Anesthesia: Definition, History, and Principles PDF
Document Details
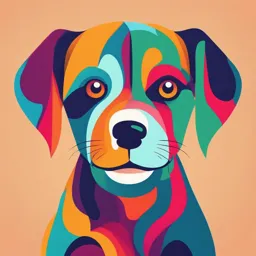
Uploaded by EasyDaisy
Assoc. Prof. M. Atanasova
Tags
Summary
This document is lecture notes on anesthesia, covering its definition, history, principles, endotracheal intubation, and various types of volatile anesthetics. It discusses different stages of general anesthesia and their characteristics.
Full Transcript
Anesthesia-definition, history. General anesthesia. Principles. Endotracheal intubation. Volatile anesthetics and general volatile anesthesia Assoc. Prof. M. Atanasova Anesthesia - basic From Greek anesthesia means “no sensation” When the effect of...
Anesthesia-definition, history. General anesthesia. Principles. Endotracheal intubation. Volatile anesthetics and general volatile anesthesia Assoc. Prof. M. Atanasova Anesthesia - basic From Greek anesthesia means “no sensation” When the effect of ether was discovered “anesthesia” used as a name for the new phenomenon Anesthesia is defined as a abolition of sensation Analgesia is defined as the abolition of pain Triade of general Anesthesia: 1. Need for unconsciousness 2. Need for analgesia 3. Need for muscle relaxation General anesthesia – reversible, unconscious state using pharmacology. It is characterized by amnesia (sleep, hypnosis or basal narcosis), analgesia (freedom from pain), depression of reflexes, muscle relaxation 2 Anesthesia - basic Stages of general anesthesia (Guedelʼs signs), typically seen in case of ether: 1. Analgesia and sedation stage – starts from beginning of anesthetic inhalation and lasts upto the loss of consciousness; Patient is conscious, can hear and see, and feels, spontaneous respiration, reflexes present, pain is progressively abolished. Possible small surgery procedures 2. Excitation stage – from loss of consciousness to beginning of regular respiration; muscle tone increases, jaws are tightly closed, uncontrolled movements, vomiting, HR and BP may rise and pupils dilate due to SY stimulation, increase in respiratory rate; this stage is not found with modern anesthesia 3. Anesthesia for surgery – it begins with lack of lid reflex, extends from onset of regular respiration to cessation of spontaneous breathing; 4 sub-stages 3 Anesthesia - basic Stages of general anesthesia: 4 sub-stages 1. Roving eye balls; ends when eye become fixed 2. Loss of corneal and laryngeal reflexes 3. Pupil starts dilating and light reflex is lost 4. Intercostal paralysis, shallow abdominal respiration, dilated pupils 4 Anesthesia - basic Stages of general anesthesia: 4. Intoxication - cessation of breathing (respiratory arrest) and failure of circulation (cardiac arrest) – death; widely dilated pupils, muscles are totally flabby, pulse is imperceptible, BP is very low Anesthesia events practically: 1. Induction – the period of time which begins with the administration of an anesthetic upto the development of surgical anesthesia 2. Maintenance – sustaining the state of anesthesia 3. Recovery – anesthetic stopped at the end of surgical procedure and consciousness regain 5 Discovery of anesthesia Discovery of anesthesia represents a totally American Contribution to medicine 1842 - Dr. Crawford Long, a medical practitioner was the first physician known to administered the vapor of ether by inhalation to produce surgical anesthesia. The finding was not publicized 1846 – Dr. William Morton administered vapor of ether to Mr. Abbot for the removal of tumor from below of the mandible by the well-known surgeon Dr. John C. Warren The successful anesthesia took place at Massachusetts General Hospital on Friday, October 16 in 1846 6 Discovery of anesthesia 1847 - In England, Dr. James Simpson administered ether to a parturient to relieve the pain of labor 1853 – Dr. John Snow administered chloroform to Queen Victoria during the birth of Prince Leopold. Dr. Snow qualifies as the first anesthesiologist because he was the first who devote his medical practice to the administration of anesthetics Although nitrous oxide was isolated in 1772 and its anesthetic properties were described in 1799, it was 1844 when Dr. Wells demonstrated an administration of nitrous oxide for teeth extraction 7 Discovery of anesthesia 1878 - Endotracheal tube was discovered 1885 – Local anesthesia with cocaine 1934 – Thiopental was first used 1942 – Curare was first used, opened the “Age of Anesthesia” 8 Inhalation anesthesia Inhalation anesthesia refers to delivery of gases (nitrous oxide, xenon) or volatile liquids (halothane, enflurane, isoflurane, sevoflurane, desflurane) from the respiratory system to produce anesthesia Pharmacokinetics – uptake, distribution and elimination from the body Pharmacodynamics – MAC value 9 Pharmacokinetics of inhaled anesthetics PhK describes their uptake (absorption) from alveoli into the systemic circulation, distribution in the body and elimination via the lungs or metabolism in the liver By controlling the inspired partial pressure (same as concentration) of IA, a gradient is created such that the A is delivered from the A machine to the brain The brain and all other tissues equilibrate with the partial pressure of the IA delivered to them by the arterial blood The blood equilibrate with alveolar partial pressure 10 Pharmacokinetics of inhaled anesthetics Maintaining a constant and optimal alveolar partial pressure (PA) becomes an indirect but reliable method for controlling the partial pressure in the brain (Pbr) PA is used as an index of anesthetic depth, reflection of the rate of induction and recovery from anesthesia Understanding those factors that determine the PA and thus the Pbr allows the anesthesiologist to control the dose of IA delivered to the brain 11 Factors determining the PA The PA (and ultimately Pbr) of an IA is determined by input (delivery) into the alveoli minus uptake (loss) of the IA from the alveoli into the arterial blood Input is dependent on 3 factors: PI, alveolar ventilation (VA), characteristics of the anesthetic breading system Uptake of the IA is dependent on 3 factors: solubility, cardiac output, alveolar to venous partial pressure difference These 6 factors act simultaneously to determine the PA. Metabolism and percutaneous loss do not significantly influence PA during induction and maintenance of anesthesia 12 Input – 3 factors: PI, VA, Breathing system The initial high inspired partial pressure (PI) offsets the impact of uptake and thus accelerates induction of anesthesia as reflected by the rate of rise in the PA. This effect of the PI is known as the concentration effect. Second gas effect – phenomenon that occurs independently of the concentration effect. The ability of the large volume uptake of one gas (first gas) to accelerate the rate of rise of the PA of a concurrently administered companion gas (second gas). For example, the initial large volume uptake of nitrous oxide accelerates the uptake of volatile anesthetics and oxygen. 13 Input – 3 factors: PA, VA, Breathing system Increased VA, like PI promotes input of inhaled anesthetics to offset uptake. The net effect is a more rapid rate of rise in the PA and induction of anesthesia. Hypoventilation has the opposite effect, acting to slow the induction of anesthesia Controlled ventilation that results in hyperventilation and decreased venous return accelerates the rate of rise of the PA by virtue of increased input (increased VA) and decreased uptake (decreased CO) Another effect of hyperventilation is decreased cerebral blood flow due to reductions in the PaCO2 14 Input – 3 factors: PA, VA, Anesthetic breathing system Characteristics of the anesthetic breading system that influence the rate of rise of the PA are: 1. Volume of the system (acts as a buffer to slow achievement of the PA – high gas inflow negates this effect) 2. Solubility of IA in the rubber or plastic components of the system (slows the rate at which the PA rises) 3. Gas inflow from the anesthetic machine 15 Uptake – 3 factors: solubility, CO, alveolar to venous partial pressure difference Solubility of IA in blood and tissues is denoted by partition coefficient. A partition coefficient is a distribution ratio describing how the IA distributes itself between two phases at equilibrium (i. e., when the partial pressures are identical) Solubility of IA in blood is quantified as the blood : gas partition coefficient – the ratio of concentration of an anesthetic in the blood phase to the concentration of the A in the gas phase when the A is in equilibrium between the two phases 16 Uptake – 3 factors: solubility, CO, alveolar to venous partial pressure difference Partition coefficients are temperature-dependent (solubility of a gas in a liquid is increased when the temperature of the liquid decreases Lower the blood : gas partition coefficient – faster the induction and recovery Higher the blood : gas partition coefficient – slower induction and recovery 17 Uptake – 3 factors: solubility, CO, alveolar to venous partial pressure difference Based on their blood-gas partition coefficient (i.e., solubility) IA are considered as soluble (methoxyflurane), of intermediate solubility (halothane, enflurane, isoflurane) and poorly soluble (nitrous oxide) High blood-solubility means that a large amount of IA must be dissolved in the blood before equilibrium is reached with the gas phase. When the blood-gas partition coefficient is high, a large amount of IA must be dissolved in the blood before the Pa equilibrates with the PA. When blood solubility is poor, as with nitrous oxide, minimal amounts of IA have to be dissolved in the blood before equilibrium is reached such that the rate of rise of the PA and thus that of the Pa and Pbr are rapid 18 Uptake – 3 factors: solubility, CO, alveolar to venous partial pressure difference Tissue:blood partition coefficient – determine the time necessary for equilibration of the tissue with the Pa This time can be predicted by calculating a time constant (amount of IA that can be dissolved in the tissue divided by tissue blood flow) Brain:blood partition coefficients for IA are approximately 2,5, resulting in a time constant equal to roughly 5 minutes Complete equilibration of any tissue with the Pa requires at least 3 time constants (approximately 15 minutes) 19 Uptake – 3 factors: solubility, CO, alveolar to venous partial pressure difference Nitrous oxide transfer to closed gas spaces – the blood-gas partition coefficient 0,47 is 34 times greater than nitrogen (0,014). Nitrous oxide can leave the blood to enter an air-filled cavity 34 times more rapidly than nitrogen can leave cavity to enter the blood The volume or pressure of air-filled cavity increases The entrance of nitrous oxide into the air-filled cavity surrounded by a compliant wall (intestinal gas, pneumothorax, pulmonary blebs, air embolism) causes the gas space to expand The entrance of nitrous oxide into the air-filled cavity surrounded by a noncompliant wall (middle ear, cerebral ventricles) causes the increase in pressure 20 Uptake – 3 factors: solubility, CO, alveolar to venous partial pressure difference In the animal model the inhalation of 75% nitrous oxide doubles the volume of pneumothorax in 10 minutes. Therefore, the presence of a closed pneumothorax is a contraindication to the administration of nitrous oxide If the operation is short the question of whether to administer nitrous oxide to patient with a bowel obstruction is of little importance Limiting the inhaled concentration of nitrous oxide to 50% may be a prudent recommendation when bowel gas volume is increased preoperatively 21 Uptake – 3 factors: solubility, CO, alveolar to venous partial pressure difference The CO influence uptake and therefore PA by carrying away more or less anesthetic from alveoli A high CO (fear) results in more rapid uptake such that the rate of rise in the PA – the induction of anesthesia is slowed A low CO (shock) speeds the rate of rise of PA since there is less uptake to oppose input – induction of anesthesia in patient in shock is rapid 22 Uptake – 3 factors: solubility, CO, alveolar to venous partial pressure difference Highly perfused tissues (brain, heart, liver, kidneys) account for less than 10% of body mass but receive 75% of the CO – these tissues equilibrate rapidly with the Pa After 3 time constants (15 minutes) 75% of the returning venous blood is at the same partial pressure as the PA Skeletal muscles and fat are 70% of body mass but receive less than 25% of the CO These tissues act as inactive reservoir for anesthetic uptake for several hours 23 Recovery from anesthesia Can be defined as the rate at which the PA decreases with time Recovery is the inverse of induction Differences from induction: 1. The absence of a concentration effect on recovery (the PI cannot be less than zero) 2. Variable tissue concentrations of anesthetics at the start of recovery 3. Potential importance of metabolism on the rate of decline in the PA (metabolism and VA are equally important in the rate of decline in the PA of halothane, metabolism is a principal determinant in the rate of decline in the PA of methoxyflurane, and the rate of decline in the PA of less lipid soluble enflurane and isoflurane is due to VA 24 Recovery from anesthesia Diffusion hypoxia – may occur at the conclusion of nitrous oxide administration if patient is allowed to inhale room air When inhalation of nitrous oxide is discontinued the initial high volume outpouring of nitrous oxide from the blood into the alveoli can dilute the PAO2 that the PaO2 decreased Diffusion hypoxia is prevented by filling the lungs of the patient with oxygen at the conclusion of nitrous oxide administration 25 Pharmacodynamics of IA Minimum alveolar concentration – MAC – is the minimum alveolar concentration (partial pressure) of IA at 1 atmosphere that prevents skeletal muscle movement in response to a noxious stimulus (surgical skin incision) in 50% of patients In fact that MAC reflects the partial pressure at the anesthetic site of action (Pbr) has made MAC the most important index of anesthetic equal potency MAC values for combination of IA are additive. For example, 0,5 MAC nitrous oxide plus 0,5 MAC isoflurane has the same effect at the brain as either drug alone at a 1 MAC concentration 26 Pharmacodynamics of IA - MAC Clinically, a MAC concentration of IA greater than 1 is necessary, because 50% of patients would move with surgical stimulation at a 1 MAC concentration Administration of approximately 1,3 MAC prevents movement in nearly all patients during surgery In addition to its value as un index of equal potency, the MAC concept also allows a quantitative analysis of the impact of various pharmacologic and physiologic factors on anesthetic requirements 27 Impact of pharmacologic and physiologic factors on MAC – no change in MAC Duration of anesthesia Hyperkalemia or hypokalemia Magnitude of anesthetic metabolism Thyroid gland dysfunction Gender PaCO2 15 mmHg to 95 mmHg PaO2 above 38 mmHg Blood pressure above 40 mmHg 28 Impact of pharmacologic and physiologic factors on MAC – increase MAC Hyperthermia Hypernatremia Drugs that increase CNS catecholamine levels (MAO inhibitors, tricyclic antidepressants, acute cocaine ingestion, acute amphetamine ingestion) Chronic ethanol abuse 29 Impact of pharmacologic and physiologic factors on MAC – decrease MAC Hyperthermia Hypernatremia Pregnancy Lithium Lidocaine α-2 agonists PaO2 below 38 mmHg Blood pressure below 40 mmHg Increased age Preoperative medication Drugs that decrease CNS catecholamine levels (α-methyldopa, clonidine, chronic amphetamine ingestion) Acute ethanol ingestion 30 Nitrous oxide – N2O Colorless, odorless, inorganic gas, sweet taste Noninflammable and nonirritating, but of low potency Very potent analgesic, but not potent anesthetic No effect on HR and respiration Nontoxic to liver, kidney and brain Not potent alone Not good muscle relaxant Inhibits vit B-12 metabolism 31 Halothane Physical properties 1. Halogenated alkane 2. Pungent odor 3. Thymol preservative and amber-colored bottles to retard spontaneous oxidative decomposition 32 Halothane Effects on organs and systems 1. Cardiovascular: ↓ blood pressure, ↓ CO, ↓ myocardial contractility, inhibits baroreceptor-mediated reflex tachycardia, slows SA node conduction, sensitized the heart to the dysrhythmogenic effects of epinephrine 2. Respiratory: rapid shallow breathing, ↓ TV, ↑ RR, depresses hypoxic drive, bronchodilator, depresses mucociliary function 3. Cerebral: ↓CVR, ↑ cerebral blood flow the most, autoregulation impaired, ↓ cerebral metabolic rate the least 4. Renal: renal blood flow decreases 5. Neuromuscular: potentiates relaxants 6. Uterus: myometrial relaxation 7. Hepatic: ↓ hepatic blood flow, hepatic artery vasospasm 33 Halothane Biotransformation and toxicity 1. 20-40% undergoes metabolism 2. Oxidative metabolism via cytochrome P450 enzymes 3. Reductive metabolism (hypoxic conditions) yields fluoride and reactive intermediary metabolites. Increase fluoride concentrations after administration of halothane to obese but not to nonobese patients 4. Toxicity: Halothane hepatitis – allergic reaction vursus familial factor that predisposes patients; two entities: transient mild form and fulminant hepatitis; 1:35000 anesthetics; middle-aged, obese women with repeated exposure to halothane 34 Enflurane Physical properties 1. Halogenated ether 2. Mild, sweet ethereal odor 3. Colorless 35 Enflurane Effects on organs and systems 1. Cardiovascular: ↓ blood pressure, ↓ CO, ↓ myocardial contractility, ↓ SVR, ↑ HR, sensitized the heart to the dysrhythmogenic effects of epinephrine 2. Respiratory: ↓ TV, ↑ RR, depresses hypoxic drive, bronchodilator, depresses mucociliary function 3. Cerebral: ↑ cerebral blood flow, ↑ ICP, in the presence of hypocapnia, deep enflurane anesthesia has been associated with high-voltage, fast-frequency EEG changes – seizures, ↓ cerebral metabolic rate unless there is seizures activity 4. Renal: ↓ renal blood flow, ↓ urine output 5. Neuromuscular: potentiates relaxants 6. Uterus: myometrial relaxation 7. Hepatic: ↓ hepatic blood flow 36 Enflurane Biotransformation and toxicity 1. Minimal oxidative metabolism 2. Elimination primarily by ventilation 3. Most important metabolite is fluoride 4. Serum fluoride concentrations are higher in obese patients 5. Toxicity: Fluoride-induced nephrotoxicity: inability to concentrate urine, after prolonged administration, may have transient decrease in urine- concentrating ability 37 Isoflurane Physical properties 1. Halogenated ether 2. Mild, sweet ethereal odor 3. Colorless 38 Isoflurane Effects on organs and systems 1. Cardiovascular: ↓ blood pressure, mild β-adrenergic stimulation, ↓ SVR, ↑ HR 20% above awake levels (more likely to occur in young patients), least depression in CO, coronary steal syndrome, normal coronary arteries are dilated 2. Respiratory: irritant to upper airways, depresses hypoxic drive, good bronchodilator, depresses mucociliary function 3. Cerebral: Smallest ↑ cerebral blood flow, ↓ cerebral metabolic rate the most, autoregulation is better preserved with isoflurane 4. Renal: ↓ renal blood flow, ↓ urine output 5. Neuromuscular: potentiates relaxants 6. Uterus: myometrial relaxation 7. Hepatic: ↓ hepatic blood flow, hepatic artery perfusion is better maintained with isoflurane 39 Isoflurane Biotransformation and toxicity 1. Insignificant oxidative metabolism 2. Elimination primarily by ventilation 3. Metabolites are trifluoroacetic acid and difluromethanol. Difluromethanol (unstable) − formic acid + fluoride 4. Serum fluoride concentration is minimal 40 Desflurane Physical properties 1. Fluorinated methyl-ethyl ether 2. Pungent odor – not recommended for mask induction 3. Colorless 4. Low solubility – more rapid wash-in and wash-out 41 Desflurane Effects on organs and systems 1. Cardiovascular: ↓ blood pressure, ↓ SVR, ↑ HR, ↑ CVP, no coronary steal syndrome, no sensitization of myocardium to epinephrine-induced dysrhythmias 2. Respiratory: irritant to upper airways, ↓ TV, ↑RR, ↑PaCO2 3. Cerebral: ↑ cerebral blood flow, ↑ ICP, hyperventilation ↓ ICP 4. Renal: no reports of nephrotoxicity, no change in serum creatinine or blood urea nitrogen 5. Neuromuscular: potentiates relaxants 42 Desflurane Biotransformation and toxicity 1. 0,02% metabolized 2. Resists degradation by the liver 43 Sevoflurane Physical properties 1. Fluorinated methyl isopropyl ether 2. Pleasant odor 3. Colorless 4. Smooth mask induction 44 Sevoflurane Effects on organs and systems 1. Cardiovascular: ↓ blood pressure, ↓ SVR, ↑ HR > 1,5 MAC, no depression in CO, ↓ baroreceptor response, do not predispose the HR to ventricular dysrhythmias 2. Respiratory: ↓ TV, ↑ RR non-irritant to upper airways, depresses hypoxic drive, good bronchodilator, depresses mucociliary function 3. Cerebral: ↑ cerebral blood flow, ↓ cerebral metabolic rate, ↑ CBF, ↑ ICP, epileptic activity on the EEG 4. Renal: ↓ renal blood flow, ↓ urine output 5. Neuromuscular: potentiates relaxants 6. Uterus: myometrial relaxation 7. Hepatic: ↓ hepatic blood flow 45 Malignant hyperthermia MH is a life-threatening clinical syndrome of hypermetabolism involving the skeletal muscle (large quantities of calcium to be released from the sarcoplasmic reticulum) It is triggered by the IA and succinylcholine The hypermetabolism causes ↑ CO2, metabolic and respiratory acidosis, accelerated oxygen consumption, heat production, activation of SY nervous system, hyperkalemia, DIC, and multiple organ dysfunction and failure Early clinical signs: ↑ end-tidal CO2, tachycardia, muscle rigidity, tachypnea, hyperkalemia Later signs: fever, myoglobinuria, and multiple organ failure 46 Features of ideal volatile anesthetic Not disturbing smell Fast acting Low solubility in blood – fast transport to brain Stable when stored, not reacting with other chemicals Not flammable, not explosive Low metabolism, fast elimination, no cumulative effect No depressing effect on circulatory and respiratory systems 47 Endotracheal intubation Placement of a flexible plastic tube into the trachea to: maintain an open airway, serve as a conduit through which to administer certain drugs. Is performed in critically injured, ill or anesthetized patients: to facilitate ventilation of the lungs, including mechanical ventilation, to prevent the possibility of asphyxiation or airway obstruction. 48 Endotracheal intubation - indications For supporting ventilation in patient with pathologic disease: upper airway obstruction, respiratory failure, loss of consciousness For supporting ventilation during general anaesthesia: operative site near the airway, thoracic or abdominal surgery, prone or lateral surgery position, long period of surgery Patient has risk of pulmonary aspiration Difficult mask ventilation 49 Airway assessment Conditions that are associated with difficult intubation: congenital anomalies , infection in airway , tumor in oral cavity or larynx, enlarge thyroid gland , maxillofacial, cervical or lar yngeal trauma, temporomandibular joint dysfunction, burn scar at face and neck, morbidly obese or pregnancy Interincisor gap: normal more than 3 cms Mallampati classification: Class 3, 4 may be difficult intubation Thyromental distance: more than 6 cms Flexion and extension of neck Movement of temperomandibular joint 50 Laryngoscopic view Cormack-Lehane system classifies views obtained by direct laryngoscopy Grade 3,4 risk for difficult intubation! 51 Endotracheal intubation - preparing Essentials that must be present to ensure a safe intubation! They can be remembered by the mnemonic SALT Suction. This is extremely important. Often patients will have material in the pharynx, making visualization of the vocal cords difficult. Airway. The oral airway is a device that lifts the tongue off the posterior pharynx, often making it easier to ventilate a patient via mask. The inability to ventilate a patient is bad. Also a source of O2 with a delivery mechanism (ambu- bag and mask) must be available. Laryngoscope.This lighted tool is vital to placing an endotracheal tube. Tube. Endotracheal tubes are in many sizes. In the average adult a size 7.0 or 8.0 oral endotracheal tube will work just fine. 52 Endotracheal intubation – oral airway 53 Endotracheal intubation - laryngoscope 54 Laringoscopic brade Essentials that must be present to ensure a safe intubation! Macintosh (curved) and Miller (straight) blade Adult : Macintosh blade, small children : Miller blade Miller blade Macintosh blade 55 Endotracheal intubation - tecnique Sniffing position Flexion at lower cervical spine Extension at atlanto-occipital joint 56 Endotracheal intubation - preparing Topical Anesthesia: Anesthetize the mucosa of the oropharynx, and upper airway with lidocaine 2%, if time permits and the patient is awake. Direct Laryngoscopy: 1. Place the patient in the sniffing position. 2. Check the laryngoscope and blade for proper fit, and make sure that the light works. 3. Make sure that all materials are assembled and close at hand 57 Endotracheal intubation - curved blade technique Open the patient's mouth with the right hand Grasp the laryngoscope in the left hand Spread the patient's lips, and insert the blade between the teeth, being careful not to break a tooth. Pass the blade to the right of the tongue, and advance the blade into the hypopharynx, pushing the tongue to the left. Lift the laryngoscope upward and forward, without changing the angle of the blade, to expose the vocal cords. 58 Curved blade technique The anesthesiologist then takes the endotracheal tube, made of flexible plastic, in the right hand and starts inserting it through the mouth opening. The tube is inserted through the cords to the point that the cuff rests just below the cords Finally, the cuff is inflated to provide a minimal leak when the bag is squeezed 59 Straight blade technique Follow the steps outlined for curved blade technique, but advance the blade down the hypopharynx, and lift the epiglottis with the tip of the blade to expose the vocal cords. The tip of the laryngoscope blade fits below the epiglottis, which is no longer visible with the blade in position. 60 Endotracheal intubation - complications 1. Tube malpositioning (esophageal intubation ) 2. Tube malfunction or physiologic responses to airway instrumentation 3. Trauma such as tooth damage, lip/tongue/mucosal laceration, sore throat, dislocated mandible 4. Mucosal inflammation and ulceration and excoriation of nose can occur while the tube is in place 5. Laryngeal malfunction and aspiration, glottic, subglottic or tracheal edema and stenosis, vocal cord granuloma or paralysis during extubation Physiologic responses to intubation include hypertension, tachycardia, intracranial hypertension, and laryngospasm 61 Thank you! 62