Root Developmental Adaptation to Phosphate Starvation: A Review PDF
Document Details
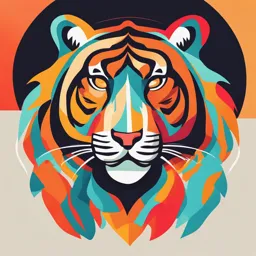
Uploaded by PainlessFeministArt
Benjamin Péret, Mathilde Clément, Laurent Nussaume and Thierry Desnos
Tags
Summary
This review examines how the model plant Arabidopsis thaliana adapts its root system to phosphorus deficiency. It discusses morphological and physiological adaptations, including root growth inhibition, enhanced lateral root formation and growth, increased root hair production. Strategies including symbiotic associations are explored, as well as the potential of using Arabidopsis as a model for improved crop selection.
Full Transcript
Review Root developmental adaptation to phosphate starvation: better safe than sorry Benjamin Péret, Mathilde Clément, Laurent Nussaume and Thierry Desnos UMR 6191 CEA, Centre National de la Recherche Scientifique, Laboratoire de Biologie du Développement des Plantes, Université d’Aix-Marseille...
Review Root developmental adaptation to phosphate starvation: better safe than sorry Benjamin Péret, Mathilde Clément, Laurent Nussaume and Thierry Desnos UMR 6191 CEA, Centre National de la Recherche Scientifique, Laboratoire de Biologie du Développement des Plantes, Université d’Aix-Marseille, 13108 Saint-Paul-lez-Durance, France Phosphorus is a crucial component of major organic ing in a shallower root system bearing more and longer molecules such as nucleic acids, ATP and membrane lateral roots as well as denser root hairs (Figure 1). Plant phospholipids. It is present in soils in the form of inor- fitness improvement through adaptation of RSA is partic- ganic phosphate (Pi), which has low availability and poor ularly relevant when competing for the acquisition of mobility. To cope with Pi limitations, plants have immobile ions such as Pi. Modifications of RSA enable evolved complex adaptive responses that include mor- the plant roots to explore the upper parts of the soil, where phological and physiological modifications. This review phosphate tends to accumulate, a strategy described as describes how the model plant Arabidopsis thaliana ‘topsoil foraging’. In many species, these alterations to adapts its root system architecture to phosphate defi- RSA are associated with alternative strategies to cope with ciency through inhibition of primary root growth, in- Pi limitations. Symbiotic associations with fungi (arbus- crease in lateral root formation and growth and cular mycorrhizae or ectomycorrhizae) are widespread and production of root hairs, which all promote topsoil for- efficient responses to increase Pi uptake [12,13]. In the aging. A better understanding of plant adaptation to low absence of mycorrhizal symbioses, some plants from dif- phosphate will open the way to increased phosphorus ferent families (e.g. Proteaceae, Casuarinaceae, Fabaceae use efficiency by crops. Such an improvement is needed and Myricaceae) can form dense clusters of lateral roots in order to adjust how we manage limited phosphorus called ‘cluster roots’ [14–17]. Despite its inability to form stocks and to reduce the disastrous environmental mycorrhizal associations and to produce cluster roots, the effects of phosphate fertilizers overuse. model plant Arabidopsis represents an ideal model to study the root developmental adaptations to low Pi and Adaptation of plants to limited phosphate availability to identify important regulators due to its simple root The exhaustion of natural resources by human activities anatomy and to the vast knowledge of its physiology, challenges our agricultural practices. Phosphorus stocks genetics and development. In this review, we discuss re- are quickly diminishing and experts agree that stocks cent studies that demonstrate how Arabidopsis thaliana could be exhausted within the next century [1,2] as a result adapts to low phosphate levels by reducing primary root of intensive use of fertilizers for crop production (Box 1). growth, enhancing lateral root formation and growth, and Phosphorus is a fundamental element that is present in increasing root hair production and elongation. In the past major organic molecules (e.g. RNA, DNA, ATP and mem- few years, the model plant has been used as a tool to brane phospholipids) and, therefore, the depletion of the identify several new elements of Pi starvation pathways world’s phosphorus cannot be resolved by substituting that will be described below (Table 1). These novel findings phosphorus usage for an alternative resource. Phosphorus will prove useful in a near future to help selection of crops (P) is present in the soil in the form of inorganic phosphate that are better adapted to low Pi levels. The objective is to (Pi) at low available concentrations, typically around 1– make more efficient use of the remaining stocks of phos- 10 mM in the soil solution [3–5]. The low availability of Pi phorus and reduce environmental pollution. Action has to results from its capacity to form insoluble complexes with be taken to anticipate a potential phosphate crisis and cations, particularly aluminum and iron under acid con- there is much to be learned from the way plants adapt to a ditions and calcium under alkaline conditions. Conse- changing environment. quently, Pi is frequently a limiting factor for plant growth and development. Phosphate starvation triggers Primary root growth inhibition a set of plant adaptive responses with the aim of reducing The alteration of the root-to-shoot growth ratio is a general Pi usage and increasing Pi uptake and recycling. This is adaptive response of plants to changes in nutrient avail- achieved through a combination of growth, developmental ability [18,19]. Deficiencies in nitrogen, P or sulfur all and metabolic responses [6–9]. Root system architecture result in a shift in dry matter allocation in favor of root (RSA) is greatly altered upon phosphate depletion, result- growth but a more comprehensive analysis of RSA responses demonstrates clear differences in the type of Corresponding author: Péret, B. ([email protected]). response. This discrepancy reflects the ability of plants to 442 1360-1385/$ – see front matter ß 2011 Elsevier Ltd. All rights reserved. doi:10.1016/j.tplants.2011.05.006 Trends in Plant Science, August 2011, Vol. 16, No. 8 Review [(Figure_1)TD$IG] Trends in Plant Science August 2011, Vol. 16, No. 8 Box 1. Diminution of phosphate resources and risks for the environment High Pi Low Pi Phosphorus is an essential macronutrient for plant growth, devel- opment and productivity. Many soils in Europe and in other parts of (iv) the world are naturally poor in plant-available phosphorus, although total soil phosphorus levels can exceed plant requirements by more than 100-fold. The reason for such apparent contradiction is that, in these soils, phosphorus is mainly unavailable to the plant because it is, for example, complexed with calcium, iron, aluminum or organic compounds. Therefore, inorganic phosphate, which is the main form of plant-assimilated phosphorus, remains limiting (iii) owing to its low solubility and high sorption capacity. Furthermore, this explains why, on average, plants effectively recover no more than 20% of phosphate present in fertilizer. In addition, the production of soluble inorganic phosphorus fertilizers depends on energy-intensive processing of sparingly soluble rock phosphates as a limited natural resource. Although (ii) the remaining lifetime of the total rock phosphate resources is expected to last for up to a few hundred years, the highest quality deposits are being depleted rapidly with predicted lifetimes of 50– 120 years. The exploitation of lower quality deposits will be (i) Key: associated with increased contamination by cadmium and other Activation heavy metals that are highly toxic to plants and, consequently, to Repression other organisms in the trophic chain. The increased demand for phosphate fertilizer has already had an impact on its price. In 2008, the phosphorus fertilizer value TRENDS in Plant Science temporarily spiked at more than US$500 per tonne (five times the Figure 1. Root architectural adaptation to soil phosphate availability. In low average price in 2007) as a result of speculation. Nevertheless, even phosphate conditions, primary root growth is strongly inhibited (i), root hair though a decrease was observed after this peak, recent prices remain production and length are increased (ii), and lateral root formation (iii) and growth high (on average more than double the price in 2007). Moreover, (iv) are enhanced. These morphological changes improve phosphate uptake by because there is no alternative source of phosphate we must either increasing the root absorption surface and by favoring foraging of topsoil, which is recycle or improve the efficiency of our phosphorus usage [104,105]. where phosphate accumulates. Adapting crop root phenotypes to low phosphate This explains the strategic maneuvering by a few countries such as conditions would greatly improve phosphate use efficiency and limit the use of the USA to secure their future phosphate supply. fertilizers, reducing costs and limiting environmental pollution. Massive use of phosphate fertilizers is not only a considerable expense for farmers but also an ecological risk because the The first visible event upon Pi starvation is a strong remaining soil phosphorus is a major contributing factor to eutrophication of lakes and rivers by run-off, leaching and soil reduction of primary root cell elongation, which occurs erosion [106,107]. Inappropriate management of phosphate fertilizer rapidly after transfer to low Pi medium, followed by a has resulted in serious water pollution and substantial waste of reduction of cell division as reported by the cell cycle phosphorus in China [108,109]. Also, livestock wastes contain large marker CYCB1;1 (CYCLIN B1;1). This is accompanied amount of phosphorus that adds up to pollute waterways. A further by a loss of quiescent center (QC) identity as reported by source is the phosphorus used in detergents that is released in household wastewater. The resulting eutrophication of rivers the QC46 marker. Interestingly, cell division activity and the development of bacteria impacts water quality, thus is maintained in the lpi1 and lpi2 (low phosphorus insen- affecting fishing, fish farms, swimming areas and drinking water sitive) mutants. The activity of the root apical meri- associated with potential massive economic losses. stem (RAM) has been proposed to determine the magnitude of Pi starvation responses. Enhancing RAM acquire different strategies to cope with nutrient limita- activity by external sucrose application enhances the star- tions, which suggests that these responses are genetically vation responses as reported by the expression of Pi star- controlled. In the case of phosphate deprivation, a strong vation markers (the high-affinity phosphate transporter inhibition of primary root growth is observed in the Ara- PHT1;1, the monogalactosyl-diacylglycerol synthase bidopsis Columbia accession (Col-0). However, detailed MGD3 and the UDP-sulfoquinovose synthase SQD1). Re- characterization of natural variation has demonstrated spectively, cytokinin treatment or osmotic stress, which the existence of contrasting strategies in response to low reduce RAM activity, result in a reduction of Pi starvation phosphate. Among 73 Arabidopsis ecotypes, half responses. However, enhanced root growth is likely to showed a reduced primary root growth on low Pi, therefore result in lower relative Pi content through root mass suggesting that root growth inhibition is genetically deter- increase. The mechanism underlying the control of Pi mined rather than only metabolically controlled. The starvation by the RAM remains to be elucidated. identification of quantitative trait loci (QTL) controlling Several transcriptomics approaches have generated the root growth response to low Pi further demonstrates lists of genes that are induced or repressed during phos- the existence of such a control. Similarly, phosphite phate starvation [27–30]. These genes have been used as (Phi), which is the reduced and metabolically inert form of candidates for reverse genetics approaches and some have Pi (H2PO3–), can repress typical responses to Pi limitation proven successful. For instance, the AINTEGUMENTA-. This suggests that there is a perception mechanism like (AIL) gene PRD (PHOSPHATE ROOT DEVELOP- for Pi that can be triggered by Phi. The major goal of MENT) is rapidly repressed in roots under low Pi condi- research on phosphate response in plants is to identify tions. Analysis of the null allele mutant prd showed a mild and understand this perception–response pathway. hypersensitivity to Pi starvation. Interestingly, genes 443 Review Trends in Plant Science August 2011, Vol. 16, No. 8 Table 1. Overview of mutants with altered root architectural response to phosphate starvation AGI Mutant name Function and mutant root phenotype Refs Primary root growth inhibition Unknown lpi1, lpi2, lpi3 Unknown function Primary root growth maintained in low Pi At1g79700 prd DNA binding protein Hypersensitivity to low Pi At1g23010 lpr1, lpr2 Multicopper oxidase At1g71040 Primary root growth maintained in low Pi At5g23630 pdr2 P5-type ATPase Hypersensitivity to low Pi At4g28610 phr1 MYB family transcription factor Slight reduction in the root-to-shoot ratio At5g60410 siz1 SUMO E3 ligase Hypersensitivity to low Pi Lateral root formation and elongation At5g60410 siz1 SUMO E3 ligase Increase of LR number on low Pi At3g03710 pnp Ribonuclease polynucleotide phosphorylase Highly branched LR on low Pi At5g23630 pdr2 A P5-type ATPase Highly branched LR on low Pi Root hair proliferation At3g25710 bhlh32 Transcription factor that can interact with TTG1 and GL3 Increase of root hair formation in low Pi At5g21040 fbx2 F-Box protein with WD40 domain Increase of root hair formation growth in low Pi At5g42810 ipk1 Inositol polyphosphate kinase Constitutive production of root hairs At3g20630 ubp14/per1 Ubiquitin protease Inhibition of root hair growth in low Pi At5g03730 hsp2 Raf like kinase, negative regulator of ethylene response Increase of root hair formation in low Pi At4g02780 ga1-3 Gibberellin biosynthesis Reduction in root hair length in low Pi At5g29000/At4g28610 phl1/phr1 MYB family transcription factor Root hair length affected in low Pi At5g60410 siz1 SUMO E3 ligase Higher root hair number in low Pi At5g13080 wrky75 Transcription factor Constitutive production of root hairs At1g27740 rsl4 Transcription factor Development of very short root hairs Abbreviations: AGI, Arabidopsis gene identifier; LR, lateral roots; Pi, phosphate. from the AIL family are expressed in actively growing and (MCO) in the Pi response. The mechanisms underlying developing tissue where they can specify meristematic or MCO function are still under investigation and have yet to division-competent states. In the context of Pi starva- be described. Interestingly, the LPR1 QTL can be tion, PRD repression could therefore mediate primary root explained by the differential expression of the LPR1 gene growth arrest. However, the absence of effect of the prd in the root tip, and contact of the root tip with Pi-depleted mutation on rich medium suggests either functional re- medium is necessary to reprogram RSA. These obser- dundancy or that the mode of action of PRD might be more vations demonstrate the importance of the root tip by complex. Further studies are necessary to understand this suggesting it could act as a sensor for local Pi levels. The mechanism but a role for PRD in reducing meristem facultative ability of Arabidopsis to use organophosphates activity would be compatible with the shift from an inde- such as nucleic acids in Pi-limiting conditions enabled the terminate to a determinate developmental program in the phosphate starvation mutant pdr2 ( phosphate deficiency primary root induced by low Pi. response 2) to be isolated. PDR2 is a P5-type ATPase Screening for mutant seedlings with maintained prima- that maintains SCR (SCARECROW) expression in low Pi, ry root growth on low Pi has helped to identify genes which is a key regulator of root patterning and, potentially involved in this pathway. These approaches therefore, affects SHR (SHORT-ROOT) movement towards led to the identification of the lpi mutant series but the the endodermis. Interestingly, PDR2 co-localizes with corresponding genes have not yet been characterized. LPR1 in the endoplasmic reticulum (ER) at the root tip, However, the study of a root QTL led to the molecular which could indicate that PDR2 and LPR1 function togeth- characterization of the lpr mutants (low phosphate root 1 er in an ER-resident pathway that adjusts root meristem and lpr2) and demonstrated a role for multicopper oxidases activity to external Pi. 444 Review Trends in Plant Science August 2011, Vol. 16, No. 8 Screening for plants with deregulation of the low Pi- accumulation, as would be expected from iron toxicity, but induced IPS1 (INDUCED BY PI STARVATION 1) reporter rather a relocalization of ROS production. Further studies gene led to the identification of the phr1 ( phosphate are needed to understand how the genetic crosstalk be- starvation response 1) mutant. PHR1 is a transcription tween iron and Pi is integrated to control plant develop- factor belonging to the MYB family that binds to P1BS mental responses to changes in its nutrient environment. sites (PHR1 BINDING SITES) in the promoter of most genes positively or negatively affected by Pi starvation Lateral root formation and elongation. Despite its central role in controlling the expression Concomitantly with primary root growth inhibition, lateral of numerous Pi-deficiency-related genes, the phr1 mutant root formation and growth is enhanced by Pi starvation. shows no major root developmental defects apart from a However, the link between the two developmental slight reduction in the root-to-shoot ratio and root hair responses is not clearly understood. Given the major role induction. The activity of the PHR1 transcription of auxin during lateral root development , the role of factor is controlled at the post-translational level by this plant hormone has been studied in response to low Pi SIZ1 [SAP (scaffold attachment factor, acinus, protein perception. Auxin fluxes are altered by primary root inhibitor of activated signal transducer and activator of growth arrest and, as a result, more auxin could be made transcription) and MIZ1 (MSX2-interacting zinc finger)] available to induce lateral root formation. Such a mecha- through SUMOylation. SUMOylation is the reversible nism has been proposed but no evidence has backed up conjugation of the small ubiquitin-like modifier (SUMO) this hypothesis. Instead, the existence of distinct mecha- peptide to protein substrates, which acts as a major post- nisms governing primary root growth arrest and increased translational regulatory process in eukaryotes. The lateral root formation can be anticipated based on the SUMO E3 ligase SIZ1 plays an important role in Pi defi- observation that the primary root of the lpi3 mutant is ciency responses. The siz1 mutant has a hypersensitive only slightly reduced on low phosphate but still produces phenotype in response to low Pi, showing a shorter primary more lateral roots. Studies focusing on auxin-induced root. The fact that the siz1 phenotype is more affected genes demonstrated an increased sensitivity to auxin in than phr1 suggests that SIZ1 has other targets beyond low Pi conditions and similar observations were made PHR1 and/or that the PHR family undergoes functional using the synthetic auxin-responsive promoter DR5 redundancy. The PHL (PHR1-LIKE) MYB transcrip- [55,56,58]. Interestingly, expression of TIR1 (TRANS- tion factors are therefore likely candidates as targets of PORT INHIBITOR RESPONSE 1), the major component SIZ1 in the control of root developmental responses to low of the auxin perception complex SCFTIR1/AuxIAA , is Pi. However, SIZ1 does not appear to be specific to Pi increased on low Pi. As a result, the auxin sensitivity of starvation because the loss-of-function siz1 mutants are Pi-depleted roots is increased even if the overall auxin altered in flowering time, immunity, thermotolerance and content is not altered. An increase in auxin sensitivity drought tolerance. is directly correlated with more lateral root initiation and A role for iron in controlling primary root growth re- emergence. Further studies are needed to understand the sponse to low Pi has been demonstrated. The presence mechanism underlying the induction of TIR1 by the Pi of iron in the medium, even at concentrations as low as transduction pathway. Interestingly, SIZ1 negatively reg- 10 mM, is required for primary root growth inhibition. This ulates lateral root formation on low Pi as demonstrated by observation suggests that iron potentiates the Pi response the increased lateral root response of the siz1 mutant to of the primary root. The interaction between iron and Pi starvation. The upregulation of several auxin-induced has long been known both in soils, where the two molecules genes in the siz1 mutant suggests a link between the bind and often precipitate, and also in planta, where they SUMO E3 ligase and the auxin pathway. However, TIR1 can be found in the form of massive protein complexes seems unlikely to be a direct target of SIZ1 because it lacks called ferritins [43,44]. Interestingly, the ferritin gene a SUMOylation motif , but such a motif is present in AtFER1 is induced by Pi starvation , most probably the transcription factor ARF7 (AUXIN RESPONSE FAC- as a result of increased iron availability in the low Pi TOR 7), which is a key regulator of lateral root formation medium. It has been proposed that the primary root. Nevertheless, the role of ARF7 in the Pi response is growth inhibition observed on low Pi is caused by a toxic unclear because the arf7 mutant still produces more later- effect of iron. However, the evidence for iron-related al roots in response to deficiency. Further description toxicity in low Pi conditions is still lacking. It is also of the link between the Pi response pathway and auxin unclear how iron toxicity could be responsible for primary signaling would be of major interest to understand how root growth inhibition without affecting lateral root increased lateral root formation is achieved on low Pi. growth. Several studies indicate that iron excess, or muta- The chloroplastic PNPase gene encodes a ribonuclease tions leading to an abnormally high level of iron, lead to an polynucleotide phosphorylase that is involved in RNA increase in reactive oxygen species (ROS) production [47– catabolism in plants and bacteria. In the green alga 50]. However, recent reports show that Pi deficiency is Chlamydomonas reinhardtii, reduced expression of the accompanied by a diminution of ROS accumulation in the cpPNPase renders cells unable to acclimate to Pi depriva- elongation zone and the root tip. ROS production tion. In Arabidopsis, the pnp mutant is characterized in the elongation zone on high Pi medium is considered to by decreased auxin responsiveness and cell division in the be an important factor accelerating root growth. These lateral root, and exhibits cell death at the lateral root tips results suggest that the mechanism responsible for prima-. Similarly, lateral root elongation in low Pi is con- ry root growth inhibition on low Pi does not involve ROS trolled by PDR2 as demonstrated by the altered expression 445 Review Trends in Plant Science August 2011, Vol. 16, No. 8 of the CYCB1;1 cell division marker in the lateral root of important role in signal transduction in both plant and the pdr2 mutant. Both PDR2 and the PNPase are animal cells by phosphorylating inositol trisphosphate involved in Pi sensing and signaling, as suggested by the (IP3) to inositol tetrakisphosphate (IP4). Both IP3 and constitutive expression of Pi responsive genes in their IP4 are crucial second messengers that regulate calcium corresponding mutants [35,65]. Interestingly, both pdr2 homeostasis. Therefore, it is likely that IP3 and IP4 are and pnp mutants produce numerous, short, highly involved in Pi signal transduction. branched lateral roots on low Pi. These structures strik- A role for UBP14 (UBIQUITIN SPECIFIC PROTEASE ingly resemble the so-called ‘cluster roots’, which are pro- 14) in root hair development has been reported. UBP14 duced by plants of several different families in response to cleaves ubiquitin from polypeptides with broad substrate iron and Pi deficiency and are associated with intense specificity. The loss-of-function ubp14 mutant is em- mobilization of nutrients [14–17]. Studying the role of bryo lethal but a recent study identified a novel mutant PDR2 and PNPase orthologs in cluster-root-forming plants allele per1 (Pi deficient root hair defective 1) without em- would therefore be of great interest. bryo defects. The per1 mutation probably results from a reduction in UBP14 translation efficiency owing to a Root hair proliferation change in codon usage. As a result, the per1 mutant Deficiency of several nutrients, including iron, zinc, man- hampers root hair growth when seedlings are Pi-starved ganese and P, stimulates root hair production; however, in. This report suggests that removal of ubiquitin moie- Arabidopsis, P has a greater effect than other nutrients on ties from protein substrates is important for root hair root hair density and length [66–68]. Root hair density induction on low Pi. Future studies should focus on identi- increases logarithmically with decreasing P availability in fying such targets. the medium : this massive increase in root hair pro- Several reports indicate that ethylene is involved in Pi duction on low Pi improves the overall root absorption starvation-mediated root hair induction [80–83]. A large- capacity, and the absorption efficiency of root hairs is also scale screen for Arabidopsis mutants with altered Pi-star- enhanced [69,70]. Root hair density is further increased by vation responses resulted in the identification of hsp2 (hy- the reduction of epidermal cell length on low Pi. Recent persensitive to phosphate starvation 2), a new allele of the studies have described how Pi starvation affects root hair ethylene pathway intermediate CTR1 (CONSTITUTIVE cell differentiation and pinpoint the role of ethylene and TRIPLE RESPONSE 1). Loss-of-function ctr1 mutants gibberellins. exhibit constitutive ethylene responses [85,86]. The hsp2 Root epidermal cells differentiate in a position-depen- mutant shows hypersensitivity to phosphate starvation, dent manner, with hair cells overlying two cortical cell files resulting in constitutive expression of Pi starvation-induced and non-hair cells overlying a single cortical file. It has genes, induction of acid phosphatases, production of antho- been shown that Pi starvation leads to ectopic development cyanins and increased root hair density. These results of hairs in the non-hair position. The specification of provide evidence that ethylene signaling is linked to the Pi non-hair versus hair cell fate depends on several factors, starvation pathway, this is further supported by transcrip- including TTG1 (TRANSPARENT TESTA GLABRA1) and tomic analysis revealing that ethylene synthesis and re- GL3 (GLABRA3). A novel transcription factor sponse genes are induced locally in response to Pi starvation (BHLH32) that acts as a negative regulator of a range of. However, further studies are needed to understand the biochemical and morphological processes induced in Pi mechanisms underlying this crosstalk. starvation has been identified. Root hair formation in A role for gibberellins (GAs) in the regulation of root hair the bhlh32 mutant is constitutive, which means that unlike length has been demonstrated. GA response is mediat- wild type, it is not suppressed by growth in the presence of ed by transcription factors containing the DELLA peptidic high levels of Pi. Furthermore, it has been demonstrated motif and that act as negative regulators of the GA response. that BHLH32 interacts in vitro with TTG1 and GL3. How- DELLAs have been found to play important roles in many ever, BHLH32 is not apparently required for the induction of aspects of the adaptation of plant growth and development root hair production. Thus, it is possible that in root in response to environmental variables. The GA-defi- epidermal cells BHLH32 might interact with TTG1 and GL3 ciency ga1-3 mutation confers a significant reduction in root to control root hair formation. An F-box protein able to hair length in Pi-starved plants, which can be restored by interact in vitro with BHLH32 has been identified. exogenous GA application. Therefore, an appropriate bioac- FBX2 encodes a protein containing both WD40 and F-box tive GA level is necessary for the increased root hair growth motifs and is a negative regulator of root hair growth. When that is characteristic of Pi-starved roots. In Pi-starvation grown in Pi-sufficient conditions, fbx2 contains significantly conditions, genes that activate bioactive GAs are repressed, more total Pi and more anthocyanins than the wild type and whereas those that de-activate GAs are induced. As a con- exhibits significant root hair growth. BHLH32 and sequence, Pi-starvation causes a reduction in bioactive GA FBX2 are probably not part of the Pi starvation sensing level, which in turn causes DELLA accumulation. Accumu- and signaling pathways per se; however, we can speculate lation of DELLAs in turn contributes to a range of charac- that they are downstream targets of the Pi starvation teristic Pi-starvation responses. The GA–DELLA system pathway. Finding the missing links between the two path- regulates the increased root hair length, which is character- ways would be of great interest. Mutants defective in IPK1 istic of Pi-starvation. (INOSITOL POLYPHOSPHATE KINASE) constitutively Several transcription factors have been shown to control produce root hairs in high Pi conditions, suggesting root hair growth during phosphate deficiency. For in- they are altered in sensing external Pi. IPKs play an stance, root hair length is strongly affected in the phr1 446 Review Trends in Plant Science August 2011, Vol. 16, No. 8 phl1 double mutant in response to phosphate starvation a new hope for a future new green revolution. In this. By contrast, a mutation in the PHR1 regulator SIZ1 review, we focused on the developmental adaptation to Pi enhances sensitivity of Arabidopsis plants to Pi depriva- deficiency and demonstrated that tremendous progress tion, resulting in a greater number of root hairs. In the has been made in understanding how Arabidopsis can cope large WRKY transcription factor family, WRKY75 is the with Pi limitation. Interestingly, most higher plants share first member reported to be involved in regulating a nutri- similar developmental responses that aim at developing a ent starvation response and root development. When shallow and proliferated root system that is efficient in WRKY75 expression was suppressed, root hair number uptake of soil Pi. The topsoil foraging strategy has been was significantly increased. However, changes in the root revealed by studies on common bean (Phaseolus vulgaris) architecture were observed under both Pi-sufficient and Pi-. Also, despite major differences between the root deficient conditions. Thus, WRKY75 is a negative system of Arabidopsis and cereals such as rice (Oryza modulator of Pi starvation responses as well as root devel- sativa) or maize (Zea mays) , numerous regulators opment. The loss-of-function of the RSL4 transcription are conserved between these species [94,95]. For instance, factor resulted in the development of very short root hairs overexpression of AtPHR1 rice orthologs leads to increased and constitutive RSL4 expression induced constitutive adventitious root length and root hair growth. How- growth, resulting in the formation of very long root hairs ever, some discrepancy exists in the cereal root develop-. Therefore, RSL4 is required for the increase in root mental responses. In response to Pi starvation, primary hair growth. Interestingly, it was found that low phosphate root growth is enhanced in rice but this response is still stress controls root hair length by modulating steady-state under the control of the PHR orthologs [94,96]. Therefore, levels of RSL4 transcript and protein, and requires the despite differences in the adaptive response of monocots phosphate signaling activity of LPR1 and LPR2. This and dicots, it is highly probable that the key regulators and observation suggests that LPR1 might interact with down- sensing mechanisms will be conserved and that transfer- stream components of the Pi sensing pathway. ring of Arabidopsis research to crop species will be infor- mative. Towards an integrated view of phosphate deficiency One key step in the understanding of Pi sensing by responses plants will be the identification of its perception machin- Scientists and agronomists share a growing interest in ery. The eukaryotic plasma membrane is the site of choice understanding plant root systems because they represent for sensing nutrients and nutrient-sensing proteins can be [(Figure_2)TD$IG] Phosphate starvation Pi sensing Local response Systemic response Root system Phosphate architecture homeostasis Pi Pi Primary root growth Pi recycling inhibition Pi Pi Lateral root Pi recovery formation and growth Root hair Pi transport formation TRENDS in Plant Science Figure 2. Local and systemic responses. Sensing of phosphate (Pi) by the model plant Arabidopsis triggers a set of adaptive responses that can be grouped into local and systemic (long distance) responses. This review focuses on developmental adaptations that are mainly controlled at the local level. However, systemic responses involve increased Pi transport through expression of high-affinity transporters, intense Pi recovery through secretion of phosphatases and Pi recycling through catabolism of phospholipids. Altogether, Pi starvation results in a shift in the developmental program to increase the fitness of the plant. A better understanding of these mechanisms should improve our capacity to enhance plants to tolerate low Pi conditions. 447 Review Trends in Plant Science August 2011, Vol. 16, No. 8 grouped into three classes: G-protein coupled receptors, 4 Schachtman, D.P. et al. (1998) Phosphorus uptake by plants: from soil to cell. Plant Physiol. 116, 447–453 nutrient transporters with an additional receptor function 5 Hinsinger, P. (2001) Bioavailability of soil inorganic P in the and transporter homologues with a nutrient receptor func- rhizosphere as affected by root-induced chemical changes: a review. tion and no transport capacity. Recently, a mechanism Plant and Soil 237, 173–195 involved in Arabidopsis nitrate sensing has been elucidat- 6 Ticconi, C.A. and Abel, S. (2004) Short on phosphate: plant ed, demonstrating that the high-affinity nitrate transport- surveillance and countermeasures. Trends Plant Sci. 9, 548–555 7 Desnos, T. (2008) Root branching responses to phosphate and nitrate. er NRT1.1 acts as a sensor and further evidence Curr. Opin. Plant Biol. 11, 82–87 suggests that other nitrate transporters might also act 8 Lin, W.Y. et al. (2009) Molecular regulators of phosphate homeostasis as transceptors. In yeast (Saccharomyces cerevisiae), in plants. J. Exp. Bot. 60, 1427–1438 recent studies have dissected the mechanism underlying 9 Rouached, H. et al. (2010) Regulation of phosphate starvation Pi perception by the phosphate transporter Pho84. Signal- responses in plants: signaling players and cross-talks. Mol. Plant 3, 288–299 ing by Pho84 does not require a complete transport cycle 10 Fitter, A. et al. (2002) Root system architecture determines fitness in but a specific conformational change. These studies an Arabidopsis mutant in competition for immobile phosphate ions have paved the way for the future molecular and functional but not for nitrate ions. Proc. Biol. Sci. 269, 2017–2022 characterization of the Pi perception machinery in Arabi- 11 Lynch, J. and Brown, M. (2001) Topsoil foraging – an architectural dopsis. adaptation of plants to low phosphorus availability. Plant and Soil 237, 225–237 Importantly, these findings must be integrated at the 12 Péret, B. et al. (2009) When plants socialize: symbioses and root whole plant level to fully comprehend such an adaptive development. Annu. Plant Rev. 37, 209–238 mechanism. Recent results show that Pi starvation 13 Smith, S.E. and Smith, F.A. (2011) Roles of arbuscular mycorrhizas in responses can be separated into two groups that are dis- plant nutrition and growth: new paradigms from cellular to ecosystem scales. Annu. Rev. Plant Biol. 62, 16.1–16.24 tinct. The developmental adaptations are locally reg- 14 Lambers, H. and Shane, M.W. (2007) Role of root clusters in ulated, whereas the effects on Pi homeostasis are regulated phosphorus acquisition and increasing biological diversity in at the systemic (long distance) level (Figure 2). However, agriculture. Wageningen UR Frontis Series 21, 235 there is likely to be crosstalks between the two pathways. 15 Lamont, B.B. (2003) Structure, ecology and physiology of root The regulation of Pi homeostasis has been extensively clusters–a review. Plant and Soil 248, 1–19 16 Neumann, G. and Martinoia, E. (2002) Cluster roots–an underground described in several reviews [6,8,9]. All these reports tend adaptation for survival in extreme environments. Trends Plant Sci. 7, to show that lack of Pi triggers a shift in the physiological 162–167 status and developmental program to increase the fitness 17 Shane, M.W. and Lambers, H. (2005) Cluster roots: a curiosity in of the plant. context. Plant and Soil 274, 101–125 As demonstrated in this review, Arabidopsis is the tool 18 Ericsson, T. (1995) Growth and shoot: root ratio of seedlings in relation to nutrient availability. Plant and Soil 168, 205–214 of choice to increase our understanding of Pi perception 19 López-Bucio, J. et al. (2003) The role of nutrient availability in and response in terms of root developmental responses. regulating root architecture. Curr. Opin. Plant Biol. 6, 280–287 However, developmental adaptations of the model plant to 20 Forde, B. and Lorenzo, H. (2001) The nutritional control of root Pi starvation are limited because of its inability to enter development. Plant and Soil 232, 51–68 into symbioses with fungi or to produce cluster roots 21 Reymond, M. et al. (2006) Identification of QTL controlling root growth response to phosphate starvation in Arabidopsis thaliana.. Therefore, there is much to gain in translating Ara- Plant Cell Environ. 29, 115–125 bidopsis research to plants that have these abilities. For 22 Chevalier, F. et al. (2003) Effects of phosphate availability on the instance, lupin (Lupinus spp.) appears to be a useful root system architecture: large-scale analysis of the natural system because of its ability to form both cluster roots variation between Arabidopsis accessions. Plant, Cell Environ. 26, 1839–1850 and nitrogen-fixing symbioses. In addition, several studies 23 Ticconi, C.A. et al. (2001) Attenuation of phosphate starvation have demonstrated the establishment of rice as a model responses by phosphite in Arabidopsis. Plant Physiol. 127, 963–972 monocot, including in the field of Pi perception and 24 Sánchez-Calderón, L. et al. (2005) Phosphate starvation induces a responses [94,95]. As a consequence, there is much to gain determinate developmental program in the roots of Arabidopsis in taking advantage of new approaches such as chemical thaliana. Plant Cell Physiol. 46, 174–184 25 Sánchez-Calderón, L. et al. (2006) Characterization of low phosphorus genetics that aim to identify new drugs that can easily be insensitive mutants reveals a crosstalk between low phosphorus- applied to several species. induced determinate root development and the activation of genes involved in the adaptation of Arabidopsis to phosphorus deficiency. Acknowledgements Plant Physiol. 140, 879–889 This work was supported by an EMBO Long-Term Fellowship (ALTF 26 Lai, F. et al. (2007) Cell division activity determines the magnitude of 503-2010) to B.P., a grant from ANR-KBBE (FOSSI) to M.C. and from phosphate starvation responses in Arabidopsis. Plant J. 50, 545–556 ANR-Blanc (CHEMIGENA) to T.D. We are grateful to Malcolm Bennett 27 Misson, J. et al. (2005) A genome-wide transcriptional analysis using for critical reading of the manuscript. We thank the reviewers for their Arabidopsis thaliana Affymetrix gene chips determined plant helpful feedback. responses to phosphate deprivation. Proc. Natl. Acad. Sci. U.S.A. The authors declare that they have no conflict of interest. 102, 11934–11939 28 Morcuende, R. et al. (2007) Genome-wide reprogramming of References metabolism and regulatory networks of Arabidopsis in response to 1 Cordell, D. et al. (2009) The story of phosphorus: global food security phosphorus. Plant Cell Environ. 30, 85–112 and food for thought. Global Environ. Change 19, 292–305 29 Thibaud, M.C. et al. (2010) Dissection of local and systemic 2 Steen, I. (1998) Phosphorus availability in the 21st century: transcriptional responses to phosphate starvation in Arabidopsis. management of a non-renewable resource. Phosphorus and Plant J. 64, 775–789 Potassium 217, 25–31 30 Wu, P. et al. (2003) Phosphate starvation triggers distinct alterations 3 Bieleski, R.L. (1973) Phosphate pools, phosphate transport, and of genome expression in Arabidopsis roots and leaves. Plant Physiol. phosphate availability. Annu. Rev. Plant Physiol. 24, 225–252 132, 1260–1271 448 Review Trends in Plant Science August 2011, Vol. 16, No. 8 31 Camacho-Cristóbal, J.J. et al. (2008) PRD, an Arabidopsis 57 Al-Ghazi, Y. et al. (2003) Temporal responses of Arabidopsis root AINTEGUMENTA-like gene, is involved in root architectural architecture to phosphate starvation: evidence for the involvement of changes in response to phosphate starvation. Planta 228, 511–522 auxin signalling. Plant, Cell & Environ. 26, 1053–1066 32 Nole-Wilson, S. et al. (2005) AINTEGUMENTA-like (AIL) genes are 58 Jain, A. et al. (2007) Differential effects of sucrose and auxin on expressed in young tissues and may specify meristematic or division- localized phosphate deficiency-induced modulation of different competent states. Plant Mol. Biol. 57, 613–628 traits of root system architecture in Arabidopsis. Plant Physiol. 33 Svistoonoff, S. et al. (2007) Root tip contact with low-phosphate media 144, 232–247 reprograms plant root architecture. Nat. Genet. 39, 792–796 59 Mockaitis, K. and Estelle, M. (2008) Auxin receptors and plant 34 Arnaud, C. et al. (2010) The root cap at the forefront. C. R. Biol. 333, development: a new signaling paradigm. Annu. Rev. Cell Dev. Biol. 335–343 24, 55–80 35 Ticconi, C.A. et al. (2004) Arabidopsis pdr2 reveals a phosphate- 60 Miura, K. et al. (2011) SIZ1 Regulation of phosphate starvation- sensitive checkpoint in root development. Plant J. 37, 801–814 induced root architecture remodeling involves the control of auxin 36 Gallagher, K.L. et al. (2004) Mechanisms regulating SHORT-ROOT accumulation. Plant Physiol. 155, 1000–1012 intercellular movement. Curr. Biol. 14, 1847–1851 61 Geiss-Friedlander, R. and Melchior, F. (2007) Concepts in 37 Ticconi, C.A. et al. (2009) ER-resident proteins PDR2 and LPR1 mediate sumoylation: a decade on. Nat. Rev. Mol. Cell Biol. 8, 947–956 the developmental response of root meristems to phosphate 62 Okushima, Y. et al. (2005) Functional genomic analysis of the AUXIN availability. Proc. Natl. Acad. Sci. U.S.A. 106, 14174–14179 RESPONSE FACTOR gene family members in Arabidopsis thaliana: 38 Martı́n, A.C. et al. (2000) Influence of cytokinins on the expression of unique and overlapping functions of ARF7 and ARF19. Plant Cell 17, phosphate starvation responsive genes in Arabidopsis. Plant J. 24, 444–463 559–567 63 Baginsky, S. et al. (2001) Chloroplast PNPase exists as a homo- 39 Rubio, V. et al. (2001) A conserved MYB transcription factor involved multimer enzyme complex that is distinct from the Escherichia coli in phosphate starvation signaling both in vascular plants and in degradosome. RNA 7, 1464–1475 unicellular algae. Genes Dev. 15, 2122–2133 64 Yehudai-Resheff, S. et al. (2007) Integration of chloroplast nucleic acid 40 Bustos, R. et al. (2010) A central regulatory system largely controls metabolism into the phosphate deprivation response in transcriptional activation and repression responses to phosphate Chlamydomonas reinhardtii. Plant Cell 19, 1023–1038 starvation in Arabidopsis. PLoS Genet. 6, 9 65 Marchive, C. et al. (2009) Abnormal physiological and molecular 41 Miura, K. et al. (2007) Sumoylation, a post-translational regulatory mutant phenotypes link chloroplast polynucleotide phosphorylase process in plants. Curr. Opin. Plant Biol. 10, 495–502 to the phosphorus deprivation response in Arabidopsis. Plant 42 Miura, K. et al. (2005) The Arabidopsis SUMO E3 ligase SIZ1 controls Physiol. 151, 905–924 phosphate deficiency responses. Proc. Natl. Acad. Sci. U.S.A. 102, 66 Bates, T.R. and Lynch, J.P. (1996) Stimulation of root hair elongation 7760–7765 in Arabidopsis thaliana by low phosphorus availability. Plant, Cell & 43 Briat, J.F. et al. (2010) New insights into ferritin synthesis and Environ. 19, 529–538 function highlight a link between iron homeostasis and oxidative 67 Ma, Z. et al. (2001) Regulation of root hair density by phosphorus stress in plants. Ann. Bot. 105, 811–822 availability in Arabidopsis thaliana. Plant, Cell & Environ. 24, 459–467 44 Briat, J.F. et al. (2010) Ferritins and iron storage in plants. 68 Schmidt, W. and Schikora, A. (2001) Different pathways are involved Biochimica et Biophysica Acta (BBA)-General Subjects 1800, 806–814 in phosphate and iron stress-induced alterations of root epidermal cell 45 Hirsch, J. et al. (2006) Phosphate deficiency promotes modification of development. Plant Physiol. 125, 2078–2084 iron distribution in Arabidopsis plants. Biochimie 88, 1767–1771 69 Bates, T.R. and Lynch, J.P. (2000) The efficiency of Arabidopsis 46 Ward, J.T. et al. (2008) The effect of iron on the primary root thaliana (Brassicaceae) root hairs in phosphorus acquisition. Am. elongation of Arabidopsis during phosphate deficiency. Plant J. Bot. 87, 964–970 Physiol. 147, 1181–1191 70 Gilroy, S. and Jones, D.L. (2000) Through form to function: root hair 47 Caro, A. and Puntarulo, S. (1996) Effect of in vivo iron development and nutrient uptake. Trends Plant Sci. 5, 56–60 supplementation on oxygen radical production by soybean roots. 71 Schiefelbein, J.W. (2000) Constructing a plant cell. The genetic control Biochim. Biophys. Acta 1291, 245–251 of root hair development. Plant Physiol. 124, 1525–1531 48 Kampfenkel, K. et al. (1995) Effects of iron excess on Nicotiana 72 Müller, M. and Schmidt, W. (2004) Environmentally induced plumbaginifolia plants (implications to oxidative stress). Plant plasticity of root hair development in Arabidopsis. Plant Physiol. Physiol. 107, 725–735 134, 409–419 49 Pekker, I. et al. (2002) Reactive oxygen intermediates and glutathione 73 Ishida, T. et al. (2008) A genetic regulatory network in the regulate the expression of cytosolic ascorbate peroxidase during iron- development of trichomes and root hairs. Annu. Rev. Plant Biol. mediated oxidative stress in bean. Plant Mol. Biol. 49, 429–438 59, 365–386 50 Pich, A. et al. (1994) Iron-dependent changes of heavy metals, 74 Chen, Z.H. et al. (2007) BHLH32 modulates several biochemical and nicotianamine, and citrate in different plant organs and in the morphological processes that respond to Pi starvation in Arabidopsis. xylem exudate of two tomato genotypes. Nicotianamine as possible Biochem. J. 405, 191–198 copper translocator. Plant and Soil 165, 189–196 75 Chen, Z. et al. (2008) Identification of an F-Box protein that negatively 51 Tyburski, J. et al. (2009) Reactive oxygen species localization in roots regulates Pi starvation responses. Plant Cell Physiol. 49, 1902–1906 of Arabidopsis thaliana seedlings grown under phosphate deficiency. 76 Stevenson-Paulik, J. et al. (2005) Generation of phytate-free seeds in Plant Growth Regul. 59, 27–36 Arabidopsis through disruption of inositol polyphosphate kinases. 52 Chacón-López, A. et al. (2011) Global expression pattern comparison Proc. Natl. Acad. Sci. U.S.A. 102, 12612–12617 between low phosphorus insensitive 4 and WT Arabidopsis reveals an 77 Xia, H.J. and Yang, G. (2005) Inositol 1,4,5-trisphosphate 3-kinases: important role of reactive oxygen species and jasmonic acid in the root functions and regulations. Cell Res. 15, 83–91 tip response to phosphate starvation. Plant Signal. Behav. 6, 382–392 78 Doelling, J.H. et al. (2001) The ubiquitin-specific protease UBP14 is 53 Liszkay, A. et al. (2003) Evidence for the involvement of cell wall essential for early embryo development in Arabidopsis thaliana. peroxidase in the generation of hydroxyl radicals mediating extension Plant J. 27, 393–405 growth. Planta 217, 658–667 79 Li, W.F. et al. (2010) Ubiquitin-specific protease 14 (UBP14) is 54 Péret, B. et al. (2009) Arabidopsis lateral root development: an involved in root responses to phosphate deficiency in Arabidopsis. emerging story. Trends Plant Sci. 14, 399–408 Mol. Plant 3, 212–223 55 Nacry, P. et al. (2005) A role for auxin redistribution in the responses 80 He, Z. et al. (2005) Assessment of inequality of root hair density in of the root system architecture to phosphate starvation in Arabidopsis thaliana using the Gini coefficient: a close look at the Arabidopsis. Plant Physiol. 138, 2061–2074 effect of phosphorus and its interaction with ethylene. Ann. Bot. 56 Pérez-Torres, C.A. et al. (2008) Phosphate availability alters lateral 95, 287 root development in Arabidopsis by modulating auxin sensitivity via a 81 López-Bucio, J. et al. (2002) Phosphate availability alters architecture mechanism involving the TIR1 auxin receptor. Plant Cell 20, and causes changes in hormone sensitivity in the Arabidopsis root 3258–3272 system. Plant Physiol. 129, 244–256 449 Review Trends in Plant Science August 2011, Vol. 16, No. 8 82 Ma, Z. et al. (2003) Regulation of root elongation under phosphorus 96 Zhou, J. et al. (2008) OsPHR2 is involved in phosphate-starvation stress involves changes in ethylene responsiveness. Plant Physiol. signaling and excessive phosphate accumulation in shoots of plants. 131, 1381–1390 Plant Physiol. 146, 1673–1686 83 Zhang, Y.J. et al. (2003) Ethylene and phosphorus availability have 97 Gaxiola, R.A. et al. (2011) A transgenic approach to enhance interacting yet distinct effects on root hair development. J. Exp. Bot. phosphorus use efficiency in crops as part of a comprehensive 54, 2351–2361 strategy for sustainable agriculture. Chemosphere 84, 840–845 84 Lei, M. et al. (2011) Ethylene signalling is involved in regulation of 98 Holsbeeks, I. et al. (2004) The eukaryotic plasma membrane as a phosphate starvation-induced gene expression and production of acid nutrient-sensing device. Trends Biochem. Sci. 29, 556–564 phosphatases and anthocyanin in Arabidopsis. New Phytol. 189, 99 Ho, C.H. et al. (2009) CHL1 functions as a nitrate sensor in plants. Cell 1084–1095 138, 1184–1194 85 Stepanova, A.N. and Alonso, J.M. (2009) Ethylene signaling and 100 Gojon, A. et al. (2011) Nitrate transceptor(s) in plants. J. Exp. Bot. 62, response: where different regulatory modules meet. Curr. Opin. 2299–2308 Plant Biol. 12, 548–555 101 Popova, Y. et al. (2010) Transport and signaling through the 86 Lin, Z. et al. (2009) Recent advances in ethylene research. J. Exp. Bot. phosphate-binding site of the yeast Pho84 phosphate transceptor. 60, 3311–3336 Proc. Natl. Acad. Sci. U.S.A. 107, 2890–2895 87 Jiang, C. et al. (2007) Phosphate starvation root architecture and 102 Raghothama, K.G. (1999) Phosphate acquisition. Annu. Rev. Plant anthocyanin accumulation responses are modulated by the Physiol. Plant Mol. Biol. 50, 665–693 gibberellin-DELLA signaling pathway in Arabidopsis. Plant 103 Gilbert, N. (2009) Environment: the disappearing nutrient. Nature Physiol. 145, 1460–1470 461, 716–718 88 Achard, P. et al. (2006) Integration of plant responses 104 Ashley, K. et al. (2011) A brief history of phosphorus: From the to environmentally activated phytohormonal signals. Science 311, philosopher’s stone to nutrient recovery and reuse. Chemosphere 91–94 84, 737–746 89 Devaiah, B.N. et al. (2007) WRKY75 transcription factor is a 105 Cordell, D. (2009) 8 Reasons why we need to rethink the management modulator of phosphate acquisition and root development in of phosphorus resources in the global food system. Global Phosphorus Arabidopsis. Plant Physiol. 143, 1789–1801 Research Initiative 90 Yi, K. et al. (2010) A basic helix-loop-helix transcription factor controls 106 Hart, M.R. et al. (2004) Phosphorus runoff from agricultural land and cell growth and size in root hairs. Nat. Genet. 42, 264–267 direct fertilizer effects: A review. J. Environ. Qual. 33, 1954–1972 91 Herder, G.D. et al. (2010) The roots of a new green revolution. Trends 107 Smil, V. (2000) Phosphorus in the environment: natural flows and Plant Sci. 15, 600–607 human interferences. Annu. Rev. Energy Environ. 25, 53–88 92 Liao, H. et al. (2001) Effect of phosphorus availability on basal root 108 Qiu, J. and Qiu, J. (2010) Phosphate fertilizer warning for China. Nat. shallowness in common bean. Plant Soil 232, 69–79 News DOI: 10.1038/news.2010.498 93 Hochholdinger, F. et al. (2004) From weeds to crops: genetic analysis 109 Le, C. et al. (2010) Eutrophication of lake waters in China: cost, of root development in cereals. Trends Plant Sci. 9, 42–48 causes, and control. Environ. Manage. 45, 662–668 94 Panigrahy, M. et al. (2009) Molecular mechanisms in response to 110 Withers, P.J. et al. (2009) Characterization of phosphorus sources in phosphate starvation in rice. Biotechnol. Adv. 27, 389–397 rural watersheds. J. Environ. Qual. 38, 1998–2011 95 Yang, X.J. and Finnegan, P.M. (2010) Regulation of phosphate 111 Dodds, W.K. et al. (2009) Eutrophication of U.S. freshwaters: analysis starvation responses in higher plants. Ann. Bot. 105, 513–526 of potential economic damages. Environ. Sci. Technol. 43, 12–19 450