Lecture Notes - Antibodies and Immunoglobulins PDF
Document Details
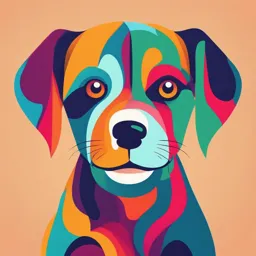
Uploaded by .keeks.
Marian University
Tags
Related
Summary
This document is a set of lecture notes on immunoglobulins and antibodies. It discusses antibody production techniques and uses of monoclonal antibodies. It explores how antibodies work to create therapeutics (drugs) and refers to the Virginia COVID antibody.
Full Transcript
All right, hopefully everything is working today. Did we have any issues with any other classes? Yes, no? There's no video. Has anybody else gotten video for their other classes? I was totally fixed to video last week when I complained because the audio wasn't working, but I guess not. So it's just...
All right, hopefully everything is working today. Did we have any issues with any other classes? Yes, no? There's no video. Has anybody else gotten video for their other classes? I was totally fixed to video last week when I complained because the audio wasn't working, but I guess not. So it's just a dark screen and I'm sorry in advance. Not that I'm gonna be doing anything exciting today, but before we do anything exciting, I'll try to make sure it works. So this is the cat, very well named because my family never decided on a real name. He's not the brightest bulb in the box, but he's got pretty good at catching rabbits. It's fitting because it's Halloween. So thank you, Ellis, for sending us your pretty black cat named The Cat. Please check your campus messages. If you have not already or communicated with me, there were some issues with people not following instructions for the in-class activity. And so to verify the authenticity of the data, also give you guys the benefit of the doubt. If you're not signed in, to draw out to know it every once in a while because it will sign you out. If every once in a while I'll sign you out, but if you can sign back in and use the same browser that you were in on, sometimes it can connect the IP address data and connect quizzes to your account, even though it's not showing up at first. So things like that. But check your messages to communicate with me and then I'll post, I gave everyone until tomorrow since I don't expect you guys to respond to me on the weekends. I just unfortunately happen to work on the weekends because it's my free time to actually catch up on everything I need to do. So by Tuesday afternoon, if I haven't heard from you, I'm gonna go ahead and post grades. So please check your messages. If I messaged you, let me know. If I have not messaged you, you're good. You didn't miss anything, please don't email me and say, hey, did you email me? No, I didn't, you're good. I don't need any more emails asking about missed emails. It would show up in your Canvas if I messaged you. There should be a little error, like number next to your inbox, whether you have messages in there or not. You guys know office hours are back to normal. Not that they were really not normal. Tuesday four to five virtual, Thursday four to five and three 16 J. This is the order we're going through in the textbook in case you have any questions about that or you wanna follow along. So today we are continuing on our antibodies and our immunoglobulins and just a cool thing like why do we care about these guys besides the fact they make up your adaptive immune system. We can use antibodies to create drugs and therapeutics. So you guys have all heard of Virginia COVID by now or knew anybody who got injected with it during COVID, very early on in the pandemic. And this is actually the science paper behind what they actually used to create this. That's where they got the name because they call it Virginia. I think they added an extra E in there now for the actual drug name. This is where they got it from and it is a neutralizing antibody, which you guys should know what neutralizing means now, but it basically prevents the virus from spreading by blocking it from infecting new cells. That's why they neutralize it here too. If you ever see the term cocktail, as you guys know, what is it? What's an alcohol cocktail or a mocktail? It's got a lot of things mixed in, right? That's what happens when we have an antibody cocktail. It's a mixture of several different antibodies. So at least it's well-named here, but that means it's a combination of monoclonal antibodies, which we will talk about today. Mono meaning one clone, but it had a couple different monoclonal antibodies to different proteins here. Our objectives for today, we will talk about how they become secreted versus transmembrane here. When we talk about a monoclonal antibody, it is produced by a clone from antibody producing cells. So we're gonna very briefly go through the immunological technique of how this is created. If you've never spent a ton of time in lab and this doesn't make sense, let me know, and I will help you with it, because sometimes it can be hard for students who have not spent time in a lab to kind of picture what's going on. So go through this as slowly as possible. Don't panic by the pictures here. This is basically describing how we could make a mouse monoclonal antibody. Now I want you guys to understand the background of this because I guarantee that at some point in your careers, you will be prescribing a monoclonal antibody to a patient. Whether it's for things like arthritis, you've heard of Humira, like that's a slightly older generation of TV commercials. There are a bunch of new ones out now, but anytime you have the word MAB at the end of a drug name, like adalimumab is Humira. If you've ever seen a drug with that, with the MAB at the end, it's talking about a monoclonal, that's where the M comes from, antibody, AB. You can guess the drug is a monoclonal antibody if you see MAB at the end. And so how we create these? We will first, we frequently use myeloma cells. If you guys have ever heard of multiple myeloma, it is a cancer of plasma cells. Now when we have myeloma cells, they can basically produce, you guys know, plasma cells produce a lot of antibodies, right? If we have these cancerous plasma cells, what is it, so cancer, in very brief, because Dr. Sittemann has a lot of detail about it, in very brief, what is the concept, like what happens with cancer? The cells are rapidly proliferating, dividing, getting a lot more cells, right? So the reason why we use myeloma cells is they're plasma cells, and we can produce a lot of them because they grow fast and they grow quickly, which means we can use them to then harvest all of the antibodies that they produce. So it's like when we've actually taken something that's pretty terrible and turn it into something good for science. So what we would first do is say we are looking for a specific target, in this case, we're looking for one of the COVID spike proteins. We can take that protein and inject it into a mouse or other small animal. It's foreign to them too, and they will mount a normal immune response, and they will start producing cells that actually secrete antibodies against whatever we inject them with. We can take that and fuse those with myeloma cells so that now these plasma cells can rapidly proliferate. Notice here, they have different colors. They have a blue, they have a yellow, and they have a green. What we're trying to do is fuse, and so they showed here that you're fusing these myeloma cells with blue, yellow, and green. Because again, you don't know, when you first sample cells from a mouse, you don't have to even inject it with something, you're gonna get all different kinds of cells. You're gonna get monocytes, you're gonna get ESNFLs, you're gonna get other things that you don't really need. And so, there's a possibility you'll fuse a lot of extra cells too, but what we want are the ones producing antibodies to that very specific antigen that we injected it with. And so we'll first fuse them, and we'll get them to grow a lot. And so we're growing all different types of cells at this point, some won't fuse well until the cells will die off because they're not viable. So clearly, we don't want those as little popcorn-looking guys here. But then we'll grow a lot of different plasma cells that can rapidly produce a lot of antibodies here. And so only these hybrids that successfully worked will continue living on here. At this point, we can then filter and sort these cells, and specifically pick these hybridoma cells. Basically, it comes from this hybrid form from the myeloma, that's where we get hybridoma form. So we go through and we then pick the specific clone that is producing antibodies to what we want. So in this case, it was the yellow plasma cell. We're gonna take that one and we're gonna catch it, and we're gonna grow that one up, divide it a lot, because it's myeloma, we can create many of these guys here. And then we basically, it'll just keep producing antibody into the supernatant or the liquid that it's growing in. We can just isolate out that liquid. And basically, we have this form where we're just rapidly producing these monoclonal antibodies in the supernatant that we can then pull out and isolate and use for any kind of drug. So we can do this, you know, like with Humira, we're looking at markers with rheumatoid arthritis. You know, if we know a specific protein involved, we see this a lot with cancer therapeutics. If we know it's a specific like estrogen receptor that's causing the cancer, we can make a target for that specific protein. So these antibodies will bind on. No idea why that goes out. So anyway, that is kind of the gist of what's going on. Does that kind of make sense for you guys? We're basically taking things that we know that they're occurring. We're taking these rapidly proliferating cells and fusing them, in this case, with rat or mice cells to create these cells that can then rapidly divide, but they're plasma cells and hybrid plasma cells producing various antibodies. And we just end up going through and selecting the specific plasma cell clone that we want. We grow those guys up and divide it a lot and just collect all the antibodies from it. So it's a really, really cool technique. If you have a chance to do it in the lab, it's really, really awesome. But it's one of the most promising forms of research we have right now, especially with personalized medicine, because in theory, we could make it to any antigen that we inject into the mice of the rats. Who in here has done Western blots? Some people, immunofluorescence or immunohistochemistry. Some of those techniques, we're using antibodies. You ever heard of an antibody cocktail where we're actually adding it to the Western blot to see what sticks and what shows up? Same way that we kind of use to produce that. It's similar to how we produce here. We can farm it by growing up a lot of these myeloma cells, fusing it, and injecting in whatever protein you wanna look for. And that's why these companies are able to make these different antibodies to then be able to sell and use. And the reason why we have to use the myeloma cells, you could, in theory, just take the plasma cells and do it. They're not gonna split as rapidly. They're not gonna produce as many antibodies, things like that, because they're gonna grow normally slowly. By fusing them and kind of immortalizing them, they're gonna live forever, proliferate a lot. We can use that so we don't have to constantly keep injecting mice and rats and constantly keep trying to get just plasma cells. By fusing them with something that's gonna divide a lot and proliferate a lot, we can kind of make this clone army down here, which we can then purpose to take the specific antibodies that we need for whatever drug treatment. So it's a really cool concept. We will refer back to this when we do our immunological techniques lecture, like right before the exam, just because a lot of the techniques use monoclonal antibodies, like for Western blots, ELISA's, immunofluorescence and immunohistochemistry. And so this is how we create those antibodies, which you then grab and use in lab. And I think the most poignant moment I've ever had in the lab when someone's like, hold your hand out, it's like one hand and put a bunch of tubes in it. They're like, you're holding $15,000 worth of stuff. And it was like five tubes, because it's so expensive. But it's a phenomenal resource that we have, that we've basically taken these scientific concepts to put them together with what we know about myeloma cells, plasma cells and antibodies to create all these fantastic drugs. When you're like, okay, cool, but Dr. D, all plasma cells really kind of look the same. How do we know one's not gonna be blue, one's not gonna be yellow, one's not gonna be green? How do we know which one we want? This is when we use flow cytometry, which is another technique. We will talk about what the immunological lectures, has anybody done flow in this class? It's pretty cool if you've ever had a chance to see it. If you don't get it, there are some pretty cool YouTube videos, and even like an online interactive fake cartoon lab, you can do it. And if you'd like to kind of practice with it, just kind of visualize it a little bit more here. But what we do with flow cytometry, we also do it, like say we wanted to tell what kind of cancer cell was proliferating in someone's blood, but it's a small lymphocyte. We don't know if it's a B or a T cell, we don't know how to treat it. We can take blood and sort via flow cytometry to figure out what cells are present here. And so all we do is we'll take a sample of cells. It can be from cell culture, it can be from someone's serum, things like that, like blood serum here. And we can go through and we can add it in, and we'll add a cocktail of antibodies. Now notice here how each of them have like a different colored label on it. All of these antibodies have been linked with what's known as a fluorophore. It's just a marker that fluoresces. And so anytime one of these antibodies binds onto its target antigen, and then it gets activated usually with like a laser or a light, in this case it's a laser, it will trigger those that are attached to glow, especially in whatever color we've tagged it in. We have a bunch of different colors that we can use, red, green, blue, hot pink. We got different choices. So we can make this cocktail with a lot of different samples and add all these antibodies in that are fluorescently labeled. And what this machine will do is it sends out this very fine stream of liquid that in theory should be sending one cell at a time past this laser. And as it goes past the laser, if it has that fluorescently tagged antibody to it, and it goes through, it will glow. And we will actually measure and track, like we tell the computer, like red means this, blue means this, like which different ones we do, we can actually send it through and figure out what cells are present in the sample. And so for here, where are T cell receptors found? Nope, nope, we're back, okay. T cell receptors, now that's your question, they're on T cells, right? Where is IgM found? It should be like bound on the membrane though, like if we're talking about immunoglobulin M B cell receptor, where is it stuck? It's not your question, B cell. This graph is showing you of this population which cells are T cells, and which cells are B cells. Because each dot on here is gonna be a different cell. So, you guys know how to read a graph. X axis, Y axis, the farther away it is, you know, more expression is there. Towards this point, which is zero, you don't see any expression, right? And so if I were to look just in this quadrant here, which is high in expressing TCR, but no BCR, what kind of cell is right here? It's a T cell, great job. And up here, you have high levels of IgM, but no levels of T cell receptor, B cell. This is an oddball, because you guys are gonna ask me, but you're like, Dr. D, it has high levels of both T cell receptor and B cell receptor, what's going on? Every once in a while, you get something known as a doublet, where two cells are stuck together, and they'll go through it, which is why I say, like we try to get single cells, but every once in a while we get a doublet, and more than likely this was a T and a B cell stuck together and went through, but you will get a handful of these oddballs here and there. So these guys here in this population that have neither a T cell receptor nor a T cell receptor nor a B cell receptor, what do you think could be in there? Anything else that's in our blood, monocytes, whatever. And we could do other labels too, we could have labeled CD16, which is found in monocytes. We have a whole list of different cell types and markers that we can use, let's say we wanted to figure out like, what's within this population, we could have stained for that too and tagged it. But that's how we use it. And even if we wanted to separate what specific antigen that we were looking for here, you could use other markers here to further break down, of these possible few cells, which one do we specifically want? Use that flow cytometry as that last step to make sure we are picking the specific plasm cell that we want for whatever target we're looking for specifically. Say we're targeting CD19, found on all B cells and it's a B cell leukemia, so we want to wipe out all B cells. In that case, again, we would look for one of the cells that was expressing CD19 and we target that in our little chart here. And so I won't really test it from this specific picture, but you will see a question on the exam with a flow cytometry graph, because again, we will cover it again for immunological techniques. That last lecture before the exam, I'm giving this to you now so you can start practicing, especially if you're a little bit more uncomfortable with lab techniques. Let me know, there are again some cute YouTube videos I can send you, some interactive things that you can play with to get a little bit more comfortable with the concepts, but you will see an easy, I'm not gonna give you a marker, you don't know, but an easy graph like this, where it's like T cell receptor, B cell receptor, which quadrant is the T cell or the B cell, you know? Be comfortable with that, yes, I'm gonna tell you this, you can pause, you can write it down, there will be a question on the exam of this, just because it is that, for some students it can be that difficult. Once you get it, it's really easy, but if you are overwhelmed by this, let me know as soon as possible so I can help you work through it, but you will see a question like this on the exam. All right, cool, so monoclonal antibodies, back to what we're doing, they are used in the treatment for a wide variety of diseases, whether they're just isolated immunoglobulins, like Regen-CoV for COVID, we can use it to target different autoimmune disorders, different cancers, things like that. I do not expect you to memorize this chart, this is just a nice appreciation of all the different drugs that we do have out there. Rituximab, that's another common one, but I forgot what the fun pharmaceutical name is. I don't know, you guys might know that one. Adelimumab is Tumira, so that's here. You've got a lot of different options here, but again, they have different targets. We know this, and we can target different things like CD19 found on all B cells, that's a specific drug here too. And those are different years, we create a lot of different targets in therapeutics over time. In multiple sclerosis alone, I think we've rolled out like 10 new monoclonal antibody treatments in the last four years. So they're rapidly expanding fields. I do not expect you to know all the different drug targets, whatever, the box here is important. Because we have a couple different monoclonal antibody types, and it affects how effective they can be, and like your risk of side effects. So some that were there fully made in mice. And so we consider those guys mouse monoclonal antibodies because we didn't use human myeloma cells. Everything here is mouse, and so everything here looks like mice. Because of that, we can see sometimes a little bit more side effects just because it does look a little foreign. So there are some considerations with that. We have something known as a chimera. Insert the joke about Teen Wolf from the chimera we talked about with CAR T cells here, but a chimera is a mixture. And so with this one, they've used the very top of the antigen binding site, that variable region is mouse, but the rest of the body is human. That way, the body recognizes it a little bit more as human. We have something known as humanized, which technically is like a chimera because technically it is a mixture of both. But these lines here are just tiny chains of genes that are mouse. So mouse is mixed in like a little bit more variably with human, that's why they call it humanized because it's mixed in a little bit more here. And then you have fully human antibodies where we've done everything in humans. And there are people who have donated cells, et cetera, to be able to do this, where we've done it in human culture. And we've been able to create a fully human monoclonal antibody. So again, I just want you to know that there are varieties over here, but I do not expect you to memorize any of these different names, targets, et cetera, in case you just have a personal curiosity with them, in which case, some people know these drug names because they've prescribed them or you can't prescribe them just yet. You've filled it in a pharmacy or you've seen someone prescribe it, things like that. So again, the overview of what we're gonna get into now, especially when we're talking about monoclonal antibodies, but like when we're talking about these immunoglobulin molecules here. You guys remember that epitope specificity of an immunoglobulin is determined before the antigen counter. Because remember, the B cell receptor is made long before it sees the bad guy. And so, because again, when they go, they leave the bone there, they travel with lymph nodes looking for the bad guy. They already know what they're looking for. It's already been created before they've even gotten to that point here. And so your number of possible epitope binding specificity is actually greater than the number of genes within the genome, which is a wild fact to think about. But how that comes across is like the paradox. How does the immune system generate this diverse array of antigen-specific molecules if we have such a limited number of genes available? Any guesses? Yes, that can be one of them, yes. Controlled mutation, any other ideas? There are actually a couple different answers. So yes, great job, that was one of them. Any other ideas? Can you say that a little loudly? Yeah, recombination is one. Mutations is another, we'll talk about that too. B cells are cool in that they have a little bit more to it. Like with the rearrangement, we'll have somatic recombination, which we will talk about. Rearrangement, isotype switching, class switching, things like that. And we'll talk about all of that a little bit today in the next class here too. And again, if you think about it, it's just mixtures of the various combinations of the variable with potentially diversity, depending on which gene chain we're on, junctional and constant. So we have a limited number of genes, but depending on also how many we mix those up, like if we do V1 with J3 versus V1 with J1. Those are different combinations, even though you have a finite number of genes total overall. This way. And so, the DNA sequence encoding a variable region is assembled from two or three gene segments, depending on what we're looking at. So first to note, we have two different types of light chains you can see. We have a lambda light chain locus, so new Greek letters, and we have a kappa light chain locus. Your heavy chain locus, technically there's one heavy chain, but it has many features of like mu, alpha, delta, epsilon, gamma, all in that one chain. And we will talk about it for right now. It doesn't show the rest of them from this heavy chain, but we will talk about that on a later slide here. We're first looking at it here, how they differ. So at the top one, we're looking at this lambda chain locus. It has about 30 functional variable lambas. So again, if you see V in front of lambda, it's the variable region of lambda. It has four pairs of functional J. So this little dot here, you guys know in math and they do dot, dot, dot, and then continue the thing. It means they're continuing on. For space reasons, they didn't add three, but there is a third chunk understood to be in this space just because it doesn't fit. It would run off the slide here. So it has one, two, three, and then technically four here. And then with each one of those junctional, it's flanked by a constant. So this J lambda one has constant lambda one. So you guys know that these two would go together. And J lambda two goes with constant lambda two. They kind of go together as chunks here. Versus when we talk about this light chain with kappa, it has about 35 variable here, but it's junctional kappa one through five are clustered together. And it only has one constant after that. So again, it would be one of these many 35 potential options, oops, with one of these junctional one through fives here, and then bound onto that one constant kappa versus here. It could be one of these variables with this junction and then this constant, or this variable with this junction and then this constant, or with the invisible still there, junctional three, constant three, or junctional four, constant four. This one is found on chromosome 22, this one is found on chromosome two, and this one is found on chromosome 14. This is important because we do have genetic disorders where there are alterations to chromosome two or 22. So say there's damage to 22 potentially. What is a consequence of that? I was gonna say it loud, I hear mumbling. Nathan, you said it slightly louder than everybody else. Yes, more than likely developmental problems or we wouldn't even be able to create lambda light chain. Versus if we had an issue on chromosome two, we would probably affect the ability to make kappa. The cool thing about this, it's not that great for the person experiencing it. However, it's again one of those body's backup mechanisms. If we knock out the ability to make at least one of the light chains, your repertoire and your diversity would be limited because you're missing a whole chunk of potential light chains that you can have, but as long as you create one of the types of light chains, you could still form a complete immunoglobulin molecule since it's a combination of that heavy with that light chain. You would just have about roughly half, granted the math's a little bit more complicated because it's like 35, five, et cetera. Slightly different in the algorithms, but you'd have roughly half of your normal breadth of repertoire as a consequence of that, but you would still have some antibodies. So you might have some immunodeficiency because you're not able to make the full breadth of immunoglobulin molecules, but you're able to make something, which is really nice. Now when we talk about your heavy chains though, they have about 40 functional heavy chains of the variable region, so you have VH, if you see VH, variable heavy. It has about a cluster of 23 diversity, and if you guys remember, it increases the diversity of the mixture because you have more possible things to mix in with instead of just having a variable, a junctional, a constant, and you now have an additional piece with the diversity that you can add on. It has about six junctional in this heavy part here, and about one through nine constants, which we will talk about because this is actually where we determine which immunoglobulin we make, depending on which constant on the heavy chain it binds onto. So it is a random recombination of these gene segments that actually gives you the full diversity of your antigen binding site for your immunoglobulin molecules here. So when we're talking about it here overall, so it's the same slide on both of these here too, it's just the first one talks about the left panel, the second slide talks about the right panel. So we're gonna go through this one here first. So we're just showing it with the light chain, it's a little bit easier, because again, fewer steps. When we're going through that somatic recombination, it's basically again, just picking a random variable and merging it with a random junctional from there. And then from there, after we've done that VJ joining, we will then add that onto the constant, and we're good, and you've made your functional light chain, your functional light chain is variable junctional constant. The L in the front's just the leader sequence where it tells it where to start. Now when we go through, so this is the right panel here, next slide, I'm sorry, left panel, left panel here, now we're going through the right panel here. When we're talking about this heavy chain, it first merges D and J first during that somatic recombination. So I always like to joke that the party doesn't start until the DJ shows up. So DJ is first, DJ is first, then you have the party. So you have a DJ recombination first, and then the variable will be clustered on here, and after we have that VDJ joining, it will then pick one of these other constant regions in order to fuse on and determine what we're gonna be. And so it's just for simplicity's sake showing you mu here. So if we had this VDJ and this constant mu, which immunoglobulin molecule are we going to make? IgM, because mu is M, and so it'll combine that here too. It is showing that each immunoglobulin domain is encoded by a separate exon, so it's just spacing them out pretty much here. And we have two additional membrane coding exons known as MC at the end here that will specify this hydrophobic sequence that will help bind it onto the membrane. So this will become important later when we talk about cleaving it off the surface. So for right now, just know that after we have that constant, this MC region here is what attaches it to that B cell membrane so that it becomes that B cell receptor and is bound there. Don't memorize this, but again, it's just showing you the possible combinations overall within and what we can do when we do combine overall. So no, again, light chains don't have any diversity. Well, actually, I'm sorry, I take that back. This is the rough estimate. I'm cool with the numbers I gave you guys before, just because I don't want you to be too overwhelmed. Laura said 30, 35, roughly. It's a little bit more than that. And I think they are adding on some slightly different. Go back that way. I went too far. Nope, stayed. There are slightly different changes to it. Notice there's a little bit more of a range. There is some interpersonal differences in variation, but again, the roughly average 30, 35 are fine with me. Yes, Ken. What is the function of the leader sequence? I'm sorry? What's the function of the leader sequence? Leader is just where it's gonna start with recombination. It's just where it binds on, starts, reads this way. The leader tells it where to go first. So it's not included in the final product? No, I usually, it leaders in front of everything, so we kind of ignore it, because I mean, it's important, it's there, it starts everything, but when we think about it, we're mostly talking about, you know, the VDJ or VJC leader is always in front. Okay, so now that it moves on, we should, there we go, should have a quick video on it. It's the same one we've seen before. Granted, we watched it with T cells, and I did mention it is slightly different. Textbook videos for this module have started to be posted already under course resources. At least the videos for this week are posted, so in case you wanna go to the course resources tab on Canvas, scroll down to textbook videos. They're already linked in there from module three, if you ever wanna watch them on your own without having to sift through a whole PowerPoint. That is, okay, not my password, nope. Immunoglobulin genes are composed of separated segments of DNA that become joined together by a process called somatic recombination to make a functional gene. In heavy chain genes, there are three gene segments, the variable or V segment, a diversity or D gene segment, and the joining or J segment. Light chain genes, such as those shown here, have only two gene segments, the V and the J segments. Gene segments that can be recombined have specific sequence motifs adjacent to them called recombination signal sequence or RSS motifs. A protein complex containing the products of the recombination activator genes, RAG1 and RAG2, binds specifically to the RSS motifs. In this example, flanking a V gene segment and a J gene segment. The individual gene segments to whose flanking RSS motifs the RAG protein complexes bind are selected at random from a number of copies present at each gene locus. The RAG protein complexes bring together the gene segments to be recombined and cleave the DNA exactly at the junction of the gene segment and its adjoining RSS motif. The cleavage creates a hairpin of DNA at the end of the gene segments and double-stranded breaks at the ends of the RSS motifs. Additional proteins, DNA-dependent protein kinase, Q, Artemis, and a dimer of DNA ligase and XRCC4 are incorporated into a large complex with the RAG proteins. These RSS ends are joined, forming what is called the signal joint to create a closed circle of DNA that plays no further role in the recombination process. The DNA hairpins at the ends of the gene segments are then cleaved. An additional enzyme, terminal deoxynucleotide transferase, or TDT, is recruited and adds additional nucleotides to the ends of the DNA strands. The other enzymes in the complex ligate together the two ends of the gene segments, completing the recombination process. So there's a little bit, there's a little bit more there than we talked about. So I'll tell you what is relevant from that because there are some slides with definitions. So then obviously you guys know that's gonna be fair game. That's kind of a nice visualization of showing how it combines the different sections. And again, it creates that kind of like signal joint, which is this excess DNA that we're basically cutting out because we don't need it anymore. So we'll go through that on some pictures here too. So it's showing the lambda, the kappa, and the heavy chains here. The video did talk about RSS, or recombination signal sequences. They are conserved sequences of non-coding DNA that are recognized by RAG-1 and RAG-2. Those are the enzymes involved in recombination. So all I need you guys to know about RAG-1 and RAG-2, they're the ones involved in recombining all of these different things here too. And so they're recognized by RAG-1 and RAG-2 enzyme complex during that V, D is in parentheses, because again, light chains don't have the diversity. So that's why it's in parentheses because only some of them have diversity segments. During that V, D, J, recombination and immature B cells here too. So we have two different types of RSS that we have. We have a nonamer, which is that nine base pairs here. It's shown in purple. And we have a heptamer, which is shown in orange here, kind of coding either side here. And it does have spacers in between there, kind of breaking it up just a little bit here too. And so we also have a 23 base pair separator here. So there's just nice breaks in it, but this allows the body to know where it can combine the different sections when it's joining a variable and a junctional. And you have this between pretty much each diversity here, if we're talking about heavy chain, each junctional and each variable. So there's a nice mixture here, but it's how the body knows where different recombination enzymes should bind on, how we can loop them back together and actually connect them and which ones we should be connecting together. Because again, spacers, it's extra space. You don't need it. It's not really relevant. And so we have these nice different recombination signal sequences that again, tell the body kind of where we are going to cut and start recombining here. And then spacers are added in to provide a little bit more space overall. Again, I showed the video from before, rag one and rag two are the enzymes involved in this recombination here too. And so again, using those RSS sequences, it kind of knows where to bind on. It helps to identify where to cleave here too. So it is showing that somatic recombination of this variable here and this junctional here because it wants to combine these two. So you remember, like we have a long chain of this. These are the extra parts that we really don't need anymore. We're trying to combine this variable with whichever junctional this one is. This one says junctional one. You had extra junctional here too. And so the binding of this rag one, rag two complex on either side allows it to kind of pin together, form a loop, like if I were to take a rope. We're gonna use a pen. Don't tell IT. We have this, it loops around. You get, because we're trying to combine this portion and this portion. When you do that, you get that nice loop of things that we don't need. And that's what gets cleaved off. As a signal joint leaves because we don't need it anymore. And then we're connected in that short little region right there. And so that's what it's showing here. The signal joint is that extra piece that gets cut out when we stitch those two places that got cut back together. So it shows you that we've got this now coding joint, which is basically just the term for the thing that we've combined that now codes for something. It's two proteins directly adjacent to each other. This is slightly longer detail from your textbook picture, but kind of the simplicity that I went through just now is the extent that I'm really comfortable with. I want you to know what the signal joint is. You know, that it's the two cleavage sites bound together with RAG1, RAG2 that creates that coding joint when we combine that variable with that junctional. Recombination enzymes like RAG1 and RAG2 produce additional diversity in the angent binding sites. We have RAG1 and RAG2, they're involved in, you know, cutting and getting things together too. But that's when TDT comes in as well. And so, in short, this one's just showing you junctional diversity with the D and the J. This can happen with other segments as well. This is just the example that it's using here. And so, it's combining those two RSS sequences, because again, we're trying to combine this diversity with this junctional with heavy chain recombination. And again, this is that RSS extra sequence. You know, it's just kind of showing it where to join. And so that RAG complex cleaves out those two pieces here. We formed those little hairpins. Remember they talked about how it had to cut those guys open to then attach them to each other here. So that's what happens here. It cut and kind of opened these guys up. And so that's where those letters came from. The G moved up there, the A followed it. It's kind of just opening up into a chain instead of a loop. Same thing here. It's now flipping up to read there. When they overlap it like that, and so those are the things known as hairpins. If it looks like a little loop, those are known as hairpins. Those need to be cleaved first. And then we have extra nucleotides that are here. By definition, all I want you to know are the definitions. So P nucleotides, here are extra nucleotides that form a palindromic sequence because again, they're the opposite of what you saw here. Does that make sense? Palindrome opposite like mom. M-O-M is mom forward and backwards. Dad. I can't think of any other palindromes right now. What? What? Bob. Bob is also palindrome. Can anyone think of a longer palindrome? I've used like mom, Bob, and dad, the easiest ones. Oh, race car is one. Race car is the coolest one because it's a full longer word. But yes, race car, forward and backwards. And so again, they're talking about palindromes because again, it's what the letters line up with. Like if you were to, you know, A with T, G with C, are we comfortable with the basics of molecular biology? You know, the letters go together. If you don't know that, let me know. I hate to reference something. You have no idea what I'm talking about. And then you mess something up on an exam. But anyway, they have those letters. So we have these palindromic sequences here and that's why they're called P nucleotides, P for palindrome. But they are what occurs when we first cleave that little hairpin loop to have these chains here, which in theory, we're gonna try to combine. But then we have this really cool option that we talk about, especially with B cell receptors, where terminal deoxynucleotide titled transferase, which we did loosely introduce with T cells. We can also see it with T cells. I was not trying to overwhelm you guys too much with that. But TDT can actually come in and add extra nucleotides. That's why they're like super colorful right here. TDT can come in and add just any random nucleotide that it wants to. And then the body will obviously match it up because you know, A with T, G with C, C with G, T with A. The letters line up to each other and they will add it in. And so these new nucleotides, that's why I call them N nucleotides or new nucleotides. They're not encoded in the germline. They are extra ones added in. But they provide additional diversity because every time this happens, when we're fusing together, you know, D and J or V, D and J, it's adding in these extra sequences that creates more variability. Because if you know that you add in even like one additional letter, it shifts the whole sequence of what you're reading. It changes all downstream proteins created from it. And so it gives you this nice variability and this massively cool broad adaptive immune response as a consequence of that. So again, with P and N, palindromic nucleotides, they're the final sequence here when they're first cleaved by that hairpin loop here. And N nucleotides are not encoded in the genome, but they add additional diversity. They're not part of diversity, they're part of just, you know, you can add them at any point but they increase the variability and diversity of. I know I keep using two adjectives of things that we just are supposed to talk about, the variable reason and diversity, but they provide more and broad diversity is the best word you can say for it for these sequences here. And they're added in, and nucleotide specifically by TDT. So then what it does is that final step, those single strands will pair and through the action of exonuclease. And I don't want you to know anything more than that. Most of you guys should know some of these enzymes from like biochem and molecular biology where they talk about it. Just know that combination of exonuclease, polymerase and ligase fuses that all together to make a fully combined double-stranded DNA molecule that's repaired to give you this full coding joint overall. Sounds good. And that coding joint for reference would be visualized kind of within here. That's where that extra diversity would be included. It's not the true diversity section, it's just that extra nucleotides that have been added in that create, you know, changes to that sequence here would be found kind of within that coding joint there when they're merged together, fused and merged together. So in naive B cells, alternative mRNA splicing produces IgM and IgD of the same antigen specificity. So we're now about to get into a very complicated topic and a lot of students have issues visualizing. We will talk about it again when we go through B cell development and talk about where these guys exist. But what's strange and unique about these different heavy chain constant regions is that mu and delta are incredibly close to each other. Which means that when we're splicing RNA for some reason we end up expressing both IgM and IgD and they think that plays into why we see both IgM and IgD expressed on the same surface of B cells when they are first released from the bone marrow. They think it's part of that maturation process. And this kind of thing where I'm like, it's like gray area of like, clearly we know D is important but we're not really sure what. Truly it does but it's involved in that. And it's due to that co-expression, the extent that I want you to know that co-expression is due to alternative mRNA splicing. We're not gonna go through tons of the mechanisms of it but it's because they're right next to each other on the genome that that's why they end up showing up together here. So if we were to actually write out that whole constant chunk from before, remember we mentioned nine, these are them here. And these are the order in which they occur and it also explains why when we see isotype switching later, which we will talk about, immunoglobulin molecules. So each individual different type of immunoglobulin molecules are other than isotype. They're similar pairs of, you know, IgM kind of looks like IgD. IgD kind of looks like IgD, they're similar in their shapes related to it. They go in a specific order when they switch. It's always IgM, IgG, IgA and then IgE. And it makes sense because those are the order in which they first appear in the constant regions. If you want to write that down again, I will repeat it a lot. The first immunoglobulin molecule we make is IgM, then followed by IgG, then IgA and then IgE. And I will repeat that again when we go through isotype switching and some of the effector functions, but it's because of the order in which they appear on this heavy chain genome. And again, notice how you have few like larger gaps between all of them, but IgM, mu heavy chain and the delta heavy chain are like directly adjacent to each other. This is why both of them will end up getting expressed on the surface of a cell together. So when we first create a B cell, they will have an IgM on the surface and an IgD first as they're going through development. They lose the IgD and then they are considered fully mature. And we will talk about that a lot more here. And so again, it is showing you all the different functional. Notice again, how we have gamma three first. Unfortunately, when they were going through, they didn't know this one was here. So they had named one two, then realized three was up here and then went back and named it four. But we actually have four different subtypes of IgG. We have IgG one, IgG two, IgG three and IgG four. You also have two types of IgA, IgA one and IgA two. Besides that, it's pretty much the standard same. You have one mu, one delta, four IgGs in yellow, two IgAs in orange, and then that one epsilon here. And those are those heavy chains. So depending on which one of these these bind to first, which, and again, if we're making our first immunoglobulin molecule, it's going to bind with the mu first and then co-express that D. And so that is our first molecule here. But when we switch, it could potentially bind onto one of these other guys here. And usually it's that G first. And so here, you know, it would splice this area out and end up being able to, you know, become then switch out the heavy chain basically, swap it out just a little bit. And then you have an IgG antibody versus if we do that again and we fuse with A, it would then trade out basically the heavy chain and produce an alpha heavy chain, thus making the molecule now IgA. It's a little complicated to picture. The more we talk about it, you're like, oh yeah, that makes a little bit more sense as we go through it. But this is just a very introduction with genetics. If it's not sticking, just kind of bear with it. We will keep going through this until you guys are comfortable with it, okay? I would like you to know the order in which they appear because it is relevant and important to which potential immunoglobulin can be made during that splicing. So that's why it's important that in the big picture, you know, IgM first could switch to IgG, could switch to IgA, could switch to IgE. And so it is also not showing the individual exons because it's just trying to put to scale here. So that is important here. But I could give you this chain here and say, you know, you have a VDJ recontin like that recombines with this one here, like what type of immunoglobulin molecule would that make? IgG what? IgG1 is correct. Or I said, oh, it fused with this one. What would that be? IgA2, yep. So there's some application questions kind of with this overall. So again, we talked about that co-expression because IgD and IgM are so close to each other on that genome here, like incredibly close. That we have issues where they will both express IgM and IgE. So basically all it's showing you is two very similar pictures, except right here, it's merging that VDJ with this constant mu versus over here, it's merging that VDJ with the delta segment. Because they are so close to each other, that is why they both get co-expressed because as the body is just kind of going through and reading it and sequencing it, there's really not a ton of space between these two. And as a consequence, it will produce both and both will be found on the surface of a B cell. So minus like some rare exceptional, like we've really screwed up molecular biology in some way, the only time you will see two immunoglobulins of different specificities together on the same B cell would be IgM and IgD. You don't see IgM and IgA on the same cell. You don't see IgM and IgG. You only see IgM and IgD together and it's important with development here. So it's not showing you all of it here, but it is showing you it's kind of this poly A tail here, this polyadenylated tail, poly meaning multiple, A meaning adenosine, it binds onto that membrane here. So it's just kind of showing you that that's the tail that helps attach it into the B cell membrane. And we'll talk about this a lot more and it's relevant a lot more when we talk about the stages of B cell development as it starts to express the immunoglobulin molecules on the surface, but for right now, again, just know that you can have co-expression, which means both are expressed at the same time with IgM and IgD and it's regulated by that alternative mRNA splicing. There's that big picture takeaway of these guys here. And so it is showing that polyadenylated tail. When you're seeing abbreviations, you'll see a little P for poly. And it has that adenylated tail. It's showing you this mu means M and then the M denotes the site produced by the membrane-bound immunoglobulin. We'll talk about S in a few minutes here too. But again, it is doing splicing and cleaving here that polyadenylated tail just to bind on. But again, I want to, so say I have it in a really difficult exam question. I give you an immunoglobulin molecule that you, it's non-descript, you can't really tell what type it is, but I give you information where I've got a polyadenylated mu M. You would be able to tell me that it is IgM and it is membrane-bound because you have enough information here because it gives you the mu so you'd know it's an M heavy chain, basically a mu heavy chain, or IgM. And if you have this M here versus the S, which we will talk about, it means membrane-bound. S means secreted and we will elaborate on that because it's actually hidden in between here. But for simplicity's sake, your textbook's introducing you to this first before adding that additional information that we also have a splice piece too that we can like cleave and then, you know, release it as a secreted form here too. So the immunoglobulin is first made in the membrane-bound form that is present on the B cell surface. You guys know this, B cells have it bound on their surface. It's plasma cells that can then cleave it and release it. And so when we first talk about the B cell receptor, this is what expressed on the surface of the B cell at first. When we talk about the B cell receptor complex, like we talked about with T cell receptor, if I ask for just B cell receptor, I'm just talking about this molecule here. If I talk about B cell receptor complex, I want these two here in Ig alpha and Ig beta found on both sides of this B cell receptor. They are the equivalent to CD3 with the T cells. Notice how they have those long tails here. They are there because they are what is needed for that intracellular signaling. They are what have the ITAMs, because again, notice the tail on the B cell receptor does not really extend that far past the cell membrane. As a consequence, you could have something bind onto the B cell receptor, but if you don't have Ig alpha and Ig beta on the side, again, I know poorly named because we are still talking about Ig molecules too. And these guys are not Ig molecules. They're Ig alpha and Ig beta, but they are adjacent and they're responsible for signaling. They're what have the cytoplasmic tails with the ITAMs. And so when something binds on, the signal travels down and it's these guys that then activate inside of the cell with phosphorylation of all the different ITAMs that eventually leads to signaling to the nucleus to activate the B cell to continue on. And so again, if I do talk about the B cell receptor complex, I'm talking about the B cell receptor with Ig alpha and Ig beta. If I'm just talking about the B cell receptor, I'm just talking about the immunoglobulin molecule. Sounds good. Ig alpha and Ig beta are also called CD79A and B. Unfortunately, they were trying to make it a little bit better because this is a horrific nomenclature. But again, it's more complicated that way. I'm not gonna be like, oh, at least I lined it well that 79A is alpha and 79B is beta, but that's it. We will pick up the rest of it next class, but it's basically gonna talk about how we do secreted versus not. So again, I mentioned there are some extra things on here too that we have a section for secreted versus membrane balance. We'll elaborate on that next class and then we will pick up there. Deep breaths, you got this. Just go through it slowly. If you have questions, come to office hours earlier. Not like earlier time-wise. I just mean earlier in the semester. Don't show up right before exams confused. You can do that too, but it would be easier if you do it earlier. Need one quick.