Membrane Receptors & Translocation PDF
Document Details
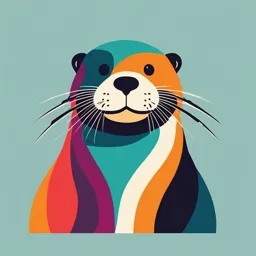
Uploaded by RichRing
Lali Shanshiashvili
Tags
Related
- 4BBY1030 Cell Biology and Neuroscience Lecture 14 - Cell Signalling - PDF
- WK 2- The Cell Building Blocks of Cell PDF
- Topic 2 - Intracellular Signalling Pathways PDF
- Lecture 6. Cell Membrane Structure PDF
- Cell Surface of the Extracellular Matrix PDF
- Building Blocks of Cells Part 2 Signal Transduction (Tyr Receptor) PDF 2024 Brunel University
Summary
This document provides an overview of membrane receptors and their role in cell signaling and transport systems. It explores the function of membrane proteins and the diversity of signaling mechanisms, including receptor tyrosine kinases and G-protein-coupled receptors. Diagrams illustrate concepts and tables outline key components.
Full Transcript
Membrane receptors. Translocation of molecules through membranes.. Membrane transport proteins. Membrane channels and pores Electrochemical-potential-driven transporters. Diseases due to loss of membrane transport systems. Primary active transporters. Cystic fibrosi...
Membrane receptors. Translocation of molecules through membranes.. Membrane transport proteins. Membrane channels and pores Electrochemical-potential-driven transporters. Diseases due to loss of membrane transport systems. Primary active transporters. Cystic fibrosis and the Cl- channel. Prof. Lali Shanshiashvili The plasma membrane is an envelope surrounding the cell ( It separates and protects the cell from the external hostile environment. Besides being a protective barrier, the plasma membrane provides a connecting system between the cell and its environment. The subcellular organelles such as the nucleus, mitochondria and lysosomes are also surrounded by membranes. The membrane is asymmetric due to the irregular distribution of proteins and lipids. The membranes are composed of lipids, proteins and carbohydrates. The actual composition differs from tissue to tissue. Among the lipids, amphipathic lipids (containing hydrophobic and hydrophilic groups) namely phospholipids, glycolipids and cholesterol, are found in the animal membranes. The structure of the membrane is considered as fluid mosaic. 1. Extrinsic (peripheral) membrane proteins are loosely held to the surface of the membrane and they can be easily separated e.g. cytochrome c of mitochondria. 2. Intrinsic (integral) membrane proteins are tightly bound to the lipid bilayer and they can be separated only by the use of detergents or organic solvents e.g. hormone receptors, cytochrome P450. Receptor proteins are either bound to plasma or intracellular membranes or are present as soluble proteins in the cytoplasm or nucleus. The molecules binding to a receptor, referred to as ligands, are a communication link, or signal. lntercellular signal molecules, that is, ligands generated by one cell to change the activity of another cell, react either with receptors on the plasma membrane or with intracellular receptors. Ligands such as charged molecules, peptides, and proteins cannot transverse the membrane because of their charge or size and react with plasma membrane receptors on the extracellular face of the membrane. Molecules that can diffuse through the plasma membrane, such as steroids, bind to intracellular soluble receptors. Intracellular signals can either bind to soluble receptors or ones on the cytoplasmic side of the membrane. The largest and most diverse group of membrane receptors is the G protein coupled receptors in both prokaryotic and eukaryotic cells. There are also plasma membrane receptors, such as the Toll-like receptors (TLR) that induce a response when bacteria or viruses bind; binding stimulates a host’s defence against the invading pathogens. G Protein–Coupled Receptors and Second Messengers G protein–coupled receptors (GPCRs) are receptors that act through a member of the guanosine nucleotide–binding protein, or G protein, family. Three essential components define signal transduction through GPCRs: a plasma membrane receptor with seven transmembrane helical segments, a G protein that cycles between active (GTP-bound) andinactive (GDP-bound) forms, and an effector enzyme (or ion channel) in the plasma membrane that is regulated by the activated G protein. An extracellular signal such as a hormone, growth factor, or neurotransmitter is the “first messenger” that activates a receptor from outside the cell. When the receptor is activated, its associated G protein exchanges its bound GDP for a GTP from the cytosol. The G protein then dissociates from the activated receptor and binds to the nearby effector enzyme, altering its activity. The effector enzyme then causes a change in the cytosolic concentration of a low molecular weight metabolite or inorganic ion, which acts as a second messenger to activate or inhibit one or more downstream targets, often protein kinases. Alfred G. Gilman and Martin Rodbell discovered the critical roles of guanosine nucleotide–binding proteins (G proteins) in a wide variety of cellular processes, including sensory perception, signalling for cell division, growth and differentiation, intracellular movements of proteins and membrane vesicles, and protein synthesis. The human genome encodes nearly 200 of these proteins, which differ in size and subunit structure, intracellular location, and function. G protein-coupled receptors (GPCRs) are integral membrane proteins that are used by cells to convert extracellular signals into intracellular responses, including responses to hormones, and neurotransmitters, as well as responses to vision, olfaction and taste signals. These receptors form a superfamily of membrane proteins comprising five distinct families based on their sequences and structural similarities: rhodopsin (family A), secretin (family B), glutamate (family C), adhesion and Frizzled/Taste2. They all share common structural motifs in which seven transmembrane (TM) helices are connected to three extracellular loops and three intracellular loops. However, despite structural similarities, GPCRs have unique combinations of signal-transduction activities involving G protein-dependent signalling pathways, as well as G protein-independent signalling pathways and complicated regulatory processes Receptor tyrosine kinases (RTKs) are a subclass of tyrosine kinases that are involved in mediating cell-to-cell communication and controlling a wide range of complex biological functions, including cell growth, motility, differentiation, and metabolism. There are 58 known RTKs in humans, and all RTKs share a similar protein structure comprised of an extracellular ligand binding domain, a single transmembrane helix, and an intracellular region that contains a juxtamembrane regulatory region, a tyrosine kinase domain (TKD) and a carboxyl (C-) terminal tail The binding of a signal molecule to its receptor is an equilibrium process. This is similar to the binding of substrate to an enzyme, but in most cases of receptor binding, there is no chemical modification of the bound ligand. As the concentration of free ligand in the aqueous phase increases or decreases, the ligand associates or dissociates from the receptor; dissociation ends the signalling process. Receptors are excellent targets for the action of pharmaceuticals. Retosiban also known as GSK-221,149- A is an oral drug which acts as an oxytocin receptor antagonist. It blocks the oxytocin- mediated contraction of the uterine smooth muscle in the female uterus that occurs during the initiation of preterm labor. This has been used to prevent preterm labor and premature birth. It has been estimated that about 5% of proteins coded in the human genome are plasma membrane receptors. The high degree of tissue specificity of action of signal molecules is because cells in different tissues express different receptors. As an example, insulin, a signal molecule, alters the metabolism of glucose in muscle and liver but not in most other tissues. Cellular response to a ligand is modulated by the number of receptor molecules in the plasma membrane. An increase in number of receptors, termed upregulation of receptor, the greater the cellular response; A decrease, or downregulation , leads to a decrease in response. Incorporation into and removal from the membrane of receptors is a controlled process. Membrane receptors that span the membrane can have a single transmembrane or multiple transmembrane amino acid domains. The G protein-coupled receptors that occur in bacteria, eukaryotic cells have seven discrete transmembrane domains (7TM receptors). In one class of receptors a surface receptor binds to a ligand and then binds to second receptor (signal receptor) that initiates the cellular reaction. In this case, the ligand binding receptor is referred to as a coreceptor. Some molecules diffuse through cell membranes. Diffusion of a chemical species through a membrane involves (1) leaving the aqueous environment on one side and entering the membrane (2) traversing the lipid milieu of the membrane and (3) leaving the membrane and entering the aqueous phase on the opposite side. Gases, such as 0 2, N 2, CO 2, NO, CO , and H 2S, and lipophilic molecules diffuse through the hydrophobic regions of a membrane. Water diffuses under osmotic forces, but in most cells the rate does not meet the needs for rapid equilibration of the aqueous phases on each side of the plasma membrane; A family of proteins, the aquaporins, facilitates transmembrane movement of water. The shell of water surrounding many solutes must be stripped away before the solute enters the lipid milieu. Inorganic ions and charged organic molecules do not diffuse at a significant rate because of their attraction to water and the exclusion of charged species by the hydrophobic environment of membranes. Uncharged organic molecules, such as sugars, as are peptides , proteins, and nucleic acids are essentially excluded because of their size and charge. If diffusion does occur , the rate is too slow to accommodate cellular needs. Aquaporins Peter Agre, American medical doctor, professor, and molecular biologist was awarded the 2003 Nobel Prize in Chemistry (which he shared with Roderick MacKinnon) for his discovery of aquaporins Over 200 different channel proteins in planes, bacteria, insects, and vertebrates permit the translocation of water and other small molecules and as a group are referred to as aquaporins (AQP). Twelve mammalian aquaporins have been identified, which are divided by amino acid sequence homology and functional characteristics into channels that are selective only for water (aquaporins) and those that permit translocation of water and small solutes (aquaglyceroporins). The aquaporins permit movement of H20 but not hydronium ions (H30+), thereby maintaining the pH gradient across the cell membrane. Humans have at least 10 aquaporins and aquaglyceroporins (AQP0 to AQP9); The primary role of the kidney is co-regulate the pH of blood, eliminate toxic substances of metabolism, and regulate water balance of the body. Each kidney contains about one million nephrons, the multicellular functional units of the tissue. Each nephron has several distinct regions, each with very specific functions in the processing of the blood filtrate, which occurs in the glomerulus. The kidney has at least seven isoforms located at distinct sites along the nephron and collecting duct. A large quantity of blood is filtered (about 150 L/day) and the water must be reabsorbed in the nephron and collecting ducts except for about 1.5 L which is excreted as urine. Aquaporins are responsible for the recovery of water from the filtrate as it flows through the lumen of the nephron. As indicated in the diagram, at least seven different isoforms of aquaporins, located at different sites along the nephron and collecting ducts, are responsible for water reabsorption. Syndrome of inappropriate antidiuretic hormone secretion Low levels of AQP2 and polyuria In some conditions, such as congestive (excessive excretion of urine) are found heart failure, liver cirrhosis, and in acquired nephrogenic diabetes pregnancy, there is an increase in the insipidus (NDI), amount of AQP2 in the kidney, leading to an expansion of the extracellular - acquired hypokalemia (low blood K+), fluid volume. - hypercalcemia (increased blood Ca2+). Humans lacking AQPl activity of the Levels of AQPl, AQP2, and AQP3 in kidney do not have any measurable animal models are reduced in tissue problems under normal but may be under stress conditions (dehydration). ischemia. Protein-Based Mechanisms for Translocation A functional classification system of the membrane transport proteins, recommended by the International Union of Biochemistry and Molecular Biology (IUBMB), divides them into classes, subclasses, families, and subfamilies based on the mechanism of transport and their properties. Many of the proteins are present only in prokaryotes; many others in both prokaryotes and eukaryotes, including mammals. There is a structural homology of the proteins in many of the subfamilies. Pores and channels involve the presence of an aqueous pore in the protein structure through which molecules diffuse; movement is controlled by a gating mechanism. Transporter proteins are similar to enzymes, binding the solute on one side of the membrane, then changing conformation, and moving the substrate to the environment on the opposite side of the membrane. This occurs within the membrane portion of transport protein. Most pores/channels and transporters have a high degree of solute specificity. The rate of substrate translocation by channels is higher than by specific transporters; for channels, the rate is about 10 7 ions/ s, which is the rate of diffusion of a substance in water, whereas with transporters it is about 10 2 - 10 3 molecules/s. A variety of substances, termed substrates, are transported, including inorganic anions and cations, water, metabolic intermediates, organic molecules, drugs, and macromolecules. In eukaryotic cells, translocated substances are unchanged during the process. Some bacteria have a class of transporters termed group translocation where the substrate is chemically modified during transport. Some substrates are transported by two different mechanisms in different cells and some cases in the same cell. Subfamilies of membrane transport proteins have many isoforms, all with the same function. As it was mentioned above, the kidney nephron has at least seven different isoforms of aquaporins, all responsible for the translocation of water. In addition, many transporters are controlled by intra- or extracellular signal molecules and are also referred to as receptors for the specific ligand. Membrane channels and pores are ubiquitous in living organisms, being presented in all types of organisms, from bacteria to higher eukaryotes. Channels and pores permit diffusion in aqueous channels created by the transporter proteins. They do not sequester molecules or ions in transit, and their specificity is based on the size and charge of the substance. Diffusion in channels and pores : (1) downs the concentration gradient of the substance crossing the membrane, (2) is activated without the utilization of metabolic energy for the actual movement of the substance, (3) is regulated by specific mechanisms that open or shut the passageway, And (4) is inhibited by various compounds. Pores consist of several different proteins and, in comparison to channels, have a very complex structure. Pores have relatively large openings, permitting a variety of small and large molecules to traverse the membrane, whereas channels have a high degree of solute specificity. b-Barrel channels have a transmembrane sequence consisting of P-strands that form a b-barrel structure with a water-filled channel from 0.6 to 3 nm in diameter. They are present in the outer membranes of mitochondria (porins), some bacteria, and plastids. Channel proteins have one of two major structures: a- channels and b-barrel channels. a -Type channels are homo- or heterooligomeric proteins, in some cases with several dissimilar subunits, and can have 2 to 22 transmembrane a -helical segments. A common physical characteristic of channels is a structure formed by amphipathic a-helices from different transmembrane segments within a single polypeptide chain or of different protein subunits to create a central aqueous channel. In addition to the domains containing the actual pore, channel proteins contain domains for ligand binding or for sensing physical changes, such as changes in the membrane potential, which control the opening and closing of the channel. a-Type channels in mammals function as cellular sensors, responding to temperature changes, touch, pain, osmolarity, pheromones, taste, and other stimuli. The two most studied classes of channels are those that respond to a change in the transmembrane potential, referred to as voltage-gated channels, and those responding to binding of a ligand, the ligand-regulated channels: Voltage-gated channels have a specific sensor domain in the protein that detects membrane potential changes and transfers the energy to the channel domain to control its gate. As an example, depolarization of the plasma membrane leads to the opening of Na + channels. The mitochondrial outer membrane contains a voltage-dependent anion channel consisting of b-sheets, which permits the passage of substances of up to 10 kDa and has a role in apoptosis Na+, Ca 2+, K+, and Cl- Voltage-Gated Ion Channels The individual voltage-gated ion channels (VIC) for Na +, Ca2+, and K+ are controlled by the transmembrane electrical potential. They open and close remarkably fast (milliseconds), and in mammalian cells are responsible for the generation of conducted electrical signals in neurons and other excitable cells. Each ion channel consists of specific glycoprotein subunits as homo- or hetero-oligomeric structures. The large a -subunits (about 260 kDa) contain the ion-conducting channel. They have four homologous domains each with six transmembrane segments, conneeted by both intracellular and extracellular loops, for a total of 24 transmembrane segments Different classes of channels are very selective concerning the inorganic ion, small organic molecule (e.g., urea), or protein allowed to move through the channel. The Na+ and K+ channel of plasma membranes of eukaryotic cells permits movement of Na + at a rate more than 10 times greater than that for K+, and some K+ channels are lO0- l,000 times more permeable to K+ than Na +. Other channels are less specific. Ligand-regulated channels respond to the binding of specific extracellular or intracellular chemical ligands (also referred to as agonists) and, depending on the channel, leads to either an opening or closing of the channel. The binding of acetylcholine to the nicotinic-acetylcholine receptor opens the channel, allowing the flow of Na + into the cell. This is important in neuronal electrical signal transmission. Other channels are controlled by binding neurotransmitters, cAMP, inositol l,4,5- trisphosphate, diacylglycerol, and G proteins. Opening of most channels is very fast, permitting bursts of ion flow of 10 7 ions per second through the membrane. This rate is necessary because many of these channels are involved in nerve conduction and muscle contraction. The surface of a nerve terminal can contain several hundred different channels, including voltage-dependent channels for Ca2+, K+, Ca2+-gated K+ channels, ligand-gated channels, and stretch-activated channels. Several deadly neurotoxins, including d-tubocurarine, the active ingredient of curare, and several toxins from snakes, including a - bungarotoxin, erabutoxin, and cobratoxin, inhibit the nicotinic- acetylcholine receptor. Succinylcholine, a muscle relaxant, opens the channel leading to depolarization of the membrane; succinylcholine is used in surgical procedures because its activity is reversible due to the rapid hydrolysis of the compound after cessation of administration. Some channels are controlled not only by ligand binding but also by phosphorylation-dephosphorylation. Several pharmacological agents are used therapeutically to modulate channels; those that inhibit are referred to as antagonists. Cystic Fibrosis and the Cl- Channel CF patients have reduced Cl- permeability that impairs fluid and electrolyte secretion, leading to dehydration. Diagnosis of CF is confirmed by a significant increase of Cl- content of sweat of affected in comparison to normal individuals. The CF gene product is the cystic fibrosis transmembrane conductance regulator (CFTR ). CFTR is a cAMP-dependent Cl- channel, which may regulate other ion channels; it is expressed in epithelial tissues. Phosphorylation of a cytoplasmic regulatory domain by protein kinase A activates the channel. MEMBRANE TRANSPORT PROTEINS This discussion centred on the movement of a single substrate by a transporter. Some systems move two substrates simultaneously in one direction and two substrates in opposite directions. When a charged substance is translocated and no ion of the opposite charge is moved or two molecules with different charges are translocated by an antiport mechanism, a charge separation occurs across the membrane. This mechanism is termed S’1 S’2 electrogenic transport and leads to the development of a membrane potential. If an opositely charged ion is moved to balance the charge or two molecules of the same charge are moved in opposite directions across the membrane, the mechanism is termed neutral or electrically silent transport. Electrochemical-potential -driven transporters utilize: 1. a uniport mechanism where a single solute is transported either by mediated diffusion or in a membrane-potential-dependent manner if the solute is charged; 2. an antiport mechanism where two or more solutes are transported in opposite directions in a tightly coupled process not utilizing any form of energy other than the electrochemical- potential gradient; the gradient across the membrane of one solute can drive the movement of the other solute. 3. a symport mechanism where two or more species are transported together in the same direction in a coupled process not utilizing any form of energy other than the electrochemical-potential gradient of one substrate; the gradient of one solute drives the movement of the other solute In the simplest transport mechanism, that is, the transport of a single solute by a uniport mechanism, molecules are transported down their chemical gradient if they have no charge or down an electrochemical gradient if charged; this is often referred to as protein-mediated diffusion or facilitated diffusion. These transporters cannot create a concentration gradient of the substance across the membrane. In a symport (two molecules being moved simultaneously in the same direction) or antiport (two molecules being moved simultaneously in opposite directions), movement down a concentration gradient of one of the solutes can drive the translocation of the other molecule. These have been referred to as secondary active transporters, to distinguish them from primary active transporters. PRIMARY ACTIVE TRANSPORTERS Primary active transport utilizes energy derived from cellular metabolism directly. The large Na+ concentra- tion gradient across the plasma membrane (high extracellular and low intracellular concentration is used to translocate sugars and amino acids by a symport mechanism into cells. Primary active transporters in mammalian cells include transport systems where 1. the free energy of hydrolysis of a pyrophosphate bond is utilized to drive the movement of substrate against their chemical or electrochemical gradient; and 2. the transport protein may be transiently phosphorylated during the transport process, but the substrate is not phosphorylated or modified. Mammalian cells utilize ATP or another nucleoside triphosphate as the energy source. Transporters utilizing ATP are also referred to as ATPases, indicating their enzyme activiyy in hydrolyzing ATP during translocation. Decarboxylation, methyl transfer, oxidoreduction, and light absorption serve to drive transport in other organisms. Three subfamilies of primary active transporters catalyze the translocation of inorganic cations {Na+, K+, Ca2+, or H+) and are classified as P-, V-, or F-type transporters. P-type transporters are phosphorylated and dephosphorylated during transport; there are over 300 members of this subfamily. V-type transporters (V for vacuole) are proton pumps, responsible for acidification of the interior of lysosomes, endosomes, Golgi vesicles, and secretory vesicles. F-type transporters, present in mitochondria, chloroplasts, and bacteria, translocate protons at the expense of ATP hydrolysis but synthesize ATP when functioning in the reverse direction, that is, when protons are transported down their concentration gradient. Ca+ Translocation Is Catalyzed by a Primary Active Transporter: Calcium is an important intracellular messenger that regulates many cellular processes from carbohydrate metabolism to muscle contraction. Cytosolic Ca2+ is about 10,000 times less than that of extracellular Ca2+. Ca2+ in the cytosol increases rapidly by release of Ca2+ from the endoplasmic or sarcoplasmic reticulum or transient opening of Ca2+ channels in the plasma membrane permitting flow of Ca2+ down the large concentration gradient. To reestablish low cytosolic levels, Ca2+ is actively transported by two dif- ferent Ca2+ -transporting ATPases (Ca2+ -ATPase), one transporting Ca2+ back into the lumen of the endoplasmic or sarcoplasmic reticulum, and the other out of the cell across the plasma membrane. The Ca2+ -ATPases are P-type transporters , phosphorylated by ATP on an aspartyl residue; Glucose is translocated by a Passive Uniport Mechanism. A family of glucose transporters (GLUT ) facilitates the transport of d- glucose across the plasma membranes of mammalian cells by a uniport mechanism. Glucose and Amino Acids Are Translocated with Na+ by a Symport Mechanism The diagram represents the transport of d-glucose driven by the movement of Na +. In the process two Na + move into the cell down the Na+ electrochemical gradient by passive facilitated transport, and glucose is carried along against its concentration gradient Cl- and HC0 3 - Are Transported by an Antiport Mechanism An anion transporter in erythrocytes and kidneys mediates the electroneutral antiport movement of Cl- and HCO 3. The transporter is referred to as the Na+ independent Cl-- HCO3 - exchanger or anion exchange protein. The transporter is important in adjusting the erythrocyte HCO 3 - concentration in arterial and venous blood. The kidney transporter is a truncated form of the erythrocyte protein and is responsible for base (HCO 3-) efflux to balance ATP-driven H+ efflux. Diseases due to Loss of Membrane Transport Systems Individuals with a decrease in glucose uptake from the intestinal trace lack the specific sodium-coupled glucose-galactose transporter. Fructose malabsorbtion syndromes are caused by an alteration in the activity of the transport system for fructose. In Harrnup disease there is a decrease in the transport of neutral amino acids in the epithelial cells of the intestine and renal tubules. In cystinuria, renal reabsorption of cystine and the basic amino acids lysine, arginine, and ornithine are abnormal, resulting in the formation of renal cystine stones. In hypophosphatemic, vitamin D – resistant rickets, renal absorption of phosphate is abnormal. Recently there have been reports about genetic-based diseases involving the sub- strate transporters for dopamine and creatine, and the mitochondrial aspartate glutamate transporter. The various ATP-requiring active transporters have been implicated in several pathophysiological conditions. Textbook of Biochemistry with clinical correlations, Thomas M. Devlin - pp.477-496