A2 Biology Chapter 1 Coordination and Response PDF
Document Details
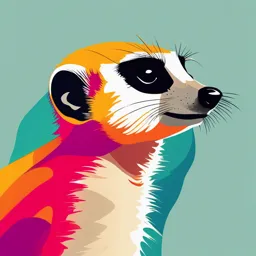
Uploaded by RegalHeliotrope8645
Escuela Amador
A2
Dr.Aly Wael
Tags
Summary
This document is a chapter from an A2 Biology course. It covers coordination and response in humans, including the nervous system, muscle contraction, and communication between the nervous and endocrine systems. The chapter also examines receptors, nerve impulses, and synaptic transmission. This chapter has many questions relating to coordination and response in biology.
Full Transcript
Dr.Aly Wael A2 Biology Chapter 1 Coordination and response 1 Dr.Aly Wael A2 Biology Points you shou...
Dr.Aly Wael A2 Biology Chapter 1 Coordination and response 1 Dr.Aly Wael A2 Biology Points you should know 1. Compare the nervous and endocrine systems as communication systems that co-ordinate responses to changes in the internal and external environment 2. Describe the structure of a sensory neuron and a motor neuron 3. Outline the roles of sensory receptor cells in detecting stimuli and stimulating the transmission of nerve impulses in sensory neurons (a suitable example is the chemoreceptor cell found in human taste buds) 4. Describe the functions of sensory, relay and motor neurons in a reflex arc 5. Describe and explain the transmission of an action potential in a myelinated neuron and its initiation from a resting potential (the importance of sodium and potassium ions in impulse transmission should be emphasised) 6. Explain the importance of the myelin sheath (salutatory conduction) in determining the speed of nerve impulses and the refractory period in determining their frequency 7. Describe the structure of a cholinergic synapse and explain how it functions, including the role of calcium ions 8. Outline the roles of synapses in the nervous system in allowing transmission in one direction and in allowing connections between one neuron and many others (summation, facilitation and inhibitory synapses are not required) 9. Describe the roles of neuromuscular junctions, transverse system tubules and sarcoplasmic reticulum in stimulating contraction in striated muscle 10. Describe the ultrastructure of striated muscle with particular reference to sarcomere structure 11. Explain the sliding filament model of muscular contraction including the roles of troponin, tropomyosin, calcium ions and ATP 12. Describe the rapid response of the Venus fly trap to stimulation of hairs on the lobes of modified leaves and explain how the closure of the trap is achieved 13. Explain the role of auxin in elongation growth by stimulating proton pumping to acidify cell walls 14. Describe the role of gibberellin in the germination of wheat or barley 15. Explain the role of gibberellin in stem elongation including the role of the dominant allele, Le, that codes for a functioning enzyme in the gibberellin synthesis pathway, and the recessive allele, le, that codes for a non-functional enzyme 2 Dr.Aly Wael A2 Biology 1.1 Co-ordination in humans: Coordination: to interact and respond to certain stimulus. Sensitivity: the ability to detect a stimuli and to make appropriate responses Stimuli: changes in an organism’s environment and are sensed by special cells called receptors and the organism responds using effectors (muscles or glands). Response: what happens when an organism reacts to the stimulus. The nervous system (rakez awy fl heta di): it is made of up nerves, nerves are large fibers made up of small neurons (neurons are the building unit of the nerve fiber, which is a modified cell). These nerves are capable of doing 2 things; sensation and ordering a muscle or a gland to function. El nervous system mat2sama l hagten; central nervous system (brain and spinal cord homa el mas2olin) peripheral nervous system (da 2ay nerve tany taba3o) Reflex arc: it is the pathway along which impulses are transmitted from a receptor to an effector during reflex action. Receptor → Sensory →Relay→ Motor→ Effector Reflex action: 1. Doesn’t require thinking 2. Uses three neurons 3. Rapid response that protects our body from any injury 4. Always same response to the same stimulus 3 Dr.Aly Wael A2 Biology Neurons: There are three types of neuron, each with a different function. 1. Sensory neurons transmit impulses from receptors to the CNS 2. Intermediate neurons (also known as relay or connector neurons) transmit impulses from sensory neurons to motor neurons 3. Motor neurons transmit impulses from the CNS to effectors Sensory neuron: Axon: It has one long axon which start at the receptor, the part before the cell body is called afferent axon (Dendron) and carry impulses towards the cell body, and an axon that carry impulses away from it. Cell body: containing nucleus located at the ganglion in the spinal chord. Motor neuron: Cell body: lies within the CNS, it contains nucleus, many mitochondria and RER, responsible for protein and neurotransmitters synthesis. Dendrites: many short thin and branching cytoplasmic extensions coming from cell body to provide large surface area for ending of other neurons. Dendrites carry impulses from other neurons towards the cell body (soma). Axon: it is a single long fiber made of cytoplasmic extension, it extends from CNS to the effector, conducts impulses away from the cell body. Within the cytoplasm of an axon there are some organelles such as mitochondria. The ends of the branches of the axon have large numbers of mitochondria, together with many vesicles containing neurotransmitter. 4 Dr.Aly Wael A2 Biology Myelin sheath: 1. Made by specialised cells called Schwann cells that wrap many times around the axons of some neurons along their length. 2. Myelin sheath, is made largely of lipid, together with some proteins. 3. It speeds up the nerve conduction, protects and insulates neuron. 4. The uncovered area of axon between Schwann cells are called nodes of Ranvier. They occur about every 1–3 mm in human neurons. The nodes themselves are very small, around 2–3 μm long. 5. Relay neuron is not mylenated. Types of receptors: A cell that responds to one such stimulus by initiating an action potential is called a receptor cell. Other receptors, such as some kinds of touch receptors, are simply the ends of the sensory neurons themselves Role of receptors: 1. Sensory receptors are specific to certain chemicals 2. They act as transducers they convert energy in one form such as light, heat or sound into receptor potential which is a change in membrane potential of receptor cell. 3. Receptor potential triggered, leads to release of neurotransmitter from the end of receptor cell which form synapse with sensory neuron. 4. If enough neurotransmitter is released, and stimulation of sensory neuron exceeds the threshold (point mo3ayana) an action potential (impulse) is generated along sensory neuron. 5 Dr.Aly Wael A2 Biology Chemoreceptors in test buds: 1. Taste buds are onion shaped structures located in the epithelium of the tongue, imbedded in papillae which cover the tongue. 2. Within each taste bud are between 50 and 100 receptor cells that are sensitive to chemicals in the liquids that we drink or chemicals from our food that dissolve in saliva. 3. Each chemoreceptor has microvilli to increase surface are and covered with receptor proteins that detect these different chemicals. 4. There are several types of receptor proteins, each detecting a different type of chemical and giving us a different sensation. There are five tastes: sweet, sour, salt, bitter and umami (savoury). Chemoreceptors sensitive to salt: 1. These are specific receptors to sodium ions present in salt 2. Sodium ions diffuse through specific channel proteins in cell membrane of microvilli in receptor cell. 3. Membrane depolarizes which is known as receptor potential. 4. If the receptor potential exceeds threshold it stimulates opening of Ca+2 voltage-gated channels. 5. Ca+2 enter the cytoplasm causes exocytosis of vesicles containing neurotransmitters from basal membrane. 6. If the receptor potential releases enough neurotransmitter, that binds to receptors on membrane of sensory neuron, this creates action potential (impulse) in the sensory neuron with which it synapses, that carry impulses to the taste center in brain. Chemoreceptors sensitive to sweet: 1. When sugar molecule bind receptors this stimulate a G protein. 2. G protein activates an enzyme to produce many molecules of cyclic AMP. 3. Cyclic AMP acts as a second messenger activating a cascade to amplify the signal 4. Leading to the closure of potassium ion channels. This also depolarizes the membrane. 5. The receptor potential should be above the threshold value in order to stimulate the sensory neuron. 6 Dr.Aly Wael A2 Biology Resting potential: In a resting axon, it is found that the inside of the axon always has a slightly negative electrical potential compared with the outside. The difference between these potentials, called the potential difference, is often between −60 mV and −70 mV. In other words, the electrical potential of the inside of the axon is between 60 and 70 mV lower than the outside. Membrane is now polarized. How resting potential is maintained: 1. Na+ and K+ cannot pass through the phospholipid bilayer, instead, they pass through carrier proteins known as sodium–potassium pump. 2. This carrier protein pumps 3 Na+ out of the cell axon and pumps 2 K+ into the axon (3 osad etnen) using ATP to move ions against concentration gradient. 3. As a result, there are more Na+ in the tissue fluid than the cytoplasm and more K+ in the cytoplasm than tissue fluid ya3ny 3andy chemical gradient 4. There are more channel proteins for K+ than Na+. These are leaky channels which are always opened that allow K+ to diffuse out of the cell faster than the diffusion of Na+ into the cell. 5. There are some negatively charged proteins in the cytoplasm that attract K+ which contribute to the overall negative state. 6. An equilibrium is established when there is no net movement of ions and which is a balance between chemical and electrical gradients. 7. Now the membrane has more negatively charged ions on the inside compared with outside (-70 mV) the membrane is now polarized. 7 Dr.Aly Wael A2 Biology Generating action potential: 1. At resting potential, Na+ and K+ voltage gated channels are closed. 2. Some Na+ channels open due to stimulus. 3. Na+ diffuses into the cell through opened gated channels down their electrochemical gradient. 4. The influx of Na+ which are positively charged causes the depolarization of the membrane 5. Therefore membrane potential becomes less negative on the inside. 6. This depolarisation triggers some more channels to open so that more sodium ions enter. There is more depolarisation. 7. If the potential difference reaches about −50 mV (threshold value), then many more channels open and the inside reaches a potential of +30 mV compared with the outside. This is an example of positive feedback 8. When the inside reaches a potential of +30 mV all the sodium ion voltage-gated channels close, so sodium ions stop diffusing into the axon. 9. At the same time, the potassium ion channels open. Potassium ions therefore diffuse out of the axon, down their concentration gradient. 10. The outward movement of potassium ions removes positive charge from inside the axon to the outside, thus returning the potential difference to normal (−70 mV). This is called repolarisation. 11. There is a delay in closing potassium channels which causes a temporary overshoot of electrical gradient and the inside potential is lower than -70 mV which is called hyper repolarization. 12. K+ channels close and Na+ channels become responsive to depolarization again. The sodium–potassium pump continues to pump Na+ out and K+ in even faster to restore resting potential. 8 Dr.Aly Wael A2 Biology Transmission of action potential: 1. Depolarization of a point of the membrane set up a local circuit between depolarized region and resting region. 2. Positively charged ions flow lengthways inside the axon away from positively charged region on either side, this also occurs on the outer side of the axon membrane. 3. These local circuits depolarize the adjacent regions creating action potential 4. Action potential begins at one end and are propagated ahead not behind because the region behind is still in recovery period, Na+ gated channels are shut tight and cannot be stimulated this is called refractory period. Saltatory conduction: It is the transmission of nerve impulses along myelinated neurons. 1. Myelin sheath insulates the axon as ions cannot pass through it. 2. Action potential only occurs at nodes of Ranvier. 3. Local circuits occurs between nodes of Ranvier. 4. Action potential jumps from node to node, this is faster transmission. Refractory period: When the sodium ion voltage-gated channels are ‘shut tight’ and cannot be stimulated to open, however great the stimulus. This period of recovery is the refractory period when the axon is unresponsive. There are several consequences of there being refractory periods: 1. Action potential can only move forward. 2. Action potentials are discrete events; they do not merge into one another. 3. There is a minimum time between action potentials occurring at any one place on a neuron. 4. The length of the refractory period determines the maximum frequency at which impulses are transmitted along neurons. 9 Dr.Aly Wael A2 Biology Synapse: It is the point where the axon of one neuron connects with the dendrite of another neuron, including a space between them called synaptic cleft. How the impulse is transmitted across the synapse: 1. The arrival of the action potential causes calcium ion voltage- gated channels to open Ca+ diffuses into the cytoplasm of the. Pre-synaptic neuron. 2. Ca+ causes vesicles that contain acetyl choline (ACh) to move towards pre-synaptic membrane and fuse with it and release ACh into synaptic cleft by exocytosis. 3. ACh diffuse along synaptic cleft and bind temporarily with receptors on post synaptic membrane. 4. Receptors and then changes shape opens Na+ channels. Na+ diffuses into cytoplasm of post synaptic neuron which causes depolarization of the membrane generating action potential. 5. Acetyl cholinesterase enzyme is present in synaptic cleft to hydrolise ACh to acetate and choline. 6. Choline is taken back to presynaptic neuron to be recycled; it combines with acetyl CoA to form ACh once more using ATP to be ready for next action potential. Function of synapse: 1. Synapses ensure one-way transmission: Impulses can only pass in one direction at synapses. This is because neurotransmitter is released on one side and its receptors are on the other. 2. Filter out low level stimuli: If the depolarisation of the postsynaptic membrane does not reach the threshold, no impulse is sent in that neuron. One advantage of this is that impulses with low frequencies do not travel from sensory neurons to reach the brain. This means that the brain is not overloaded with sensory information. 3. Synapses allow the interconnection of nerve pathways: Individual sensory and relay neurons have axons that branch to form synapses with many different neurons; this means that information from one neuron can spread out throughout the body to reach many relay neurons and many effectors as happens when we respond to dangerous situations. 10 Dr.Aly Wael A2 Biology One relay neuron integrate the information coming from many different parts of the body – something that is essential for decision-making in the brain. 4. Synapses are involved in memory and learning: if your brain frequently receives information about two things at the same time, say the sound of a particular voice and the sight of a particular face, then it is thought that new synapses form in your brain that link the neurons involved in the passing of information along the particular pathways from your ears and eyes. In future, when you hear the voice, information flowing from your ears along this pathway automatically flows into the other pathway too, so that your brain ‘pictures ‘the face which goes with the voice. Relationship between intensity of the stimulus and nerve impulse: All-or-nothing law: If the receptor potential is below a certain threshold, the stimulus only causes local depolarisation of the receptor cell. If the receptor potential is above the threshold, then the receptor cell stimulates the sensory neuron to send impulses. Above this threshold, action potentials are initiated in the sensory neuron. Action potential size: the size (amplitude) of action potential does not change with distance along the axon and also does not change regardless the intensity of the stimulus. Action potential speed: the speed of action potential does not change with different intensities of stimulus. Frequency: when the intensity of the stimulus increases, frequency of impulses increase, in other words, more impulses pass in a given time. Number of neurons stimulated: more neurons are stimulated with strong stimulus than weak stimulus. 11 Dr.Aly Wael A2 Biology Structure of striated muscle: A muscle such as a biceps is made up of thousands of muscle fibers. Each muscle fiber is a very specialised ‘cell’ with a highly organised arrangement of contractile proteins in the cytoplasm, surrounded by a cell surface membrane. The muscle fiber is multinucleated and it is called syncytium. Sarcolemma: it is the cell surface membrane of the syncytium. Transverse system tubules (T-tubules): they’re many infolding in the sarcolemma. T-tubules ran into the cytoplasm very close to the endoplasmic reticulum. Sarcoplasmic reticulum: it is the endoplasmic reticulum of syncytium. The membranes of the sarcoplasmic reticulum have huge numbers of protein pumps that transport calcium ions into the cisternae of the SR. Sarcoplasm: it is the cytoplasm of syncytium. It contains large number of mitochondria to produce ATP. Myofibril: Parallel groups of thick filaments lie between groups of thin ones. Both thick and thin filaments are made up of protein. The thick filaments are made mostly of myosin, whilst the thin ones are made mostly of actin. 12 Dr.Aly Wael A2 Biology Structure of sarcomere: Sarcomere is the part between two Z lines and it is the contractile part. A band: it is the darkest part of the striation which contain overlap of thick myosin filaments and thin actin filaments. I band: The lighter parts where there are no thick filaments, only thin actin filaments. H band: the lighter area within the A band, represents the parts where only the thick filaments are present. M line: provide attachment to myosin filaments. Z line: provide attachment to actin filaments. Structure of thick and thin filaments: Myosin: it is a fibrous protein with a globular head. The fibrous portion helps to anchor the molecule into the thick filament. Within the thick filament, many myosin molecules all lie together in a bundle with their globular heads all pointing away from the M line. It forms the thick filaments. Actin: it is a globular protein. Many actin molecules are linked together to form a chain. Two of these chains are twisted together to form a thin filament. Also twisted around the actin chains is a fibrous protein called tropomyosin. Another protein, troponin, is attached to the actin chain at regular intervals. Actin has myosin binding sites. 13 Dr.Aly Wael A2 Biology Sliding filament mechanism of muscle contraction: 1. Action potential arrives at the neuromuscular junction (synapse between neuron and muscle). Then action potential propagates along sarcolemma and T-tubules of muscle fiber. 2. Depolarization of T-tubule causes nearby Ca2+ voltage-gated channels on SR to open, now the SR is more permeable to Ca2+ so it diffuses into sarcoplasm (from SR). 3. Ca2+ binds to troponin causing troponin-tropomyosin complex to change shape, this causes the myosin binding site on actin filaments to be exposed (uncovered). 4. ATPase enzyme in myosin head catalyses the hydrolysis of ATP to ADP which releases energy causing myosin heads to be energized. 5. Energized myosin heads can bind to myosin binding site on actin forming cross bridge between the thin and thick filaments. (Cross bridge formation) 6. Then myosin head tilts pulling the acting filament toward the M line causing shortening of sarcomere and releases ADP molecule. (Power Stroke) 7. Another ATP molecule binds to the myosin head causing it to detach from actin filament. (Cross bridge detachment) 8. When there’s no stimulation, Ca2+ channels in SR close, Ca2+ leave their binding site on actin and are pumped back into SR by active transport, this allows tropomyosin to block actin filament again. 14 Dr.Aly Wael A2 Biology Comparison between endocrine and nervous system: P.O.C Endocrine system Nervous system Electrical → Action Communication by: Chemical → Hormones potential / Nerve impulses Pathway: Blood plasma Nerve fibers/Neurons Speed: Slower Faster All over the body but only Spread: Specific parts of the body target organs respond Effect: widespread Localised Duration: Long lasting Short lasting 15 Dr.Aly Wael A2 Biology 1.2 Co-ordination in plants: Chemicals known as plant hormones or plant growth regulators are responsible for most communication within plants. Auxins: they influence many aspects of growth including elongation growth which determines the overall length of roots and shoots Gibberellins: which are involved in seed germination and controlling stem elongation. Abscisic acid (ABA): it is another plant hormone, which controls the response of plants to environmental stresses such as shortage of water. Auxin and elongation growth: Indol acetic acid (IAA/auxin) is the principle one of many types of auxins. IAA is secreted from growing tips (meristem) in shoots or roots. It is transported by active transport or through phloem away from the tip. IAA control plant growth by stimulating cell elongation by absorption of water. Mechanism of cell elongation by auxin: 1. When auxin binds to receptor on membrane, it stimulate the ATPase proton pump to pump move H+ out of the cytoplasm into cell wall lowering the pH of the cell wall. 2. Low pH activates expansins proteins (enzymes) present in cell wall which loosens the bonds between micro fibrils and disrupt the non-covalent interaction between cellulose and hemicellulose. 3. This stimulates K+ channels to open allowing K+ to enter increasing it’s concentration in cytoplasm lowering the water potential inside the cell. 4. Water enters the cell by osmosis through aqua porins increasing the pressure potential inside causing the cell wall to stretch and cell elongates. 16 Dr.Aly Wael A2 Biology Gibberellin: are plant growth regulators that are synthesised in most parts of plants. They are present in especially high concentrations in young leaves and in seeds, and are also found in stems, where they have an important role in determining their growth. 1) Role of gibberellin in stem elongation: Plant stem height is determined by gene with 2 alleles: o The dominant allele (Le) of this gene regulates the synthesis of the last enzyme in pathway that produces an active form of gibberellin, GA1. Active gibberellin stimulates cell division and cell elongation in the stem, so causing the plant to grow tall. o Recessive allele for short stem (le) which codes for non-functioning enzyme, therefore cannot produce active gibberellin. This recessive allele le is due to substitution mutation. 2) Role of gibberellin in seed germination: 1. DELLA is a type of protein (transcription factor) that inhibits the production of mRNA which codes for amylase. 2. Gibberellins hormone promotes destruction DELLA protein. 3. When seeds are formed they remain dormant due to lack of water to be able to stand adverse conditions. 4. When water is added to seeds it stimulates the embryo to produce gibberellins, which destroys DELLA. 5. mRNA is made and amylase is produced. 6. Amylase diffuse into endosperm, hydrolyses the starch into maltose then to glucose. 7. Glucose is translocated to the embryo to be used in respiration or making cellulose. 17 Dr.Aly Wael A2 Biology Venus fly trap: Structure: 1. The modified leaf is divided into two lobes on either side of a mid- rib. 2. When open the surface of lobes is convex (bulging upwards), red in colour with three stiff sensory hairs that detect when being deflected. 3. Along the edge of lobes there are nectary glands to attract insects and stiff hairs that interlock to trap the insect inside. 4. Many glands on the surface of the leaf secrete enzymes to digest the trapped insects. Mechanism of closing the trap: 1. An insect stimulate at least two of the three hairs or one hair twice within 20 to 35 sec. 2. An action potential is generated and reaches cells of mid rib (hinge cells) through cell membranes and plasmodesmata. 3. In response of action potential the concentration of auxin increases in hinge cells. 4. Auxin stimulates proton pump to pump move H+ out of the cytoplasm into cell wall lowering the pH of the cell wall. 5. Low pH dissolves calcium pectate that holds the cell walls fibers together causing loosening of cell wall. 6. Loss of H+ makes inside the cell more negative, so positively charged Ca2+ are attracted into the cells lowering it’s water potential. 7. Water flows by osmosis from cells on the top to cells underneath of lobe leaf. 8. Cell walls of lower part of the leaf and hinge cells, expand, causing the lobes of the leaf to become concave and hinge cells to close the trap. 9. The trapped insect keeps deflecting the sensory hair more, this trigger further action potential forcing the edges of the lobes together, sealing the trap. 10. Further deflection of sensory hairs by the prey stimulates the entry of Ca2+ into glad cells on the upper surface. 11. Ca2+ stimulates exocytosis of vesicles containing digestive enzymes. 12. Once insect is digested, the trap reopens as cells on the upper surface grow slowly. 18