Respiratory Mechanics: Lung Capacities & Volumes PDF
Document Details
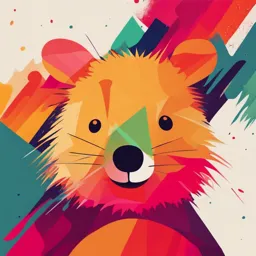
Uploaded by InstructiveTheme
BAU Medical School
Mehmet Ozansoy, Ph.D.
Tags
Summary
This document provides an overview of respiratory mechanics, focusing on lung capacities and volumes. It explains the physical properties of the lungs, including compliance, elasticity, and surface tension. It also details the different pressures involved in breathing, including intrapleural pressure, alveolar pressure, and transpulmonary pressure. The document further describes various respiratory functions, conditions, and associated measurements.
Full Transcript
RESPIRATORY MECHANICS LUNG CAPACITIES & VOLUMES Mehmet OZANSOY, Ph.D. Dept. of Physiology MECHANICS OF RESPIRATION The lungs and the chest wall are elastic structures. Normally, no more than a thin layer of fluid is present between the lungs and the chest wall (intrapleural space) The pres...
RESPIRATORY MECHANICS LUNG CAPACITIES & VOLUMES Mehmet OZANSOY, Ph.D. Dept. of Physiology MECHANICS OF RESPIRATION The lungs and the chest wall are elastic structures. Normally, no more than a thin layer of fluid is present between the lungs and the chest wall (intrapleural space) The pressure in the "space" between the lungs and chest wall (intrapleural pressure) is subatmospheric Pulmonary ventilation consists of two phases: inspiration and expiration. Inspiration (inhalation) and expiration (exhalation) are accomplished by alternately increasing and decreasing the volumes of the thorax and lungs. The lungs can be expanded and contracted in two ways: by downward or upward movement of the diaphragm to lengthen or shorten the chest cavity by elevation or depression of the ribs to increase or decrease the anteroposterior and lateral diameter of the chest cavity. Intrapleural pressure Alveolar pressure Transpulmonary pressure The movement of air into and out of the lungs occurs as a result of pressure differences induced by changes in lung volumes. Physical Properties of the Lungs Ventilation is influenced by the physical properties of the lungs, including their compliance, elasticity, and surface tension. In order for inspiration to occur, the lungs must be able to expand when stretched; they must have high compliance (ability to expand like a balloon) For expiration to occur, the lungs must get smaller when this tension is released: they must have elasticity (the tendency of a structure to return to its initial size after being distended.) The tendency to get smaller is also aided by surface tension forces exerted by fluid in the alveoli. When water forms a surface with air, the water molecules on the surface of the water have an especially strong attraction for one another. As a result, the water surface is always attempting to contract. This tension causes the alveoli to try to collapse. Surfactant • Surfactant is a surface-active agent in water, which means that it greatly reduces the surface tension of water. • It is secreted by special surfactant-secreting epithelial cells. Surfactant is a complex mixture of several phospholipids, proteins, and ions. • Surfactant begins to be produced in late fetal life. Surfactant is a surface active agent in water, which means that it greatly reduces the surface tension of water. It is secreted by special surfactant-secreting epithelial cells called type II alveolar epithelial cells, which constitute about 10 per cent of the surface area of the alveoli. These cells are granular, containing lipid inclusions that are secreted in the surfactant into the alveoli Surfactant is a complex mixture of several phospholipids, proteins, and ions. The most important components are the phospholipid Dipalmitoylphosphatidylcholine (DPPC), surfactant apoproteins, and calcium ions. The DPPC, along with several less important phospholipids, is responsible for reducing the surface tension Respiratory distress syndrome (RDS), where the alveoli are collapsed in a neonate due to lack of surfactant, occurs in 60% of babies born at less than 28 weeks of gestation. Most of these babies can be saved by the use of mechanical ventilators and by exogenous surfactant delivered into the baby’s lungs by an endotracheal tube. The lung is an elastic structure that collapses like a balloon and expels all its air through the trachea whenever there is no force to keep it inflated. Also, there are no attachments between the lung and walls of the chest cage, except where it is suspended at its hilum from the mediastinum, the middle section of the chest cavity. Instead, the lung “floats” in the thoracic cavity, surrounded by a thin layer of pleural fluid that lubricates movement of the lungs within the cavity. Pleural Pressure Pleural pressure is the pressure of the fluid (negative subatmospheric pressure – a slight suction) in the thin space between the visceral pleura and parietal pleura. The normal pleural pressure at the beginning of inspiration is negative about −5 centimeters of water (cm H2O), which is the amount of suction required to hold the lungs open to their resting level. During normal inspiration, expansion of the chest cage pulls outward on the lungs with greater force and creates more negative pressure: Intrapleural pressure decreases Then, during expiration, the events are essentially reversed: Intrapleural pressure increases (becomes less negative) Pneumothorax A pneumothorax occurs when air enters the pleural space, raising the intrapleural pressure so that the pressure difference keeping the lung against the chest wall is abolished. The lung then collapses due to its elastic recoil. Puncture from a broken rib or a trauma. Because each lung is in a separate pleural compartment, a pneumothorax usually occurs in only one lung. Intraalveolar Pressure In a closed system, the pressure of a gas and the volume of its container are inversely proportional. When the glottis is open and no air is flowing into or out of the lungs, the pressures in all parts of the respiratory tree, all the way to the alveoli, are equal to atmospheric pressure To cause inward flow of air into the alveoli during inspiration, the pressure in the alveoli must fall to a value slightly below atmospheric pressure. (subatmospheric/negative pressure) Transpulmonary Pressure The transpulmonary pressure is the pressure difference between that in the alveoli and that on the outer surfaces of the lungs (pleural pressure). Transpulmonary pressure keeps the lungs against the chest wall. During inspiration, it is the transpulmonary pressure that causes the lungs to expand as the thoracic volume expands. The transpulmonary pressure is positive during both inspiration and expiration. (During insp. increases & becomes more positive) Alveolar Ventilation The ultimate importance of pulmonary ventilation is to continually renew the air in the gas exchange areas of the lungs, where air is in proximity to the pulmonary blood. These areas include the alveoli, alveolar sacs, alveolar ducts, and respiratory bronchioles. The rate at which new air reaches these areas is called alveolar ventilation Some of the air a person breathes never reaches the gas exchange areas but simply fills respiratory passages where gas exchange does not occur, such as the nose, pharynx, and trachea. This air is called dead space air because it is not useful for gas exchange. On expiration, the air in the dead space is expired first, before any of the air from the alveoli reaches the atmosphere. Therefore, the dead space is very disadvantageous for removing the expiratory gases from the lungs Mucocilliary Escalation All the respiratory passages, from the nose to the terminal bronchioles, are kept moist by a layer of mucus that coats the entire surface. The mucus is secreted partly by individual mucous goblet cells in the epithelial lining of the passages and partly by small submucosal glands. In addition to keeping the surfaces moist, the mucus traps small particles out of the inspired air and keeps most of these from ever reaching the alveoli The entire surface of the respiratory passages, both in the nose and in the lower passages down as far as the terminal bronchioles, is lined with ciliated epithelium, with about 200 cilia on each epithelial cell. These cilia beat continually at a rate of 10 to 20 times per second. The direction of their “power stroke” is always toward the pharynx. That is, the cilia in the lungs beat upward, whereas those in the nose beat downward. This continual beating causes the coat of mucus to flow slowly, at a velocity of a few millimeters per minute, toward the pharynx. Then the mucus and its entrapped particles are either swallowed or coughed to the exterior PULMONARY VOLUMES & PULMONARY CAPACITIES Pulmonary Volumes Tidal volume is the volume of air inspired or expired with each normal breath; it amounts to about 500 ml in the average healthy man. Inspiratory reserve volume is the extra volume of air that can be inspired over and above the normal tidal volume when the person inspires with full Force (≈ 3000 ml) Expiratory reserve volume is the maximum extra volume of air that can be expired by forceful expiration after the end of a normal tidal expiration. (≈ 1100 ml) Residual volume is the volume of air remaining in the lungs even after the most forceful expiration. (≈ 1200 ml) (It remains in the lungs because the alveoli and bronchioles normally do not collapse and the larger airways are noncollapsible). Respiratory rate (number of breaths per minute) = 12-16 /min Tidal Volume = 500 ml Total minute volume (minute ventilation) Minute ventilation (mL/min) = Tidal volume (mL/breath) x Respiratory rate (breaths/min) = 12x500 = 6000ml The conducting airways have a volume of about 150mL. Because these airways do not permit gas exchange with the blood, the space within them is called the anatomical dead space. Thus, the volume of fresh air entering the alveoli during each inspiration equals the tidal volume minus the volume of air in the anatomical dead space. ~ 350ml Pulmonary Capacities Inspiratory capacity is the amount of air (≈3500 ml) that a person can breathe in, after a normal expiration and distending the lungs to the maximum amount. Functional residual capacity is the amount of air that remains in the lungs at the end of the normal expiration (≈2300 ml). Vital capacity is the maximum amount of air a person can expel from the lungs after first filling the lungs to their maximum extent and then expiring to the maximum extent (≈4600 ml). «The maximum amount of air that can be forcefully exhaled after a maximum inhalation» Total lung capacity is the maximum volume to which the lungs can be expanded with the greatest possible effort (≈5800 ml); it is equal to the vital capacity plus the residual volume. The vital capacity (VC) is an important measurement when assessing pulmonary function. A variant on this measurement is the forced expiratory volume in 1 sec (FEV 1 ), in which the person takes a maximal inspiration and then exhales maximally as fast as possible. The important value is the fraction of the total “forced” vital capacity expired in 1 sec (forced expiratory volume in 1 sec FEV1). Healthy individuals can expire approximately 80% of the vital capacity in 1 sec. Measurement of vital capacity and FEV 1 are useful diagnostically and are known as pulmonary function tests. RECORDING CHANGES IN PULMONARY VOLUME—SPIROMETRY • Pulmonary function may be assessed clinically by means of a technique known as spirometry. •A record of the breathing - spirogram RESPIRATION PROBLEMS On the basis of pulmonary function tests, lung disorders can be classified as restrictive or obstructive. Restrictive disorders Pulmonary fibrosis → lung tissue is damaged – fibrous tissue Volume & capacities (vital capacity) is reduced to below normal. FEV1 is normal. Obstructive disorders Asthma, emphysema, chronic bronchitis. → Increased airway resistant (bronchoconstriction & obstruction by mucus) Vital capacity is usually normal. FEV1 is less than normal. Measurement of Maximum Expiratory Flow When a person expires with great force, the expiratory airflow reaches a maximum flow beyond which the flow cannot be increased any more even with greatly increased additional force. Constricted lung diseases include fibrotic diseases of the lung itself, such as tuberculosis and silicosis and diseases that constrict the chest cage, such as kyphosis, scoliosis, and fibrotic pleurisy. In diseases with airway obstruction, it is usually much more difficult to expire than to inspire because the closing tendency of the airways is greatly increased by the extra positive pressure required in the chest to cause expiration. Over a period of months or years, this effect increases both the TLC and the RV The classic disease that causes severe airway obstruction is asthma. Forced Expiratory Vital Capacity (FVC) In performing the FVC maneuver, the person first inspires maximally to the total lung capacity, then exhales into the spirometer with maximum expiratory effort as rapidly and as completely as possible. The total distance of the downslope of the lung volume record represents the FVC