Chapter 14 DNA Structure, Replication and Organization PDF
Document Details
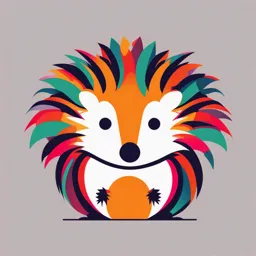
Uploaded by StaunchConnemara4071
null
Peter J. Russell, Paul E. Hertz, Beverly McMillan
Tags
Related
- Lecture 1 DNA Structure, Function, Metabolism and Replication v1 PDF
- DNA and the Molecular Structure of Chromosomes PDF
- Lecture 2.1 Molecular and Cellular Biology (DNA Replication) PDF
- FLS L1 DNA Structure and Replication 2024
- Human Genetics and Molecular Biology Notes PDF
- DNA Structure, Replication, Transcription, and Translation PDF
Summary
This document is chapter 14 of a biology textbook, detailing DNA structure, replication, and organization, focusing on sections 3-5. It includes explanations of DNA replication mechanisms and associated enzymes. The document provides diagrams and visual aids.
Full Transcript
CHAPTER 14 DNA Structure, Replication, and...
CHAPTER 14 DNA Structure, Replication, and Organization (Sections 3-5) Peter J. Russell Paul E. Hertz Beverly McMillan www.cengage.com/biology/russell 14.3 DNA Replication Complementary base-pairing explains DNA replication: Semiconservative replication Hydrogen bonds between the two strands break The two strands unwind and separate Each strand acts as a template for synthesis of a new, complementary strand Each new double helix has one old strand, derived from the parental DNA molecule, and one new strand Semiconservative Replication Complementary base pairing in Watson and Crick’s the DNA double helix: model for DNA A pairs with T, G replication pairs with C. Direction of replication The two chains unwind and separate. Each “old” strand is a template for the addition of bases according to the Product molecules base-pairing rules. The result is two DNA are half old and Old helices that are exact half new New copies of the parental DNA molecule with one “old” strand and one “new” strand. Figure 14-7, p. 29 DNA Polymerases DNA polymerases assemble complementary polynucleotide chains from individual deoxyribonucleotides Four different deoxyribonucleoside triphosphates (one for each DNA base) are used: dATP, dGTP, dCTP, and dTTP DNA polymerase adds a nucleotide only to the 3′ end (the exposed hydroxyl group) of the newly forming nucleotide chain Antiparallel Strands A 3′–OH group is exposed at the “newest” end of a new DNA strand; the “oldest” end has an exposed 5′ triphosphate DNA polymerases only assemble nucleotide chains in the 5′→3′ direction Because DNA strands run antiparallel to each other, the template strand is “read” in the 3′→5′ direction New Template strand strand 3′ end 3′ end 5′ end 5′ end DNA polymerase 3′ end Pyrophosphate Incoming nucleotide 3′ Deoxyribonucleosid end e triphosphate Hydrolysis (dATP) provides energy for DNA chain Direction of elongation new chain reaction 2 1 growth Stepped Art 5′ end 5′ end Figure 14-10, p. 29 DNA Polymerase Structure DNA polymerases consist of several polypeptide subunits arranged in different “hand-shaped” domains Template DNA lies over the “palm” in a groove formed by the “fingers” and “thumb” The template strand and the 3′–OH of the new strand meet at the active site for the polymerization reaction of DNA synthesis, located in the palm domain DNA Polymerase “Hand” Model Polymerizatio Template Pal n reaction base m active site Template strand New DNA strand Incoming Finger nucleotid s Thum e Figure 14-11a, p. 29 Sliding DNA Clamp DNA polymerase extends the new DNA strand, one nucleotide at a time, by moving along the template Sliding DNA clamp Protein that encircles DNA and attaches to the rear of DNA polymerase (relative to forward movement) “Ties” DNA polymerase to the template strand and increases rate of DNA synthesis DNA Polymerase and Sliding Clamp Template Sliding DNA strand DNA clamp polymerase New Direction DNA of DNA strand synthesis Figure 14-11b, p. 29 DNA Replication Basics 1. The two strands of the DNA molecule unwind 2. DNA polymerase adds nucleotides to an existing chain 3. Direction of new synthesis is in the 5′→3′ direction, which is antiparallel to the template strand 4. Nucleotides enter a newly synthesized chain according to the A-T and G-C complementary base- pairing rules Unwinding and Stabilizing DNA In the bacterial chromosome, unwinding of DNA for replication occurs at a small, specific region (origin of replication, ori ) DNA helicase unwinds the DNA strands, producing a Y-shaped replication fork Single-stranded binding proteins (SSBs) coat the exposed single-stranded DNA segments, keeping them from pairing Topoisomerase cuts and rejoins DNA to prevent twisting in circular bacterial chromosomes Origin of Helicase, SSBs, and Topoisomerase replication (ori) determines the start point 3′ for Replication fork replication. 5′ 3′ 5′ Direction of DNA helicase, recruited by unwinding proteins that bind to the ori, uses energy of Topoisomerase ATP hydrolysis to unwind prevents twisting the DNA as DNA unwinds strands,3′producing the replication fork. 5′ 3′ 5′ Direction of unwinding Single-stranded binding proteins (SSBs) coat and stabilize single-stranded DNA, preventing the two strands from reforming double-stranded DNA. Figure 14-12, p. 29 RNA Primers and Primase DNA polymerases add nucleotides only to an existing strand A new strand begins with a short chain of RNA (primer), synthesized by the enzyme primase Primase leaves the template, and DNA polymerase takes over, extending the RNA primer with DNA nucleotides as it synthesizes the new DNA chain RNA primers are replaced with DNA later in replication RNA Primers and Primase Primase synthesizes a short RNA primer to initiate a new3′DNA strand 5′ 5′ RNA Primase primer leaves; DNA polymerase takes over 3′ 5′ 5′ New DNA extended DNA from primer by DNA polymerase Figure 14-13, p. 29 Continuous and Discontinuous DNA Synthesis Because the two strands of a DNA molecule are antiparallel, only one template strand runs in a direction that allows DNA polymerase to make a continuous 5′→3′ copy DNA polymerase copies the other strand in short lengths, (Okazaki fragments) synthesized in the direction opposite to that of DNA unwinding (discontinuous replication) Leading and Lagging Strands Leading strand In DNA replication, the new DNA strand synthesized in the direction of DNA unwinding Synthesized on the leading strand template Lagging strand New DNA strand synthesized discontinuously, in the direction opposite DNA unwinding Synthesized on the lagging strand template Continuous and Discontinuous DNA Synthesis Leading strand template 3′ Replication fork 5′ 3′ Continuous synthesis 5′ Discontinuous 5′ 5′ 3′ 3′ 3′ synthesis 5′ 3′ Direction of DNA 5′ unwinding Lagging strand template 3′ 5′ Leading strand 5′ Lagging strand 3′ 3′ 5′ Figure 14-14, p. 29 Enzymes in DNA Replication Many enzymes coordinate to replicate DNA: DNA helicase unwinds DNA Primase initiates all new strands DNA polymerase III is the main polymerase DNA polymerase I “corrects” RNA bases on the lagging strand DNA ligase binds Okazaki fragments together DNA Replication Single-stranded binding proteins 3′ (SSBs) Replication forkTopoisomerase 5′ Primase 5′ RNA primers 5′ 3′ 3′ 5′ Direction of replication DNA helicase 1 (unwinding enzyme) DNA polymerase III Leading 3′ strand 5′ 3′ 5′ RNA DNA RNA 5′ 3′ 3′ 5′ Lagging 2 strand DNA polymerase III Figure 14-15a, p. 29 DNA Replication DNA polymerase 3′ III 5′ DNA polymerase 3′ 5′ III 5′ 3′ 3′ 5′ 3′ 5′ 3 First Primer being Second Newly Okazaki extended by Okazakisynthesized fragmen DNA fragment primer t polymerase III DNA polymerase 3′ III 5′ 3′ 5′ Nic 5′ 3′ k 3′ 5′ 4 Figure 14-15b, p. 29 DNA Replication 3′ 5 5′ 3′ 5′ DNA 5′ 3′ 3′ ligase 5′ DNA 3′ polymerase III 5′ 6 Leading DNA 3′ 5′ strand polymerase III 5′ 3′ 3′ Lagging 5′ 3′ 5′ strand Newly Primer being synthesi extended by zed DNA primer Figure 14-15c, p. 29 Major Enzymes of DNA Replication Major Enzymes of DNA Replication Major Enzymes of DNA Replication Telomeres The RNA primer in DNA replication causes a problem for replicating linear chromosomes of eukaryotes When the primer is removed it leaves a gap at the 5′ end of the new DNA strand that DNA polymerase can’t fill – causing the chromosome to shorten with each replication The ends of most eukaryotic chromosomes are protected by a buffer of noncoding DNA (the telomere) consisting of short, repeating sequences (the telomere repeat) Telomerase With each replication some telomere repeats are lost, but genes are unaffected Buffering fails when the entire telomere is lost Telomerase stops the shortening of telomeres by adding telomere repeats to chromosome ends: An RNA section binds to DNA and is the template for addition of telomere repeats Active only in rapidly dividing embryonic cells, in germ cells – and in cancerous somatic cells Telomerase Function Single-stranded region left Telomere after primer removal repeats 5′ 3′ 1 3′ 5′ Telomerase 5′ 3′ 2 3′ 5′ 3′ 5′ RNA of telo- RNA template for merase new telomere repeat gure 14-18a, p. 302 DNA Telomerase Function New DNA 5′ 3′ 3 3′ 5′ 3′ 5′ Single-stranded region left after primer removal 5′ 3′ 4 3′ 5′ 5′ Longer 5′ end of chromosome Figure 14-18b, p. 30 14.4 Mechanisms That Correct Replication Errors Errors made during replication (base-pair mismatches) are corrected in a proofreading mechanism by DNA polymerases After replication is complete, remaining base-pair mismatches are corrected by a DNA repair mechanism Proofreading Mechanism The proofreading mechanism allows DNA polymerases to back up and remove mispaired nucleotides If a newly added nucleotide is mismatched, DNA polymerase reverses, using 3′→5′ exonuclease activity to remove the fresh incorrect nucleotide DNA polymerase then resumes forward synthesis and inserts the correct nucleotide Template DNA strand polymerase 1 New strand 2 New strand 3 4 Stepped Art Figure 14-19, p. 30 DNA Repair Mechanisms DNA repair mechanisms correct base-pair mismatches that remain after proofreading (mismatch repair) Distortions in DNA structure caused by mispaired bases provide recognition sites for mismatch repair enzymes Repair enzymes cut the new DNA strand on each side of the mismatch, and remove a portion of the chain DNA polymerase fills the gap with new DNA, and DNA ligase seals the nucleotide chain Template Base-pair strand mismatch 1 New strand 2 3 Nick left after gap filled in 4 Stepped Art Figure 14-20, p. 30 Mutation and Evolutionary Processes Errors that remain in DNA after proofreading and DNA repair are a primary source of mutations (changes in DNA sequence that are passed on in replicated copies) Mutation in a gene may alter the protein encoded by the gene, which may alter how the organism functions – mutations are the ultimate source of variability acted on by natural selection 14.5 DNA Organization in Eukaryotes and Prokaryotes Enzymatic proteins catalyze every step in DNA replication Numerous other proteins organize DNA and control its function in both eukaryotes and prokaryotes Chromosomal Proteins of Eukaryotes Chromosomal proteins In eukaryotes, two major types of proteins (histone and non-histone proteins) associated with DNA structure and regulation in the nucleus Chromatin Complex of DNA and its associated proteins Structural building block of a chromosome Histones in Eukaryotic Cells Histones Small, positively-charged (basic) proteins complexed with DNA in eukaryotic chromosomes Bind to DNA by an attraction to negatively charged phosphate groups of DNA Compacts DNA in nuclei and regulates DNA activity Five types of histones exist in most eukaryotic cells: H1, H2A, H2B, H3, and H4 Histones and DNA Packing Nucleosome Two molecules each of H2A, H2B, H3, and H4 combine to form a beadlike nucleosome core particle around which DNA winds for almost two turns 10-nm chromatin fiber A DNA molecule wound into a series of nucleosomes connected by short DNA segments (linkers) like beads on a string (compacts DNA by a factor of 7) Histones and Chromatin Fibers 30-nm chromatin fiber One H1 molecule binds to both the nucleosome and the linker DNA, causing nucleosomes to pack into a coiled structure 30 nm in diameter Solenoid model One possible model for the 30-nm fiber Nucleosomes spiral helically, with about six nucleosomes per turn Levels of Organization in Eukaryotic Chromatin and Chromosomes Histone H1 binds to nucleosomes Histone tail and linker DNA, causing Histone nucleosomes to form coiled Solenoi structure. d DNA Linke Nucleosome: r DNA wound around core of molecules each of H2A, H2B, H3, H4 10-nm chromatin fiber 30-nm chromatin fiber Nucleosom Linker Chromatin Chromosom es s fiber e in metaphase Figure 14-21, p. 30 Euchromatin and Heterochromatin In order for a gene to become active, the association of DNA with histones must loosen In interphase, chromatin fibers are loosely packed in some regions (euchromatin) and densely packed in others (heterochromatin) During mitosis and meiosis, chromatin fibers fold and pack into thick, rodlike chromosomes Heterochromatin and Gene Expression Some regions of heterochromatin include genes that have been turned off and placed in a compact storage form Example: X-chromosome inactivation in mammalian females Early in development, one of the two X chromosomes is packed into a block of heterochromatin (Barr body) and inactivated Non-histone Proteins and Gene Expression Non-histone proteins All proteins associated with DNA that are not histones Many non-histone proteins help control gene expression: Some modify histones to loosen or tighten their association with DNA in chromatin Some are regulatory proteins that activate or repress the expression of a gene Some are components of enzyme-protein complexes needed for expression of a gene Prokaryotic Chromosome The single prokaryotic chromosome is simply organized, and has fewer associated proteins than those of eukaryotes Two classes of proteins have functions similar to eukaryotic histones and non-histones – - organizes DNA structurally - regulates gene activity DNA in Bacteria Bacterial chromosome Primary DNA molecule of most bacteria Usually circular with only one copy per cell Some bacteria have two or more chromosomes, and some bacterial chromosomes are linear Nucleoid In bacterial cells, an irregularly shaped mass in which the DNA circle is packed and folded DNA of the nucleoid is suspended directly in the cytoplasm, with no surrounding membrane Replication of Circular DNA Replication begins from a single origin in the DNA circle, forming two forks that travel around the circle in opposite directions Eventually, the forks meet at the opposite side from the origin to complete replication Replication of Circular DNA Origi n Replicatio n forks DNA double helix Figure 14-22, p. 30 Other Bacterial DNA and Proteins In addition to the main chromosome of the nucleoid, many bacteria contain other DNA molecules (plasmids) that are duplicated and distributed to daughter cells during cell division Certain positively-charged proteins help organize bacterial DNA into loops – providing some compaction Bacterial DNA also combines with genetic regulatory proteins with functions similar to those of non-histone proteins of eukaryotes