Separation by Absorption and Stripping PDF
Document Details
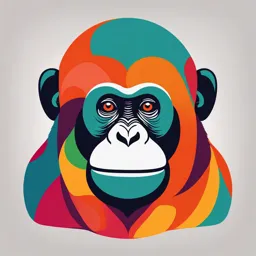
Uploaded by ManageableGalaxy
Cebu Institute of Technology - University
Tags
Related
Summary
This document discusses separation by absorption and stripping processes, which are similar to distillation but involve mass transfer between a gas phase and a liquid phase. It details the equipment used for these processes, including trayed columns, packed columns, and spray towers. It also notes the specifics of selecting an absorber.
Full Transcript
Separation by Absorption and Stripping Thickness, in. 10 103 1 III 1 1...
Separation by Absorption and Stripping Thickness, in. 10 103 1 III 1 1 1 —r i ill i— i - - - - ^ Cork _ 60 a -o Foamglass o 1(SI.= ^ Fiberglass u- O O 0 £ I02 10 >2 £ - 1 c-: - §- - o- - u - b a. a. _ c o U U All with al uminum acket (use for eq uipmenl only) J an. 20 02 10 10 -2 10-' Thickness, m Figure 15-17 Installed cost of various industrial insulations for columns, towers, and tanks SEPARATION BY ABSORPTION AND STRIPPING Absorption and stripping processes are similar to the distillation process. In the latter, the mass transfer is between the liquid and the vapor in each stage throughout the col- umn. In absorption and stripping, the mass transfer occurs between a gas phase and the liquid phase on each stage throughout the column. In absorption the solute, or compo- nent to be absorbed, is transferred from the gas phase to the liquid phase. In stripping, the reverse is true; that is, the transfer is from the liquid phase to the gas phase. Absorbers are often coupled with strippers to provide recovery of the adsorbent, as shown in Fig. 15-18. Since the stripping process is not perfect, the absorbent recovered contains components that were present in the fresh feed to the absorber. When water is used as the absorbent, it is many times more common to recover the absorbent by distillation than by stripping. The equipment used for absorption and stripping is very similar to that used for distillation except that no reboiler or condenser is required in the process. The separa- tion process is conducted with trayed columns, packed columns, as well as spray towers and centrifugal contactors. The column internals for trayed columns preferably include sieve and valve trays. The packed towers use random and structured packing similar to the geometric configurations noted earlier in Figs. 15-3 and 15-4. In most applications the choice of the absorber is between a trayed column and a packed column. The latter is generally favored when the requirements indicate a col- umn diameter less than 0.65 m and a packed height of less than 6 m. Packed towers should also be considered if the separation process involves corrosive fluids or if there 798 chapter 15 Separation Equipment—Design and Costs Solute gas Treated gas (optional) I CW CW Recovered ; solute Feed- product exchanger Rich solvent Lean solvent Solute-rich Condensate Steam feed gas (a) (b) Figure 15-18 Gas absorber using a solvent regenerated by stripping: (a) absorber; (b) stripper is a need for a minimum pressure drop across the column, as in vacuum or near ambi- ent pressure operation. The use of a spray tower may be advantageous if the solute is very soluble in the liquid phase and only one or two stages are required for the separa- tion process. Otherwise, trayed columns, which can be designed and scaled up more easily, are normally preferred. The absorbent selected is one that should have a high solubility for the solute and have a low volatility to reduce absorbent losses and to facilitate its recovery in the stripper. If there is a choice, preference should be given to an absorbent with a low vis- cosity to achieve lower pressure drops and higher mass- and heat-transfer rates in the column. Additionally, the absorbent should be nontoxic, nonflammable, and noncorro- sive to facilitate its safe use. The most widely used absorbents are water, hydrocarbon oils, and aqueous solutions of acids and bases. The most common stripping agents are air, steam, inert gases, and hydrocarbon gases. Most absorbers generally are operated at pressures greater than ambient pressure and temperatures near ambient. This operating decision minimizes the stage require- ments and/or the absorbent flow rate, which in turn reduces the equipment volume re- quired to accommodate the gas flow. On the other hand, the operating pressure should be low and the temperature high for a stripper to reduce the stage requirements and/or the flow rate of the stripping agent. However, care must be exercised in the use of these guidelines. For example, an absorber should not be operated at a pressure and/or a Separation by Absorption and Stripping temperature that would condense the feed gas. Similarly, a stripper should not operate with pressure and temperature conditions that would vaporize the liquid feed. In design problems involving flow rates for the absorbent or the stripping agent and the number of stages required, there is always a tradeoff between the two parameters at flow rates greater than the minimum value. The next section briefly outlines an analyt- ical method that can be used to determine the minimum flow rate and the tradeoff involved for a mixture that is dilute in the solute. For this situation, the absorption or stripping process is essentially isothermal, and an energy balance can be neglected. The separation process becomes complicated with concentrated mixtures and necessitates the use of computer-aided methods. Numerous literature sources detailing the design procedures for absorption and stripping are available. ' Design Procedures for Trayed Columns Separating Dilute Solutes Initiation of the design procedures for separations by either absorption or stripping is very similar to the procedures outlined for distillation design. This includes establish- ing the objectives of the separation; identifying feed components, concentrations, and flow rates; providing operating conditions whenever these are known; selecting a suit- able solvent; and obtaining appropriate equilibrium data. The objectives of the separa- tion generally will specify the percentage of the solute in the gas stream that is to be removed in the separation process. Guidelines for selecting reasonable process condi- tions and a suitable solvent have been discussed previously. Appropriate equilibrium data can be obtained or generated with the use of the literature references cited in the distillation design procedures section. Determination of Number of Equilibrium Stages Graphical methods for deter- mining equilibrium stages become very time-consuming when more than a few stages are required or the best operating conditions are to be determined. Under such condi- tions, an algebraic method is preferred for manual calculations if an appropriate simu- lator is not available. The Kremser method for single-section cascades, as modified by Edmister,* is applicable for absorption and stripping of dilute mixtures. The fraction of a solute i absorbed is given by a^ - A,. (15-30) a;^' -1 while the fraction of solute i stripped is = solute fraction stripped (15-31) where A, and 5, are the solute absorption and stripping factors for component /, Tor example see, S. Diab and R. N. Maddox, Chem. Eng., 89(26): 38 (1982), and J. D. Seader and E. J. Henley, Separation Process Principles, J. Wiley, New York, 1998. t W. C. Edmister, AlChEJ.. 3: 165 (1957). 800 chapter 15 Separation Equipment—Design and Costs respectively, and N is the number of equilibrium stages required for the specified solute fraction either absorbed or stripped. The absorption and stripping factors at low solute concentrations are conveniently defined in terms of L and V, the entering liquid and vapor flow rates into the column, respectively, and the vapor/liquid equilibrium ratio for the solute i, as Aj = — (15-32) KiV and KjV = (15-33) Estimating Ki values in either of these two equations is a function of temperature, pres- sure, and liquid-phase composition. For dilute solute mixtures and essentially ambient pressure, a number of common expressions for Ki can be estimated from pf K, = — ideal solution and subcritical temperature (15-34c/) P yP^ pj' Kj = ——- nonideal solution and subcritical temperature (15-34/?) P Hi Kj = — solute at supercritical temperature (15-34c) P PS Kj = slightly soluble solute at subcritical temperature (15-34(i) Xi p where pf is the vapor pressure of the solute, p the pressure of the gas, y™ the activity coefficient of the liquid at infinite dilution, H the Henry's law constant, and x* the mol fraction of the solute under equilibrium conditions. Once the absorption and stripping factors are estimated, Eqs. (15-30) and (15-31) can be used to determine the fraction of the solute absorbed or stripped when the number of equilibrium stages is known. EXAMPLE 15-4 Determination of Number of Theoretical Stages Required to Strip Wastewater Containing Volatile Organic Compounds to an Acceptable Level A water recovery stripper using dry air at 150C and 103 kPa as the stripping gas is to remove 99.9+ per- cent of the benzene and elhylbenzene in a wastewater stream. The flow rates of the air and wastewater streams are 2.41 and 0.0475 nrVs, respectively. The stripping is to be done at 20oC and 103 kPa. Necessary thermodynamic data from API tabulations are listed here. Volatile organic Concentration in Vapor pressure Solubility in water at component wastewater, mg/L at 20 C, kPa 20° C, mol fraction Benzene 150 10.55 0.00041 Ethylbenzene 20 1.03 0.000035 Separation by Absorption and Stripping 801 Solution Since the solute concentration is dilute, the Kremser relation may be used and applied independently to both organic impurities. Absorption of air by the water and stripping of water by the air will be considered negligible. The K value can be computed with either Eq. (15-34/?) or Eq. (15-34J). Since solubility data are available, use the latter equation. For benzene _ rof / 10.55 _ 250 ' ~ X*p ~ 0.00041(103) _ for elhylbenzene _ 1.03 _ 285 _ _ ' 0.000035(103) The vapor and liquid rates are obtained from 2.41 V = = 0.102 kg D mol/s assuming ideal gas 22.4(273/288) & 0.0475 (103) , L = —— = 2.636 kg mol/s pi = 1000 kg/nr 18.02 The stripping factors for benzene and ethylbenzene can now be calculated individually with Eq. (15-33): KiV 250(0.102) S — — =9.67 for benzene L 2.636 285(0.102) S — — = 11.03 for ethylbenzene J L 2.636 With the aid of Eq. (15-31) the fraction stripped in each theoretical stage can be evaluated with a spreadsheet computer program, to provide the following results: Percent stripped as a function of stages required Organic stripped Stage 1 Stage 2 Stage 3 Stage 4 Benzene 90.62 99.04 99.90 99.96 Ethylbenzene 91.68 99.25 99.93 99.99 Thus, three theoretical stages should be sufficient to achieve a 99.9+ percent recovery for the two volatile organic compounds. The actual height of the tower will depend on the stage efficiency provided by the stripping column under the specified operating conditions. In addition, various other airflow rates should be investigated to make certain that flooding or weeping will not occur in the column. Determination of Stage Efl^iency and Column Height To determine the actual number of plates required for an absorption or stripping process, the number of theo- retical stages must be adjusted by a stage efficiency E0. For well-designed trays and flow rates near the capacity limit, E0 depends mainly on the physical properties of the liquid and vapor streams. Values of stage efficiency are normally predicted either by 802 chapter 15 Separation Equipment—Design and Costs comparison with performance data for industrial columns or by using empirical corre- lations or semitheoretical relationships. A widely used empirical equation, based on the observation that overall stage efficiencies depend primarily on the average molar viscosity, is given by E0 = 19.2 - 57.8 log iiL (15-35) where ixL is the average viscosity in cP. This relation unfortunately provides inade- quate results for absorbers and strippers when applied to components exhibiting wide ranges in volatility or equilibrium ratios. To minimize some of these deficiencies, a more general empirical correlation has been suggested log E0=\.597 - 0.199 log - 0.0896 log (15-36) Pl/16.02 V PL/16.02/ where K is the equilibrium ratio, ML the molecular weight of the liquid, iil the viscosity of the liquid in cP, and pL the density of the liquid in kg/m3. This correlation must also be used with caution. The use of semitheoretical models to arrive at tray efficiencies for each component on each tray and then to average these to obtain an overall stage efficiency is much more complex and requires adequate software. A number of commercial simulation programs are available for such calculations. Note that the above correlations only apply to separation devices in which discrete stages are present in the column. Thus, they should not be applied to packed towers or continuous-contact devices. In these units, the efficiency becomes part of an equip- ment and system-dependent parameter such as the HETP. The actual number of trays is given by N N*a=— (15-37) Eo where Aacl is the actual number of trays and N the theoretical stages or trays. The actual column height is given by Eq. (15-10). Evaluation of Tray Diameter Determination of the diameter of an absorber or strip- per column with trays involves the same procedural steps that were outlined earlier for trayed distillation columns. This entails calculating the vapor velocity at flooding by using Fig. 15-5 for either sieve or bubble-cap trays and a specified tray spacing. (Fig- ure 15-5 appears to be conservative for valve trays.) The actual velocity at the top and bottom of the column is assumed to be between 70 and 90 percent of the net vapor velocity at flood conditions. The net and total column areas at both locations are ob- tained with Eqs. (15-8) and (15-8fl), respectively. The larger of the top or bottom diameter is used for the entire column. Design Procedures for Packed Columns Separating Dilute Solutes Since packed columns are continuous differential contacting devices, they are best analyzed by mass-transfer considerations rather than by the equilibrium stage concept described for trayed columns. The rate of mass transfer for absorption and stripping in Separation by Absorption and Stripping 803 a packed column is normally expressed in terms of a volumetric mass-transfer coeffi- cient ka, where a represents the area of mass transfer per unit volume of packed bed. At steady state and no chemical reaction within the column, the rate of solute mass trans- fer across the gas-phase film must equal the rate across the liquid-phase film. If the sys- tem is dilute with respect to the solute, the rate of mass transfer per unit volume of packed bed may be written in terms of the mol fraction driving forces in each of the two phases or in terms of a partial-pressure driving force in the gas phase and a concentra- tion driving force in the liquid phase. Determination of the packed height of a column most commonly involves the overall gas-phase coefficient since the liquid usually has a strong affinity for the solute so that resistance to mass transfer is mostly in the gas phase. This requires obtaining the height of a transfer unit HTU and the number of transfer units NTU as defined by Eqs. (15-12) and (15-13). The approach outlined to determine the HTU for a packed column used in distillation service is also adequate for use in a packed column for absorption and stripping service. To obtain the height of packing required, the number of transfer units needs to be established, as noted in Eq. (15-11). For absorption and stripping with dilute solute mixtures, the integral in Eq. (15-13) can be eliminated. This is done by using the lin- ear equilibrium condition y* = to eliminate y* and using the linear solute material balance operating line to eliminate v. The resulting relationship after integration and the substitution of L/KV = A becomes ln{[(A - 1)/A][(yin - /Ucin)/(youl - ^in)] + 1/A} NTU = (15-38) (A — 1)/A The total height of the column is the sum of the height required for the packing and the additional height required for phase disengagement and flow distribution hardware. The generalized pressure drop correlation shown in Fig. 15-7 can also be used once a suitable pressure drop per unit height of packing between 200 and 400 Pa/m has been selected to determine the cross-sectional area of the absorber or stripper. The col- umn diameter is obtained from the cross-sectional area. Design Procedures for Packed Columns Separating Concentrated Solutes The design procedures associated with absorbing or stripping dilute solute mixtures cannot be used as solute concentrations are increased since Eq. (15-13) cannot be inte- grated analytically. There are several factors that make the separation process more complex. In absorption, for example, if there is appreciable absorption, the mass flux rate decreases from the bottom to the top of the absorber. Also, as solute is transferred from the gas to the liquid phase, there is a general heating effect with an increase in temperature down the column. Thus, isothermal operation does not exist, resulting in a continual change in vapor/liquid equilibrium and the requirement for material and energy balances over the length of the column. The most common approach to determine the height of the packed column under these circumstances is to use a computer program that will stepwise move through the column to establish the equilibrium and operating conditions. The equilibrium 804 chapter 15 Separation Equipment—Design and Costs conditions will be established with appropriate thermodynamic data or correlations. The operating conditions will be determined after convergence is attained with heat and material balances. Again, a number of commercial computer programs are available to simulate the absorption or stripping processes for such operating conditions. SEPARATION BY EXTRACTION In liquid/liquid extraction, components of a liquid are separated according to their preferential distribution in a suitable solvent. Intimate contact between the two liquid phases is made to achieve the maximum approach to equilibrium. After contacting the phases, provision is made to separate the two liquid phases. The extraction is ac- complished either with simple extractors in which one phase is dispersed in the other under countercurrent flow conditions or in mechanical agitators where dispersion is enhanced by mechanical agitation and shear. The simplest extraction system involves the solute (the material to be extracted), the solvent, and the carrier (the nonsolute portion of the feed mixture). In extraction the designer must also differentiate between the light phase, the heavy phase, the dis- persed phase, the continuous phase, and the feed, raffinate, and extract phases. An extraction system always includes at least another separation device to recover the solvent from the extract phase. For example, Fig. 15-19 shows an industrial extrac- tion system where the trayed extraction column is provided with reflux in the form of extract product from the distillation column to reduce the equilibrium limitations placed on the purity of the extracted material. Note that this system utilizes a low-boiling solvent that is recovered as the distillate bottoms from the distillation column. Equipment Selection for Liquid/Liquid Extraction Because of the wide diversity of applications utilizing liquid/liquid extraction, a wide variety of separation devices have been developed. Some equipment is similar to that Raffinate Makeup solvent Condenser Extract product Feed Distillation column Extraction column Interchanger Reboiler Figure 15-19 Industrial extraction system Separation by Extraction 805 Table 15-8 General guidelines affecting extraction equipment selection Equipment class Advantages Limitations Continuous counterflow Low capital cost, low operating Can exhibit low efficiency, limited contactors, no mechanical costs, simple construction capacity with small density differ- agitation ences, flow rate restrictions, poor scale-up Continuous counterflow Medium capital cost, good Limited capacity with small density contactors, with mechanical dispersion, capability of many differences, flow rate restrictions, agitation stages, easy scale-up inadequate for emulsifying systems Mixed-settlers Good contacting, high efficiency, High capital cost, high operating capability of many stages, wide costs, large liquid holdup, may re- flow rates, good scale-up quire interstage pumping and storage Centrifugal extractors Low holdup volume, short holdup High capital cost, high operating time, small solvent requirement, costs, high maintenance costs, lim- handle low-density differences ited number of stages per unit between phases used for distillation, absorption, and stripping. However, such devices can be ineffi- cient unless the liquid viscosities are low and the differences in phase density are high. For this reason, mechanically agitated devices are often better choices. In either case, the design engineer needs to evaluate the number of theoretical stages that will be re- quired for the separation and to determine the dimensions of the equipment selected for the extraction. Extractors normally are grouped into four different classes: (1) continuous coun- terflow contactors, (2) continuous counterflow contactors with mechanical agitation, (3) mixer-settlers, and (4) centrifugal extractors. Spray, packed, and trayed columns are utilized as continuous counterflow contactors. Examples of extraction equipment that provide external mechanical agitation to increase the dispersion between liquids include such devices as the Scheibel and Oldshue-Rushton columns, the rotating-disk contactor (RDC), the asymmetric rotating-disk (ARD) contactor, the Kuhni column, the Karr reciprocating-plate column, and the Graesner extractor. The Lurgi column is an example of the mixer-settler extractors, and the Podbielniak extractor is one of several industrial centrifugal extractors. With such an array of extraction equipment available, the design engineer will need to consider the advantages and limitations associated with each class of equipment. Table 15-8 presents some general guidelines prepared by Seader and Henley ' that can provide assistance in this selection process. Reissinger and Schroeder have compared a number of industrial column-type extrac- tors for maximum liquid capacity and maximum column design. Their results are tab- ulated in Table 15-9. Liquid capacities per unit of cross-sectional area are highest with the Karr extractor and lowest with the Graesser extractor. J. D. Seader and E. J. Henley, Separation Process Principles, J. Wiley, New York, 1998. 'K, H. Reissinger and J. Schroeder, in Encyclopedia of Chemical Processing and Design, vol. 21, J. J. McKetta et al., eds.. Marcel Dekker, New York, 1984. 806 chapter 15 Separation Equipment—Design and Costs Table 15-9 Maximum loading and diameter of commercial column-type extractors Commercial Approx. maximum liquid column type loading, m3/m2-h Maximum column diameter, m Lurgi 30 8.0 Pulsed packed 40 3.0 Pulsed sieve tray 60 3.0 Scheibel 40 3.0 RDC 40 8.0 ARD 25 5.0 Khuni 50 3.0 Karr 100 1.5 Graesser 200oC No No No No Yes Adsorbate concentration in feed, 10% No Yes Yes Yes No High product purity required Yes Yes Yes Possible8 Yes Thermal regeneration required Yes Yes No No Unlikely Difficult purge/adsorbate separation Possible11 Unlikely Unlikely NA Possible1" 'Based on the information provided by G. E. Keller, II, R. A. Anderson, and C. M. Yon, in Handbook of Separation Process Technology, R. W. Rousseau, ed., J. Wiley, New York, 1987, chap. 12, p. 670, with permission. ^Includes powdered, fixed-bed and moving-bed processes. ^Requires very high adsorption pressure and low desorption pressure. iQnly if adsorbate does not need to be recovered. General Design Concepts for Separation by Adsorption A dynamic phase equilibrium is established in adsorption for the distribution of the adsorbate between the fluid and the adsorbent surface. This equilibrium is generally expressed in terms of the partial pressure or the concentration of the adsorbate in the fluid and the adsorbate loading on the adsorbent. Unfortunately, no acceptable theory has been developed to estimate adsorption equilibria. Thus, if such data are not avail- able, it will be necessary to obtain experimental equilibrium data for the specific ad- sorbate or mixture of adsorbates and the absorbent material of interest. The adsorption isotherms obtained from these experimental studies will provide an upper limit on the adsoiption of the adsorbate from a given fluid mixture on a specific adsorbent for a designated set of conditions. Experimental adsorption isotherms are classified into five types, as shown in Fig. 15-27. The type I isotherm corresponds to unimolecular adsorption, generally ap- plicable to gases at temperatures above their critical temperature. The type II isotherm is associated with multimolecular adsorption and is observed for gases at temperatures below their critical temperature and pressures below but approaching the saturation temperature. Both types I and II are desirable isotherms that exhibit strong adsorption characteristics. The type III isotherm is undesirable since its adsorption effect is low except at high pressures. The adsorption isotherms for types IV and V indicate that the multimolecular adsorption observed in types II and III is augmented by capillary con- densation. Note that a hystersis phenomenon can occur in the multimolecular adsorp- tion regions of types IV and V. Experimental adsorption data for 18 different pure gases adsorbing on various adsorbents are summarized by Valenzuela and Meyers.' Their analysis showed that t D. R Valen/uela and A. L. Meyers, Adsorption Equilibrium Data Handbook, Prentice-Hall, Englewood Cliffs, NJ. 1989. Separation by Adsorption 831 — — Relative pressure pjpo III — — -C -C — — < Relative pressure p/Po Relative pressure p//?0 V V — Do -c -P -3 1 I O E < < 0 1.0 0 1.0 Relative pressure pjp{) Relative pressure p/p^ Figure 15-27 Brunauer's five types of adsorption isotherms adsorption isotherms for these gases varied considerably with different adsorbents and that adsorbate loading varied essentially inversely with their molecular weight. For practical applications, however, the classical equations of Langmuir and Freundlich are still the best because of their simplicity and ability to correlate type I isotherms. The Langmuir relation is derived from simple mass action kinetics, assuming chemisorptions. The net rate of adsorption is the difference between the rates of adsorp- tion and desorption, given by dq' = kap(\ - co) — kjco (15-69) 832 chapter 15 Separation Equipment—Design and Costs where q' is the equilibrium loading or amount adsorbed per unit mass of adsorbent, 9 the time, p the partial pressure, ka and kj the mass-transfer coefficients of adsorption and desorption, respectively, and co the fraction of the surface covered by adsorbed molecules, defined as the ratio q'/q'max. At equilibrium, dq'/d6 = 0 and Eq. (15-69) reduces to (15-70) where K is the adsorption equilibrium constant given by the ratio ka/ kj. Solving Eq. (15-70) for the net rate of adsorption results in q' = q™xKP (15-71) ' \ + Kp At low pressures where Kp