of 1,25-dihydroxycholecalciferol are elevated in pregnancy. They would cause bone loss in the mother if bone resorption were not simultaneously inhibited by an increase in the plasma calcitonin level. SUMMARY OF CALCIUM HOMEOSTATIC MECHANISMS The actions of the three principal hormones that regulate the plasma concentration of Ca2+ can now be summarized. PTH increases plasma Ca2+ by mobilizing this ion from bone. It increases Ca2+ reabsorption in the kidney, but this may be offset by the increase in filtered Ca2+. It also increases the formation of 1,25- dihydroxycholecalciferol. 1,25-Dihydroxycholecalciferol increases Ca2+ absorption from the intestine and increases Ca2+ reabsorption in the kidneys. Calcitonin inhibits bone resorption and increases the amount of Ca2+ in the urine. EFFECTS OF OTHER HORMONES & HUMORAL AGENTS ON CALCIUM METABOLISM Calcium metabolism is affected by various hormones in addition to 1,25- dihydroxycholecalciferol, PTH, and calcitonin. Glucocorticoids lower plasma Ca2+ levels by inhibiting osteoclast formation and activity, but over long periods they cause osteoporosis by decreasing bone formation and increasing bone resorption. They decrease bone formation by inhibiting protein synthesis in osteoblasts. They also decrease the absorption of Ca2+ and PO43– from the intestine and increase the renal excretion of these ions. The decrease in plasma Ca2+ concentration also increases the secretion of PTH, and bone resorption is facilitated. Growth hormone increases Ca2+ excretion in the urine, but it also increases intestinal absorption of Ca2+, and this effect may be greater than the effect on excretion, with a resultant positive calcium balance. Insulin-like growth factor I (IGF-I) generated by the action of growth hormone stimulates protein synthesis in bone. As noted previously, thyroid hormones may cause hypercalcemia, hypercalciuria, and, in some instances, osteoporosis. Estrogens prevent osteoporosis by inhibiting the stimulatory effects of certain cytokines on osteoclasts. Insulin increases bone formation, and there is significant bone loss in untreated diabetes. BONE PHYSIOLOGY Bone is a special form of connective tissue with a collagen framework impregnated with Ca2+ and PO43– salts, particularly hydroxyapatites, which have the general formula Ca10(PO4)6(OH)2. Bone is also involved in overall Ca2+ and PO43– homeostasis. It protects vital organs, and the rigidity it provides permits locomotion and the support of loads against gravity. Old bone is constantly being resorbed and new bone formed, permitting remodeling that allows it to respond to the stresses and strains that are put upon it. It is a living tissue that is well vascularized and has a total blood flow of 200–400 mL/min in adult humans. STRUCTURE There are two types of bone: compact or cortical bone, which makes up the outer layer of most bones (Figure 21–8) and accounts for 80% of the bone in the body; and trabecular or spongy bone inside the cortical bone, which makes up the remaining 20% of bone in the body. In compact bone, the surface-to-volume ratio is low, and bone cells lie in lacunae. They receive nutrients by way of canaliculi that ramify throughout the compact bone (Figure 21–8). Trabecular bone is made up of spicules or plates, with a high surface to volume ratio and many cells sitting on the surface of the plates. Nutrients diffuse from bone extracellular fluid (ECF) into the trabeculae, but in compact bone, nutrients are provided via haversian canals (Figure 21–8), which contain blood vessels. Around each haversian canal, collagen is arranged in concentric layers, forming cylinders called osteons or haversian systems. The protein in bone matrix is over 90% type I collagen, which is also the major structural protein in tendons and skin. This collagen, which weight for weight is as strong as steel, is made up of a triple helix of three polypeptides bound tightly together. Two of these are identical α1 polypeptides encoded by one gene, and one is an α2 polypeptide encoded by a different gene. Collagens make up a family of structurally related proteins that maintain the integrity of many different organs. Over 40 collagen genes that contribute to at least 28 distinct trimeric collagens have so far been identified. BONE GROWTH During fetal development, most bones are modeled in cartilage and then transformed into bone by ossification (enchondral bone formation). The exceptions are the clavicles, the mandibles, and certain bones of the skull in which mesenchymal cells form bone directly (intramembranous bone formation). During growth, specialized areas at the ends of each long bone (epiphyses) are separated from the shaft of the bone by a plate of actively proliferating cartilage, the epiphysial plate (Figure 21–9). The bone increases in length as this plate lays down new bone on the end of the shaft. The width of the epiphysial plate is proportional to the rate of growth. The width is affected by several hormones, but most markedly by the pituitary growth hormone and IGF-I. Linear bone growth can occur as long as the epiphyses are separated from the shaft of the bone, but such growth ceases after the epiphyses unite with the shaft (epiphysial closure). The cartilage cells stop proliferating, become hypertrophic, and secrete vascular endothelial growth factor (VEGF), leading to vascularization and ossification. The epiphyses of the various bones close in an orderly temporal sequence, the last epiphyses closing after puberty. The normal age at which each of the epiphyses closes is known, and the “bone age” of a young individual can be determined by radiographing the skeleton and noting which epiphyses are open and which are closed. The periosteum is a dense fibrous, vascular, and innervated membrane that covers the surface of bones. This layer consists of an outer layer of collagenous tissue and an inner layer of fine elastic fibres that can include cells that have the potential to contribute to bone growth. The periosteum covers all surfaces of the bone except for those capped with cartilage (eg, at the joints) and serves as a site of attachment of ligaments and tendons. As one ages, the periosteum becomes thinner and loses some of its vasculature. This renders bones more susceptible to injury and disease. BONE FORMATION & RESORPTION The cells responsible for bone formation are osteoblasts and the cells responsible for bone resorption are osteoclasts. Osteoblasts are modified fibroblasts. Their early development from the mesenchyme is the same as that of fibroblasts, with extensive growth factor regulation. Later, ossification-specific transcription factors, such as runt-related transcription factor 2 (Runx2; also known as core binding factor subunit alpha-1), contribute to their differentiation. Osteoclasts, on the other hand, are members of the monocyte family. Osteoclasts erode and absorb previously formed bone. They become attached to bone via integrins in a membrane extension called the sealing zone. This creates an isolated area between the bone and a portion of the osteoclast. Proton pumps (ie, H+- dependent ATPases) then move from endosomes into the cell membrane apposed to the isolated area, and they acidify the area to approximately pH 4.0. The acidic pH dissolves hydroxyapatite, and acid proteases secreted by the cell break down collagen, forming a shallow depression in the bone (Figure 21–10). The products of digestion are then endocytosed and move across the osteoclast by transcytosis, with release into the interstitial fluid. The collagen breakdown products have pyridinoline structures, and pyridinolines can be measured in the urine as an index of the rate of bone resorption. Throughout life, bone is being constantly resorbed and new bone is being formed. The calcium in bone turns over at a rate of 100% per year in infants and 18% per year in adults. Bone remodeling is mainly a local process carried out in small areas by populations of cells called bone-remodeling units. First, osteoclasts resorb bone, and then osteoblasts lay down new bone in the same general area. This cycle takes about 100 days. Modeling drifts also occur in which the shapes of bones change as bone is resorbed in one location and added in another. Osteoclasts tunnel into cortical bone followed by osteoblasts, whereas trabecular bone remodeling occurs on the surface of the trabeculae. About 5% of the bone mass is being remodeled by about 2 million bone-remodeling units in the human skeleton at any one time. The renewal rate for bone is about 4% per year for compact bone and 20% per year for trabecular bone. The remodeling is related in part to the stresses and strains imposed on the skeleton by gravity. Chapter 22 Reproductive Development & Function of the Female Reproductive System Introduction In most species of mammals, the multiple differences between the male and the female depend primarily on a single chromosome (the Y chromosome) and a single pair of endocrine structures, namely the testes in the male and the ovaries in the female. The differentiation of the primitive gonads into testes or ovaries in utero is genetically determined in humans, but the formation of male genitalia depends on the presence of a functional, secreting testis; in the absence of testicular tissue, development is female. Evidence indicates that male sexual behavior and, in some species, the male pattern of gonadotropin secretion are due to the action of male hormones on the brain in early development. After birth, the gonads remain quiescent until adolescence, when they are activated by gonadotropins from the anterior pituitary. Hormones secreted by the gonads at this time cause the appearance of features typical of the adult male or female and the onset of the sexual cycle in the female. In human females. ovarian function regresses after a number of years and sexual cycles cease (the menopause). In males, gonadal function slowly declines with advancing age, but the ability to produce viable gametes persists. In both sexes, the gonads have a dual function: the production of germ cells (gametogenesis) and the secretion of sex hormones. The androgens are steroid sex hormones that are masculinizing in their action; the estrogens are those that are feminizing. Both types of hormones are normally secreted in both sexes. The ovaries secrete large amounts of estrogens and small amounts of androgens, a pattern that is reversed in males. Androgens are secreted from the adrenal cortex in both sexes, and some of the androgens are converted to estrogens in fat and other extragonadal and extra-adrenal tissues. The ovaries also secrete progesterone, a steroid that has special functions in preparing the uterus for pregnancy. Particularly during pregnancy, the ovaries secrete the polypeptide hormone relaxin, which loosens the ligaments of the pubic symphysis and softens the cervix, facilitating delivery of the fetus. In both sexes, the gonads secrete other polypeptides, including inhibin B, a polypeptide that inhibits follicle-stimulating hormone (FSH) secretion. The secretory and gametogenic functions of the gonads are both dependent on the secretion of the anterior pituitary gonadotropins, FSH, and luteinizing hormone (LH). The sex hormones and inhibin B feed back to inhibit gonadotropin secretion. In males, gonadotropin secretion is noncyclic; but in postpubertal females an orderly, sequential secretion of gonadotropins is necessary for the occurrence of menstruation, pregnancy, and lactation. FIGURE 22–19 Feedback regulation in female FIGURE 23–10 Feedback regulation in male SEX DIFFERENTIATION and DEVELOPMENT The Sex Chromosomes Sex is determined genetically by two chromosomes, called the sex chromosomes, to distinguish them from the somatic chromosomes (autosomes). In humans and many other mammals, the sex chromosomes are called X and Y. The Y chromosome is necessary and sufficient for the production of testes, and the testis-determining gene product is called SRY (for sex-determining region of the Y chromosome). SRY is a DNA-binding regulatory protein. It bends the DNA and acts as a transcription factor that initiates transcription of a cascade of genes necessary for testicular differentiation, including the gene for müllerian inhibiting substance (MIS). The gene for SRY is located near the tip of the short arm of the human Y chromosome. Diploid male cells contain an X and a Y chromosome (XY pattern), whereas female cells contain two X chromosomes (XX pattern). As a consequence of meiosis during gametogenesis, each normal ovum contains a single X chromosome, but half of the normal sperm contain an X chromosome and half contain a Y chromosome (Figure 22–1). When a sperm containing a Y chromosome fertilizes an ovum, an XY pattern results and the zygote develops into a genetic male. When fertilization occurs with an X-containing sperm, an XX pattern and a genetic female results. FIGURE 22–1 Basis of genetic sex determination. In the two-stage meiotic division in the female, only one cell survives as the mature ovum. In the male, the meiotic division results in the formation of four sperms, two containing the X and two the Y chromosome. Fertilization thus produces a male zygote with 22 pairs of autosomes plus an X and a Y or a female zygote with 22 pairs of autosomes and two X chromosomes. Development of the Gonads On each side of the embryo, a primitive gonad arises from the genital ridge, a condensation of tissue near the adrenal gland. The gonad develops a cortex and a medulla. Until the sixth week of development, these structures are identical in both sexes. In genetic males, the medulla develops during the seventh and eighth weeks into a testis, and the cortex regresses. Leydig and Sertoli cells appear, and testosterone and MIS are secreted. The MIS causes regression of mullerian duct. In genetic females, the cortex develops into an ovary and the medulla regresses. the müllerian duct system then develops into uterine tubes (oviducts) and a uterus. The embryonic ovary does not secrete hormones. Hormonal treatment of the mother has no effect on gonadal (as opposed to ductal and genital) differentiation in humans. PUBERTY A burst of testosterone secretion occurs in male fetuses before birth. In the neonatal period there is another burst, with unknown function, but thereafter the Leydig cells become quiescent. There follows in all mammals a period in which the gonads of both sexes are quiescent until they are activated by gonadotropins from the pituitary to bring about the final maturation of the reproductive system. (Figure 22–8). This period of final maturation is known as adolescence. It is often also called puberty, although puberty, strictly defined, is the period when the endocrine and gametogenic functions of the gonads have first developed to the point where reproduction is possible. In girls, the first event is thelarche, the development of breasts, followed by pubarche, the development of axillary and pubic hair, and then by menarche, the first menstrual period. Initial menstrual periods are generally anovulatory, and regular ovulation appears about a year later. In contrast to the situation in adulthood, removal of the gonads during the period from soon after birth to puberty causes only a small increase in gonadotropin secretion, so gonadotropin secretion is not being held in check by the gonadal hormones. In children between the ages of 7 and 10, a slow increase in estrogen and androgen secretion precedes the more rapid rise in the early teens. The age at the time of puberty is variable. puberty generally occurs between the ages of 8 and 13 in girls and 9 and 14 in boys. Another event that occurs in humans at the time of puberty is an increase in the secretion of adrenal androgens. The onset of this increase is called adrenarche. It occurs at age 8–10 years in girls and age 10–12 years in boys. (DHEA) values peak at about age 25 in women and slightly later than that in men. They then decline slowly to low values in old age. The rise appears to be due to an increase in the activity of 17α-hydroxylase. Control of the Onset of Puberty The gonads of children can be stimulated by gonadotropins; their pituitaries contain gonadotropins and their hypothalami contain gonadotropin-releasing Dehydroepiandrosterone hormone (GnRH) Thus, it seems clear that pulsatile secretion of GnRH brings on puberty. During the period from birth to puberty, a neural mechanism is operating to prevent the normal pulsatile release of GnRH. The nature of the mechanism inhibiting the GnRH pulse generator is unknown. However, one or more genes produce products that stimulate secretion of GnRH, and inhibition of these genes before puberty is an interesting possibility (Clinical Box 22–2). CLINICAL BOX 22–2 Leptin: It has been argued for some time that a critical body weight must normally be reached for puberty to occur. Thus, for example, young women who engage in strenuous athletics lose weight and stop menstruating, as do girls with anorexia nervosa. If these girls start to eat and gain weight, they menstruate again, that is, they “go back through puberty.” It now appears that leptin, the satiety-producing hormone secreted by fat cells, may be the link between body weight and puberty. Obese ob/ob mice that cannot make leptin are infertile, and their fertility is restored by injections of leptin. Leptin treatment also induces precocious puberty in immature female mice. However, the way that leptin fits into the overall control of puberty remains to be determined MENOPAUSE The human ovaries become unresponsive to gonadotropins with advancing age, and their function declines, so that sexual cycles disappear (menopause). This unresponsiveness is associated with and probably caused by decline in the number of primordial follicles, which becomes precipitous at the time of menopause. 1. The ovaries no longer secrete progesterone and 17β-estradiol in appreciable quantities, and estrogen is formed only in small amounts by aromatization of androstenedione in peripheral tissues. 2. The uterus and the vagina gradually become atrophic. 3. As the negative feedback effect of estrogens and progesterone is reduced, secretion of FSH is increased, and plasma FSH increases to high levels, LH levels are moderately high. In women, a period called perimenopause precedes menopause and can last up to 10 years. During perimenopause FSH levels will increase before an increase in LH is observed due to a decrease in estrogen, progesterone, and inhibins and the menses become irregular. This usually occurs between the ages of 45 and 55. The average age at onset of the menopause is 52 years. The loss of ovarian function causes many symptoms such as sensations of warmth spreading from the trunk to the face (hot flushes; also called hot flashes) and night sweats. In addition, the onset of menopause increases the risk of many diseases such as osteoporosis, ischemic heart disease, and renal disease. Hot flushes are said to occur in 75% of menopausal women and may continue intermittently for as long as 40 years. They also occur when early menopause is produced by bilateral ovariectomy, and they are prevented by estrogen treatment. Their cause is unknown. However, they coincide with surges of LH secretion. LH is secreted in episodic bursts at intervals of 30–60 min or more (circhoral secretion), and in the absence of gonadal hormones these bursts are large. Each hot flush begins with the start of a burst. However, LH itself is not responsible for the symptoms, because they can continue after removal of the pituitary. Instead, it appears that some estrogen- sensitive event in the hypothalamus initiates both the release of LH and the episode of flushing. Although the function of the testes tends to decline slowly with advancing age, the evidence is unclear whether there is a “male menopause” (andropause) similar to that occurring in women. THE FEMALE REPRODUCTIVE SYSTEM THE MENSTRUAL CYCLE The reproductive system of women, unlike that of men, shows regular cyclic changes that teleologically may be regarded as periodic preparations for fertilization and pregnancy. In humans and other primates, the cycle is a menstrual cycle, and its most conspicuous feature is the periodic vaginal bleeding that occurs with the shedding of the uterine mucosa (menstruation). The length of the cycle is notoriously variable in women, but an average figure is 28 days from the start of one menstrual period to the start of the next. By common usage, the days of the cycle are identified by number, starting with the first day of menstruation. Ovarian Cycle 1.The follicular phase : From the time of birth, there are many primordial follicles under the ovarian capsule. Each contains an immature ovum. At the start of each cycle, several of these follicles enlarge, and a cavity forms around the ovum (antrum formation). This cavity is filled with follicular fluid. grow rapidly on about the 6th day and becomes the dominant follicle, while the others regress, forming atretic follicles. The atretic process involves apoptosis. It is uncertain how one follicle is selected to be t