Fundamentals of Remote Sensing PDF
Document Details
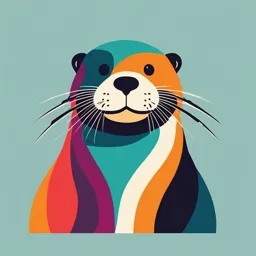
Uploaded by AmenableIntelligence
Universidad de Birmingham
Tags
Summary
This document is a tutorial on the fundamentals of remote sensing. It discusses the science and art of collecting information about the Earth's surface without physical contact. The tutorial covers key concepts like electromagnetic radiation, interactions with the atmosphere, and sensor types and applications in different fields. It's meant for an introductory undergraduate course in remote sensing
Full Transcript
Fundamentals of Remote Sensing A Canada Centre for Remote Sensing Remote Sensing Tutorial Natural Resources Canada Ressources naturelles Canada Fundamentals of Remote Sensing - Table of Contents Page 2 Table of Contents 1. Introduction 1.1 What is Remote Sensing? 1.2 Electromagnetic Radiatio...
Fundamentals of Remote Sensing A Canada Centre for Remote Sensing Remote Sensing Tutorial Natural Resources Canada Ressources naturelles Canada Fundamentals of Remote Sensing - Table of Contents Page 2 Table of Contents 1. Introduction 1.1 What is Remote Sensing? 1.2 Electromagnetic Radiation 1.3 Electromagnetic Spectrum 1.4 Interactions with the Atmosphere 1.5 Radiation - Target 1.6 Passive vs. Active Sensing 1.7 Characteristics of Images 1.8 Endnotes Did You Know Whiz Quiz and Answers 5 7 9 12 16 19 20 22 23 27 2. Sensors 2.1 On the Ground, In the Air, In Space 2.2 Satellite Characteristics 2.3 Pixel Size, and Scale 2.4 Spectral Resolution 2.5 Radiometric Resolution 2.6 Temporal Resolution 2.7 Cameras and Aerial Photography 2.8 Multispectral Scanning 2.9 Thermal Imaging 2.10 Geometric Distortion 2.11 Weather Satellites 2.12 Land Observation Satellites 2.13 Marine Observation Satellites 2.14 Other Sensors 2.15 Data Reception 2.16 Endnotes Did You Know Whiz Quiz and Answers 34 36 39 41 43 44 45 48 50 52 54 60 67 70 72 74 75 83 Canada Centre for Remote Sensing Fundamentals of Remote Sensing - Table of Contents Page 3 3. Microwaves 3.1 Introduction 3.2 Radar Basic 3.3 Viewing Geometry & Spatial Resolution 3.4 Image distortion 3.5 Target interaction 3.6 Image Properties 3.7 Advanced Applications 3.8 Polarimetry 3.9 Airborne vs Spaceborne 3.10 Airborne & Spaceborne Systems 3.11 Endnotes Did You Know Whiz Quiz and Answers 92 96 99 102 106 110 114 117 123 125 129 131 135 4. Image Analysis 4.1 Introduction 4.2 Visual interpretation 4.3 Digital processing 4.4 Preprocessing 4.5 Enhancement 4.6 Transformations 4.7 Classification 4.8 Integration 4.9 Endnotes Did You Know Whiz Quiz and Answers 141 144 147 149 154 158 161 164 166 167 170 5. Applications 5.1 Introduction 5.2 Agriculture 174 177 Crop Type Mapping Crop Monitoring 5.3 Forestry Clear cut Mapping Species identification Burn Mapping Canada Centre for Remote Sensing 184 Fundamentals of Remote Sensing - Table of Contents 5.4 Geology 222 Planimetry DEMs Topo Mapping 5.9 Oceans & Coastal 215 Rural/Urban change Biomass Mapping 5.8 Mapping 209 Type and Concentration Ice Motion 5.7 Land Cover 203 Flood Delineation Soil Moisture 5.6 Sea Ice 196 Structural Mapping Geologic Units 5.5 Hydrology Page 4 232 Ocean Features Ocean Colour Oil Spill Detection 5.10 Endnotes Did You Know Whiz Quiz 240 241 250 Credits 254 Permissions 256 Download 257 Notes for Teachers 258 Canada Centre for Remote Sensing Page 5 Section 1.1 What is Remote Sensing? 1. Introduction to Fundamentals 1.1 What is Remote Sensing? So, what exactly is remote sensing? For the purposes of this tutorial, we will use the following definition: "Remote sensing is the science (and to some extent, art) of acquiring information about the Earth's surface without actually being in contact with it. This is done by sensing and recording reflected or emitted energy and processing, analyzing, and applying that information." In much of remote sensing, the process involves an interaction between incident radiation and the targets of interest. This is exemplified by the use of imaging systems where the following seven elements are involved. Note, however that remote sensing also involves the sensing of emitted energy and the use of non-imaging sensors. 1. Energy Source or Illumination (A) - the first requirement for remote sensing is to have an energy source which illuminates or provides electromagnetic energy to the target of interest. 2. Radiation and the Atmosphere (B) - as the energy travels from its source to the target, it will come in contact with and interact with the atmosphere it passes through. This interaction may take place a second time as the energy travels from the target to the sensor. 3. Interaction with the Target (C) - once the energy makes its way to the target through the atmosphere, it interacts with the target depending on the properties of both the target and the radiation. Canada Centre for Remote Sensing Section 1.1 What is Remote Sensing? Page 6 4. Recording of Energy by the Sensor (D) - after the energy has been scattered by, or emitted from the target, we require a sensor (remote - not in contact with the target) to collect and record the electromagnetic radiation. 5. Transmission, Reception, and Processing (E) - the energy recorded by the sensor has to be transmitted, often in electronic form, to a receiving and processing station where the data are processed into an image (hardcopy and/or digital). 6. Interpretation and Analysis (F) - the processed image is interpreted, visually and/or digitally or electronically, to extract information about the target which was illuminated. 7. Application (G) - the final element of the remote sensing process is achieved when we apply the information we have been able to extract from the imagery about the target in order to better understand it, reveal some new information, or assist in solving a particular problem. These seven elements comprise the remote sensing process from beginning to end. We will be covering all of these in sequential order throughout the five chapters of this tutorial, building upon the information learned as we go. Enjoy the journey! Canada Centre for Remote Sensing Section 1.2 Electromagnetic Radiation Page 7 1.2 Electromagnetic Radiation As was noted in the previous section, the first requirement for remote sensing is to have an energy source to illuminate the target (unless the sensed energy is being emitted by the target). This energy is in the form of electromagnetic radiation. All electromagnetic radiation has fundamental properties and behaves in predictable ways according to the basics of wave theory. Electromagnetic radiation consists of an electrical field(E) which varies in magnitude in a direction perpendicular to the direction in which the radiation is traveling, and a magnetic field (M) oriented at right angles to the electrical field. Both these fields travel at the speed of light (c). Two characteristics of electromagnetic radiation are particularly important for understanding remote sensing. These are the wavelength and frequency. Canada Centre for Remote Sensing Section 1.2 Electromagnetic Radiation Page 8 The wavelength is the length of one wave cycle, which can be measured as the distance between successive wave crests. Wavelength is usually represented by the Greek letter lambda (λ). Wavelength is measured in metres (m) or some factor of metres such as nanometres (nm, 10-9 metres), micrometres (µm, 10-6 metres) (µm, 10-6 metres) or centimetres (cm, 10-2 metres). Frequency refers to the number of cycles of a wave passing a fixed point per unit of time. Frequency is normally measured in hertz (Hz), equivalent to one cycle per second, and various multiples of hertz. Wavelength and frequency are related by the following formula: Therefore, the two are inversely related to each other. The shorter the wavelength, the higher the frequency. The longer the wavelength, the lower the frequency. Understanding the characteristics of electromagnetic radiation in terms of their wavelength and frequency is crucial to understanding the information to be extracted from remote sensing data. Next we will be examining the way in which we categorize electromagnetic radiation for just that purpose. Canada Centre for Remote Sensing Section 1.3 The Electromagnetic Spectrum Page 9 1.3 The Electromagnetic Spectrum The electromagnetic spectrum ranges from the shorter wavelengths (including gamma and x-rays) to the longer wavelengths (including microwaves and broadcast radio waves). There are several regions of the electromagnetic spectrum which are useful for remote sensing. For most purposes, the ultraviolet or UV portion of the spectrum has the shortest wavelengths which are practical for remote sensing. This radiation is just beyond the violet portion of the visible wavelengths, hence its name. Some Earth surface materials, primarily rocks and minerals, fluoresce or emit visible light when illuminated by UV radiation. Canada Centre for Remote Sensing Section 1.3 The Electromagnetic Spectrum Page 10 The light which our eyes - our "remote sensors" - can detect is part of the visible spectrum. It is important to recognize how small the visible portion is relative to the rest of the spectrum. There is a lot of radiation around us which is "invisible" to our eyes, but can be detected by other remote sensing instruments and used to our advantage. The visible wavelengths cover a range from approximately 0.4 to 0.7 µm. The longest visible wavelength is red and the shortest is violet. Common wavelengths of what we perceive as particular colours from the visible portion of the spectrum are listed below. It is important to note that this is the only portion of the spectrum we can associate with the concept of colours. Violet: 0.4 - 0.446 µm Blue: 0.446 - 0.500 µm Green: 0.500 - 0.578 µm Yellow: 0.578 - 0.592 µm Orange: 0.592 - 0.620 µm Red: 0.620 - 0.7 µm Blue, green, and red are the primary colours or wavelengths of the visible spectrum. They are defined as such because no single primary colour can be created from the other two, but all other colours can be formed by combining blue, green, and red in various proportions. Although we see sunlight as a uniform or homogeneous colour, it is actually composed of various wavelengths of radiation in primarily the ultraviolet, visible and infrared portions of the spectrum. The visible portion of this radiation can be shown in its Canada Centre for Remote Sensing Section 1.3 The Electromagnetic Spectrum Page 11 component colours when sunlight is passed through a prism, which bends the light in differing amounts according to wavelength. The next portion of the spectrum of interest is the infrared (IR) region which covers the wavelength range from approximately 0.7 µm to 100 µm - more than 100 times as wide as the visible portion! The infrared region can be divided into two categories based on their radiation properties - the reflected IR, and the emitted or thermal IR. Radiation in the reflected IR region is used for remote sensing purposes in ways very similar to radiation in the visible portion. The reflected IR covers wavelengths from approximately 0.7 µm to 3.0 µm. The thermal IR region is quite different than the visible and reflected IR portions, as this energy is essentially the radiation that is emitted from the Earth's surface in the form of heat. The thermal IR covers wavelengths from approximately 3.0 µm to 100 µm. The portion of the spectrum of more recent interest to remote sensing is the microwave region from about 1 mm to 1 m. This covers the longest wavelengths used for remote sensing. The shorter wavelengths have properties similar to the thermal infrared region while the longer wavelengths approach the wavelengths used for radio broadcasts. Because of the special nature of this region and its importance to remote sensing in Canada, an entire chapter (Chapter 3) of the tutorial is dedicated to microwave sensing. Canada Centre for Remote Sensing Section 1.4 Interactions with the Atmosphere Page 12 1.4 Interactions with the Atmosphere Before radiation used for remote sensing reaches the Earth's surface it has to travel through some distance of the Earth's atmosphere. Particles and gases in the atmosphere can affect the incoming light and radiation. These effects are caused by the mechanisms of scattering and absorption. Scattering occurs when particles or large gas molecules present in the atmosphere interact with and cause the electromagnetic radiation to be redirected from its original path. How much scattering takes place depends on several factors including the wavelength of the radiation, the abundance of particles or gases, and the distance the radiation travels through the atmosphere. There are three (3) types of scattering which take place. Canada Centre for Remote Sensing Section 1.4 Interactions with the Atmosphere Page 13 Rayleigh scattering occurs when particles are very small compared to the wavelength of the radiation. These could be particles such as small specks of dust or nitrogen and oxygen molecules. Rayleigh scattering causes shorter wavelengths of energy to be scattered much more than longer wavelengths. Rayleigh scattering is the dominant scattering mechanism in the upper atmosphere. The fact that the sky appears "blue" during the day is because of this phenomenon. As sunlight passes through the atmosphere, the shorter wavelengths (i.e. blue) of the visible spectrum are scattered more than the other (longer) visible wavelengths. At sunrise and sunset the light has to travel farther through the atmosphere than at midday and the scattering of the shorter wavelengths is more complete; this leaves a greater proportion of the longer wavelengths to penetrate the atmosphere. Mie scattering occurs when the particles are just about the same size as the wavelength of the radiation. Dust, pollen, smoke and water vapour are common causes of Mie scattering which tends to affect longer wavelengths than those affected by Rayleigh scattering. Mie scattering occurs mostly in the lower portions of the atmosphere where larger particles are more abundant, and dominates when cloud conditions are overcast. The final scattering mechanism of importance is called nonselective scattering. This occurs when the particles are much larger than the wavelength of the radiation. Water droplets and large dust particles can cause this type of scattering. Nonselective scattering gets its name from the fact that all wavelengths are scattered about equally. This type of scattering causes fog and clouds to appear white to our eyes because blue, green, and red light are all scattered in approximately equal quantities (blue+green+red light = white light). Canada Centre for Remote Sensing Section 1.4 Interactions with the Atmosphere Page 14 Absorption is the other main mechanism at work when electromagnetic radiation interacts with the atmosphere. In contrast to scattering, this phenomenon causes molecules in the atmosphere to absorb energy at various wavelengths. Ozone, carbon dioxide, and water vapour are the three main atmospheric constituents which absorb radiation. Ozone serves to absorb the harmful (to most living things) ultraviolet radiation from the sun. Without this protective layer in the atmosphere our skin would burn when exposed to sunlight. You may have heard carbon dioxide referred to as a greenhouse gas. This is because it tends to absorb radiation strongly in the far infrared portion of the spectrum - that area associated with thermal heating - which serves to trap this heat inside the atmosphere. Water vapour in the atmosphere absorbs much of the incoming longwave infrared and shortwave microwave radiation (between 22µm and 1m). The presence of water vapour in the lower atmosphere varies greatly from location to location and at different times of the year. For example, the air mass above a desert would have very little water vapour to absorb energy, while the tropics would have high concentrations of water vapour (i.e. high humidity). Because these gases absorb electromagnetic energy in very specific regions of the spectrum, they influence where (in the spectrum) we can "look" for remote sensing purposes. Those areas of the spectrum which are not severely influenced by atmospheric absorption and thus, are useful to remote sensors, are called atmospheric windows. By comparing the characteristics of the two most common energy/radiation sources (the sun and the earth) with the atmospheric windows available to us, we can define those wavelengths that we can use most effectively for remote sensing. The visible portion of the spectrum, to which our eyes are most sensitive, corresponds to both an atmospheric window and the peak energy level of the sun. Note also that heat energy emitted by the Earth corresponds to a window around 10 µm in the thermal IR portion of the spectrum, while the large window at wavelengths beyond 1 mm is associated with the Canada Centre for Remote Sensing Section 1.4 Interactions with the Atmosphere Page 15 microwave region. Now that we understand how electromagnetic energy makes its journey from its source to the surface (and it is a difficult journey, as you can see) we will next examine what happens to that radiation when it does arrive at the Earth's surface. Canada Centre for Remote Sensing Section 1.5 Radiation - Target Interactions Page 16 1.5 Radiation - Target Interactions Radiation that is not absorbed or scattered in the atmosphere can reach and interact with the Earth's surface. There are three (3) forms of interaction that can take place when energy strikes, or is incident (I) upon the surface. These are: absorption (A); transmission (T); and reflection (R). The total incident energy will interact with the surface in one or more of these three ways. The proportions of each will depend on the wavelength of the energy and the material and condition of the feature. Absorption (A) occurs when radiation (energy) is absorbed into the target while transmission (T) occurs when radiation passes through a target. Reflection (R) occurs when radiation "bounces" off the target and is redirected. In remote sensing, we are most interested in measuring the radiation reflected from targets. We refer to two types of reflection, which represent the two extreme ends of the way in which energy is reflected from a target: specular reflection and diffuse reflection. Canada Centre for Remote Sensing Section 1.5 Radiation - Target Interactions Page 17 When a surface is smooth we get specular or mirror-like reflection where all (or almost all) of the energy is directed away from the surface in a single direction. Diffuse reflection occurs when the surface is rough and the energy is reflected almost uniformly in all directions. Most earth surface features lie somewhere between perfectly specular or perfectly diffuse reflectors. Whether a particular target reflects specularly or diffusely, or somewhere in between, depends on the surface roughness of the feature in comparison to the wavelength of the incoming radiation. If the wavelengths are much smaller than the surface variations or the particle sizes that make up the surface, diffuse reflection will dominate. For example, finegrained sand would appear fairly smooth to long wavelength microwaves but would appear quite rough to the visible wavelengths. Let's take a look at a couple of examples of targets at the Earth's surface and how energy at the visible and infrared wavelengths interacts with them. Leaves: A chemical compound in leaves called chlorophyll strongly absorbs radiation in the red and blue wavelengths but reflects green wavelengths. Leaves appear "greenest" to us in the summer, when chlorophyll content is at its maximum. In autumn, there is less chlorophyll in the leaves, so there is less absorption and proportionately more reflection of the red wavelengths, making the leaves appear red or yellow (yellow is a combination of red and green wavelengths). The internal structure of healthy leaves act as excellent diffuse reflectors of near-infrared wavelengths. If our eyes were sensitive to near-infrared, trees would appear extremely bright to us at these wavelengths. In fact, measuring and monitoring the near-IR reflectance is one way that scientists can determine how healthy (or unhealthy) vegetation may be. Water: Longer wavelength visible and near infrared radiation is absorbed more by water than shorter visible wavelengths. Thus water typically looks blue or blue-green due to stronger reflectance at these shorter wavelengths, and darker if viewed at red or near infrared wavelengths. If there is suspended sediment present in the upper layers of the water body, then this will allow better reflectivity and a brighter appearance of the water. The apparent colour of the water will show a slight shift to longer Canada Centre for Remote Sensing Section 1.5 Radiation - Target Interactions Page 18 wavelengths. Suspended sediment (S) can be easily confused with shallow (but clear) water, since these two phenomena appear very similar. Chlorophyll in algae absorbs more of the blue wavelengths and reflects the green, making the water appear more green in colour when algae is present. The topography of the water surface (rough, smooth, floating materials, etc.) can also lead to complications for water-related interpretation due to potential problems of specular reflection and other influences on colour and brightness. We can see from these examples that, depending on the complex make-up of the target that is being looked at, and the wavelengths of radiation involved, we can observe very different responses to the mechanisms of absorption, transmission, and reflection. By measuring the energy that is reflected (or emitted) by targets on the Earth's surface over a variety of different wavelengths, we can build up a spectral response for that object. By comparing the response patterns of different features we may be able to distinguish between them, where we might not be able to, if we only compared them at one wavelength. For example, water and vegetation may reflect somewhat similarly in the visible wavelengths but are almost always separable in the infrared. Spectral response can be quite variable, even for the same target type, and can also vary with time (e.g. "green-ness" of leaves) and location. Knowing where to "look" spectrally and understanding the factors which influence the spectral response of the features of interest are critical to correctly interpreting the interaction of electromagnetic radiation with the surface. Canada Centre for Remote Sensing Section 1.6 Passive vs. Active Sensing Page 19 1.6 Passive vs. Active Sensing So far, throughout this chapter, we have made various references to the sun as a source of energy or radiation. The sun provides a very convenient source of energy for remote sensing. The sun's energy is either reflected, as it is for visible wavelengths, or absorbed and then reemitted, as it is for thermal infrared wavelengths. Remote sensing systems which measure energy that is naturally available are called passive sensors. Passive sensors can only be used to detect energy when the naturally occurring energy is available. For all reflected energy, this can only take place during the time when the sun is illuminating the Earth. There is no reflected energy available from the sun at night. Energy that is naturally emitted (such as thermal infrared) can be detected day or night, as long as the amount of energy is large enough to be recorded. Active sensors, on the other hand, provide their own energy source for illumination. The sensor emits radiation which is directed toward the target to be investigated. The radiation reflected from that target is detected and measured by the sensor. Advantages for active sensors include the ability to obtain measurements anytime, regardless of the time of day or season. Active sensors can be used for examining wavelengths that are not sufficiently provided by the sun, such as microwaves, or to better control the way a target is illuminated. However, active systems require the generation of a fairly large amount of energy to adequately illuminate targets. Some examples of active sensors are a laser fluorosensor and a synthetic aperture radar (SAR). Canada Centre for Remote Sensing Section 1.7 Characteristics of Images Page 20 1.7 Characteristics of Images Before we go on to the next chapter, which looks in more detail at sensors and their characteristics, we need to define and understand a few fundamental terms and concepts associated with remote sensing images. Electromagnetic energy may be detected either photographically or electronically. The photographic process uses chemical reactions on the surface of light-sensitive film to detect and record energy variations. It is important to distinguish between the terms images and photographs in remote sensing. An image refers to any pictorial representation, regardless of what wavelengths or remote sensing device has been used to detect and record the electromagnetic energy. A photograph refers specifically to images that have been detected as well as recorded on photographic film. The black and white photo to the left, of part of the city of Ottawa, Canada was taken in the visible part of the spectrum. Photos are normally recorded over the wavelength range from 0.3 µm to 0.9 µm - the visible and reflected infrared. Based on these definitions, we can say that all photographs are images, but not all images are photographs. Therefore, unless we are talking specifically about an image recorded photographically, we use the term image. A photograph could also be represented and displayed in a digital format by subdividing the image into small equal-sized and shaped areas, called picture elements or pixels, and representing the brightness of each area with a numeric value or digital number. Indeed, that is exactly what has been done to the photo to the left. In fact, using the definitions we have just discussed, this is actually a digital image of the original photograph! The photograph was scanned and subdivided into pixels with each pixel assigned a digital number representing its relative brightness. The computer displays each digital value as different brightness levels. Sensors that Canada Centre for Remote Sensing Section 1.7 Characteristics of Images Page 21 record electromagnetic energy, electronically record the energy as an array of numbers in digital format right from the start. These two different ways of representing and displaying remote sensing data, either pictorially or digitally, are interchangeable as they convey the same information (although some detail may be lost when converting back and forth). In previous sections we described the visible portion of the spectrum and the concept of colours. We see colour because our eyes detect the entire visible range of wavelengths and our brains process the information into separate colours. Can you imagine what the world would look like if we could only see very narrow ranges of wavelengths or colours? That is how many sensors work. The information from a narrow wavelength range is gathered and stored in a channel, also sometimes referred to as a band. We can combine and display channels of information digitally using the three primary colours (blue, green, and red). The data from each channel is represented as one of the primary colours and, depending on the relative brightness (i.e. the digital value) of each pixel in each channel, the primary colours combine in different proportions to represent different colours. When we use this method to display a single channel or range of wavelengths, we are actually displaying that channel through all three primary colours. Because the brightness level of each pixel is the same for each primary colour, they combine to form a black and white image, showing various shades of gray from black to white. When we display more than one channel each as a different primary colour, then the brightness levels may be different for each channel/primary colour combination and they will combine to form a colour image. Canada Centre for Remote Sensing Section 1.8 Endnotes Page 22 1.8 Endnotes You have just completed Chapter 1 - Fundamentals of Remote Sensing. You can continue to Chapter 2 - Satellites and Sensors or first browse the CCRS Web site1 for other articles related to remote sensing fundamentals. For instance, you may want to look at some conventional2 or unconventional definitions3 of "remote sensing" developed by experts and other rif-raf from around the world. We have an explanation and calculation on just how much you need to worry about the effect of radiation4 from Canada's first remote sensing satellite: RADARSAT. The knowledge of how radiation interacts with the atmospheric is used by scientists in the Environmental Monitoring Section of CCRS to develop various "radiation products"5. Check them out! Learn more on how various targets like water6, rocks7, ice8, man-made features9, and oil slicks10 interact with microwave energy. Our Remote Sensing Glossary11 can help fill out your knowledge of remote sensing fundamentals. Try searching for specific terms of interest or review the terms in the "phenomena" category. 1http://www.ccrs.nrcan.gc.ca/ 2http://www.ccrs.nrcan.gc.ca/ccrs/learn/terms/definition/convdef_e.html 3http://www.ccrs.nrcan.gc.ca/ccrs/learn/terms/definition/unconvdef_e.html 4http://www.ccrs.nrcan.gc.ca/ccrs/learn/fun/radiation/radiation_e.html 5http://www.ccrs.nrcan.gc.ca/ccrs/rd/apps/landcov/rad/emrad_e.html 6http://www.ccrs.nrcan.gc.ca/ccrs/data/satsens/radarsat/images/man/rman01_e.html 7http://www.ccrs.nrcan.gc.ca/ccrs/data/satsens/radarsat/images/nwt/rnwt01_e.html 8http://www.ccrs.nrcan.gc.ca/ccrs/data/satsens/radarsat/images/pei/rpei01_e.html 9http://www.ccrs.nrcan.gc.ca/ccrs/rd/ana/cnfdbrig/confed_e.html 10http://www.ccrs.nrcan.gc.ca/ccrs/data/satsens/radarsat/images/uk/ruk01_e.html 11http://www.ccrs.nrcan.gc.ca/ccrs/learn/terms/glossary/glossary_e.html Canada Centre for Remote Sensing