Aircraft Materials - Ferrous (UTT 2015-10-).PDF
Document Details
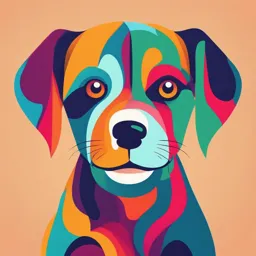
Uploaded by GrandGallium
The University of Trinidad and Tobago
2015
UTT
Tags
Summary
These lecture notes from the University of Trinidad and Tobago (UTT) cover the characteristics and properties of ferrous materials, including fatigue, strength, and toughness. Topics such as elasticity, ductility, malleability, and hardness are discussed, along with testing methods. The course is part of an aeronautical and airworthiness engineering program.
Full Transcript
**BSc IN AERONAUTICAL AND** **AIRWORTHINESS ENGINEERING** **THE UNIVERSITY OF TRINIDAD AND TOBAGO** Administration Building UTT O'Meara Campus Lots \# 74 -- 98 O'Meara Industrial Estate, Arima Mail: P.O. Bag 724, Couva Telephone: (868) 642-8888/223-4888 Fax: (868) 643-1617 Website: www.utt.e...
**BSc IN AERONAUTICAL AND** **AIRWORTHINESS ENGINEERING** **THE UNIVERSITY OF TRINIDAD AND TOBAGO** Administration Building UTT O'Meara Campus Lots \# 74 -- 98 O'Meara Industrial Estate, Arima Mail: P.O. Bag 724, Couva Telephone: (868) 642-8888/223-4888 Fax: (868) 643-1617 Website: www.utt.edu.tt **Aircraft Engineering Materials and Hardware Ferrous and Composites-- BAAE 2010** 1. **AIRCRAFT MATERIALS - FERROUS** **WEEK -- 1 - 3** 1. **AIRCRAFT MATERIALS - FERROUS** **TOPICS:** 1. **Characteristics and Properties of Materials** 2. **Testing of Metals** 3. **Practical Test (Ferrous Metals)** 1. **Characteristics and Properties of Materials** **Introduction** **FATIGUE** This is a progressive localized structural damage. It occurs in materials subjected to repeated or fluctuating strain and stresses having a maximum value which is less than ultimate strength of the material. **STRENGTH** The ability of a material to withstand forces which tend to deform it. Also the ability to withstand stress without breaking. **TOUGHNESS** The property a material possess of being flexible. Tough material is capable of withstanding hard use but is not easily machined and is capable of being bent without fracture. Toughness is measured by an impact tester. **HARDNESS** The property a material possess to resist surface abrasion. It is determined by the resistance to penetration by an indenter which is harder than the material. Some hardness test are: Brinell Rockwell Vickers Diamond Pyramid **DUCTILITY** The property which allows a material to be drawn into a thinner section (wire) without breaking. Eg. Stretching chewing gum. A ductile material can be cold worked. (can be drawn at room temperature) The measure of ductility is given as a percentage elongation in a tensile test. Materials possessing high ductility are steel, copper, nickel, brass, gold, tungsten, aluminum, silver and platinum. Ductility is measured by a bend test. **MALLEABILITY** This is the property a material possess which enables it to be mechanically deformed by compressive forces during rolling, hammering, extruding, etc without fracture or work hardening. **ELASTICITY** The property a material possess of being able to return to its normal shape and size when a deforming force is removed. It is a measure of the stiffness or rigidity of a material. All material has an elastic limit. If this is exceeded permanent deformation results. **IMPACT TOUGHNESS** The property a material possess to absorb a shock load without fracture. It is determined by the IZOD or NOTCHED BAR TEST. Impact failures are more likely to occur at low temperatures except in the cases of aluminum, nickel and copper alloys. **BRITTLENESS** This is the property a material possess of fracturing without out distortion occurring under a load. It shows a lack of ductility in the material and when an impact test is carried out, low readings are recorded. It is often referred to as "Shortness" and a material which is "Hot Short" is brittle at elevated temperature whereas a "Cold Short" material is brittle at low temperatures. E.g. Cast iron is a cold short material and alpha brass is a hot short material. **PLASTICITY** This is the property a material possesses of undergoing permanent deformation in the solid state without fracture. It is the extreme opposite of elasticity. Materials possessing high plasticity are lead, copper and gold, all of which can be easily fabricated into complicated shapes. **DURABILITY** The ability of the airframe to resist, cracking, corrosion, thermal degradation, delamination, wear and the effects of foreign object damage for a specified period of time. The most important mechanical property of a metal is the amount by which it can be deformed before it breaks. A malleable metal is one which can be deformed a great deal by compression before it shows signs of cracking. Malleable metals can be rolled, forged or extruded. Since these are all processes where the metal is shaped under pressure. Malleability usually increase with temperature, so processes involving pressure are usually hot working processes i.e. they are carried out on heated pieces of metal. A ductile metal is one which can be deformed a great deal by tension before it fractures. While all ductile metals are malleable, it does not mean that malleable metals are ductile. Some metals although soft, are also weak in tension and tear apart while being stretched. The ductility of all metals increases as the temperature rises, because they are weaker at high temperatures. In the various hardness tests a compressive force is used to make a small dent in the surface of a test piece and a comparison of hardness values made. The toughness of a material and its ability to resist mechanical shock is measured by an impact test. The ductility of a material is usually found by means of a bend test. In these tests the test piece is destroyed during the testing process. These tests are therefore known as destructive tests and can only be applied to individual test pieces. These are taken from a batch of material after manufacture and are assumed to be representative of the batch. The SI units applicable to the testing of metallic material are as follows: MASS : KILOGRAM (kg) FORCE : NEWTON (N) STRESS : NETONS/SQ. METER (N/M2) WORK, ENERGY : JOULE (J) DIMENSIONS : METRE (M) **Materials can be divided into the following groups shown below.** 1. **Ferrous Metals** **[IRON AND STEEL]** **INTRODUCTION** Iron is a very important metal in engineering. Little used in its pure form it is however the main constituent of steel. Iron ore (magnetite and hematite) is quarried or mined from the earth's crust and converted by a series of processes into pig iron. IRON PRODUCTION Pig iron is produced by smelting iron ore in a blast furnace similar to that illustrated in the Fig. below. The iron ore is first washed and then added to the blast furnace together with high quality coke, low in sulphur content and limestone, (which combines with impurities and forms a slag). During charging, the double bell arrangement prevents gases escaping. These gases are later cleaned and used to heat the air entering the blast main, to about 800oC, reducing the amount of coke required by half. The oxygen in the air causes the coke to burn fiercely, generating heat and [producing carbon monoxide gas which reduces the ore to metal]. Iron, which is the heaviest product drips to the bottom of the furnace. The lighter slag floats on top of the iron. When the iron reaches the level of the slag tapping hole, the slag is run off. The iron is then tapped out from the bottom of the furnace. The molten pig iron may be cast into small moulds known as pigs. If the blast furnace is situated close to a steel works, the molten iron is conveyed direct to the steel making furnaces. **CAST IRON** Pig iron from the blast furnace is not refined enough for making castings. In a foundry the pig iron is re-melted together with broken pieces of pig iron, coke and some limestone (to absorb impurities) in a small blast furnace called a cupola. The molten iron, having a melting point of 1130 ⁰C, flows to the bottom of the furnace and is tapped directly into ladles or moulds. Cast iron is an important material for the following reasons:- 1\. It is a cheap metal, since it can be produced by simple adjustments to the composition of ordinary pig iron. 2\. Rigidity and strength under compression are good. 3\. It is easy to machine. 4\. It casts easily due to its fluidity when molten. 5\. Its composition can be altered to make it stronger and tougher if required. Iron is prepared by a reduction process (Iron ore reduced by carbon monoxide ore). Steel is prepared by an oxidation process (addition of oxygen to the pig iron to purify it). (**Note**: **cast iron is also brittle and heavy**). **THE COMPOSITION OF CAST IRON** Ordinary cast iron contains the following elements:- Carbon 3.0 to 4% Silicon 1.0 to 3.0% Manganese 0.5 to 1.0% Sulphur up to 0.1% (Induce Brittleness) Phosphorus up to 1.9% Also Chromium 0.5 -- 1.0% for Stainless Steel **WROUGHT IRON** Probably the first form of iron used by man. [It is very pure, containing 99.9% iron]. It is made from oxidising nearly all the carbon and other elements from pig iron in a furnace. Wrought iron is able to take large shock loads without permanent damage and was used for making chains and lifting hooks, etc. Its fibrous nature gives visible warning on the surface, if it is about to break. **STEEL (OXIDATION PROCESS)** Steel is made from pig iron, but in modern steel making, large amounts of scrap steel can be used up if required. There are three methods of making steel - The Open Hearth Process, the Basic Oxygen Process and the Electric Furnace Process. **OPEN HEARTH PROCESS** In the Open Hearth Process, the heat required to melt the pig iron and scrap is obtained by burning a mixture of gas and air over the metal. The gas and air is pre-heated to a high temperature in regenerators. Lime is added to the charge, in order to form a basic slag and so remove the phosphorus which is present in most pig irons. The slag floats on the surface of the molten steel and is run off. Carbon is then added in the form of anthracite which dissolves in the molten steel. Finally the molten steel is transferred to a large ladle which in turn pours the steel into mould to produce ingots each of several tones mass. BASIC OXYGEN STEELMAKING A typical basic oxygen furnace consists of a steel cased converter lined with dolomite holding up to 400 tones of metal. The charge consists of up to 40% of scrap steel, lime and molten pig iron. Oxygen is then blown at the surface of the molten charge from a water cooled 'lance'. This is lowered through the mouth of the converter to within 0.5 metres of the surface of the metal. The impurities in the metal are oxidised during blow which lasts about fifteen minutes. During this time the temperature is carefully controlled and the molten metal analysed. When the temperature and metal analysis are satisfactory, the hood is lifted, the converter tilted and steel poured from below the slag into the ladle. **ELECTRICAL ARC STEEL MAKING** An electrical arc furnace, The heat required to melt the charge is produced by electric arcs struck between carbon electrodes and the steel. The impurities are oxidised from the charge by melting it under a covering of slag which absorbs the oxidised impurities and may then be run off by tilting the furnace. The charge consists of scrap, iron ore, blast furnace pig iron and limestone. The advantage of the electric furnace is that there is no gas, fumes or impurities which are present in fuel fed furnaces and which may allow impurities into the molten metal. Electrical furnaces are used to make high quality steels. NOTE:-When the impurities are oxidised, they combine with oxygen and form an oxide which floats on top of the molten steel, together with the slag. **GRAIN STRUCTURES** **ATOMS AND MOLECULES** All matter is made up of atoms. Matter is anything which occupies space and has weight. A material which is made up of just one type of atom is called an element. Copper and iron are examples of elements. Each atom is composed of a nucleus, which is positively charged, and electrons which are negatively charged. The atom can be considered of consisting of the nucleus, which makes up most of the mass of the atom, surrounded by a cloud of electrons, which orbit the nucleus. The Atom The term molecule is used to describe groups of atoms which tend to exist together in a stable form. For instance, hydrogen tends to exist in a stable form as a combination of two hydrogen atoms rather than individual atoms. Some molecules may exist as combinations of atoms from a number of different elements. Water for example, consists of molecules each of which is made up of two hydrogen atoms and one oxygen atom (H2O). **THE ATTRACTION OF ATOMS** The graph below shows the basic form of a force-separation graph which we can use to describe the behaviour of atoms. It shows that as atoms are pulled apart, there is an attraction force which tries to pull them back. This force reaches a maximum at a certain point, after which the attraction force deteriorates. If however, the atoms are forced together, there is a repulsive force. This is fortunate, since without this force there would be nothing to stop the attractive forces pulling atoms, or molecules closer and closer together until eventually all the matter vanished into a very small point. **BONDS** The way in which atoms join together, or molecules join together, is called bonding. The following are the different types of bonding that can occur; the type of bonding existing within a material determines many of its properties. **Ionic Bonding** An individual atom is electrically neutral, having as much positive charge as negative charge. However, if an atom loses an electron (a packet of negative charge), it ends up with a net positive charge. It is then referred to as an ion -- in this case a positive ion. If an atom gains an extra electron, it ends up with a net negative charge, becoming a negative ion. In an ionically bonded material, an atom of one element gives an electron to an atom of another element. One element then consists of positive ions, the other of negative ions. Unlike charged bodies attract each other; therefore the two elements are strongly attracted to each other. **Covalent Bonding** The electrons in an atom can be considered to rotate orbit around the nucleus similar to the moon orbiting the earth. In this type of bonding, atoms share one or more of the electrons since the electrons will orbit both nuclei in a figure eight pattern. Oxygen is a typical example of this, where two oxygen atoms are bonded together by two of their electrons being shared. **Electron Cloud/Metallic Bonding** In a metal, such as copper, we have atoms freely losing electrons and becoming positive ions. The electrons that have been lost (in the case of copper, one electron is lost from each copper atom) do not combine with any ion but remain as a cloud of negative charge floating between the ions. The result is bonding, this form of bonding often being referred to as metallic bonding since it is typical of metals. The free electrons explain why metals are such good conductors of electricity. **CRYSTAL (OR GRAIN) STRUCTURE** When a metal is in the molten state, the atoms are free to move and there is no logical arrangement of the atoms. As the molten material cools into a solid, the atoms layer themselves into a structure as shown below; there are many different forms and patterns of the layering, (that shown below is one of the simplest. Metals are crystalline substances. This may seem a strange statement in that metals do not generally look like crystals, with their geometrically regular shapes. The photograph below shows a magnification of copper sulphate in a semi-molten state. It shows clearly the crystals forming within the solution. The photograph below shows the crystal structure of aluminium CRYSTAL (OR GRAIN) STRUCTURE The term grain is used to describe the crystals within the metal. A grain is merely a crystal without its geometrical shape and flat faces because its growth has been impeded by contact with other crystals. Within a grain the arrangement of particles is just as regular and repetitive as with a crystal with a smooth surface. The grains on the surface of a metal are not generally visible. They can be made visible by careful etching of the surface with suitable chemical. **THE FORMATION OF METAL GRAINS** As a metal cools from a molten state, crystallization occurs around tiny impurities in the molten metal. As more and more atoms build up on top of the crystals in a regular pattern, the growing crystals change into a "tree-like" growth called a **dendrite**. The dendrites grow so large their arms collide with the arms of other dendrites, and so more growth can take place in that direction. The spaces between the arms of the dendrite then begin to fill in, until the whole metal has solidified. **THE DEFORMATION OF METALS** **Elastic Deformation**: is the term applied when a metal undergoes a stress which deforms it, but when the stress\` is removed, the metal returns to its original size and shape. **Plastic Deformation**: is the term applied when a metal undergoes a stress which deforms it, but when the stress is removed, the metal does not return to its original shape. **WHAT HAPPENS TO METALS WHEN PLASTIC DEFORMATION OCCURS?** Metals are crystalline. This means that the metal atoms are arranged in a regular manner within the crystal. This regular array of atoms enables us to consider the atom in a crystal as lying in a number of planes. The different planes have different densities of atoms and different spaces between parallel, like, planes. When plastic deformation occurs we can consider planes of atoms to be sliding past each other. This plane along which this movement occurs is called the slip plane. The slip plane tends to occur between those parallel planes with the highest atomic density and the greatest separation. Slip is also most likely to occur between planes which contain dislocations. Slip only occurs in those planes which are suitable angles to the applied stress. The result is that the surface of the metal shows a series of steps due to the different movements of the various plane atoms. The slip lines do not cross over from one grain to another. Thus the bigger the grain, the more slippage can occur. **A large grained material is generally more ductile than a small grained material**. The smaller the grains, the stronger the material. **COLD WORKING AND WORK HARDENING** The term cold working is used to describe any plastic deformation of a metal at room temperature. Types of Cold Working: **Forging** -- beating with a hammer into shape. **Drop forging** -- a mould or template to form the material. **Cold rolling** -- solid metal passing through some metal via a roller or machine until desired shape or thickness is reached. As the amount of plastic deformation increases, so the tensile strength increases. The material becomes harder as a result of cold working and this is called work hardening. The following table shows some of the changes that take place when a sheet of annealed aluminium is rolled and its thickness reduced. Reduction in Tensile Strength Elongation Hardness ------------------- ------------------ ------------ ---------- Sheet thickness % N/m \% HV 0 92 40 20 15 107 15 28 30 125 8 33 40 140 5 38 60 155 3 43 It can be appreciated from the above table that after about 60% reduction in thickness the material will be too brittle to be useful. THE STRUCTURE OF COLD WORKED METALS When stress is applied to a metal grain deformation starts along the slip planes most suitably orientated. The effect of this is to cause the grains to become elongated and distorted. Two of the reasons for the increase in strength and hardness during cold working are: 1\. Inability of slip planes to move any further because they have hit other grains. 2\. Dislocations slip to an area where they are unwanted and other atoms are forced apart to make room for them. This results in "locked up" internal stresses. **THE HEAT TREATMENT OF WORK HARDENED METALS (ANNEALING)** The work hardening of a metal is usually an advantage (such as the riveting process) but sometimes during forming a metal component, the metal becomes over hard and brittle before the component has been fully formed, and further working results in cracking of the metal. Also, it is known that the internal stresses described in part 2 above reduce the materials resistance to corrosion (stress corrosion). **The Relief of Stress** This often takes place at relatively low temperatures at which atoms nevertheless are able to move small distances into positions where they will incur less strain. There is however, no observable alteration in the distorted structure which was produced by cold working and both tensile strength and hardness will remain at a high level. Low temperature annealing which does not cause recrystallisation and which consequently does not lead to a fall in strength and stiffness engendered by cold work, is useful in dissipating local strain which might otherwise give rise to stress corrosion. **Recrystallisation** -- The situation of distorted crystals can be reversed by heating further than that required for stress relief. As the annealing temperature increases, a point is reach where new crystals begin to grow from nuclei initiated within the most heavily deformed regions. These nuclei form at points of high energy such as crystal boundaries and other localities rich in piled-up and entangled dislocations. The new crystals so formed are very small at first but grow steadily until they have absorbed the whole of the distorted structure. The new crystals do not show any directionality as did the distorted crystals which they replace. The recrystallisation temperature varies between metal types but for (Tin-melting temperature) aircraft metals is generally 0.3Tm -0.5Tm. The greater the percentage impurities and alloying elements, the greater is the recrystallisation temperature. Pure lead recrystallises at below room temperature, therefore it is impossible to work harden it. **Grain Growth** At temperatures above that of recrystallisation of the metal, the newly formed crystals will tend to grow by absorbing each other in cannibal fashion. **Residual Stress** is the stress that cannot be removed and is left back. **STEEL** Plain carbon steel are alloys of iron and up to 1.7% carbon. Most ordinary steels also contain up to 1.0% manganese, left over during the steel making process. The manganese helps reduce the sulphur content of the steel. Both sulphur and phosphorus are harmful impurities causing brittleness in steels. Most specifications allow no more than 0.06% of either of these elements. High quality steels would contain no more than 0.04% of each element. The manganese dissolves in the solid steel, slightly increasing its strength and hardness. The relative density of steel is 7.9 **GENERAL** Plain carbon steel owes its properties to the presence of carbon. The effects of carbon in iron are best understood by first considering pure iron. Pure iron is very ductile and has a tensile strength of 18 tons/sq.in (28 Hbar). The addition of carbon to the iron increases the tensile strength, hardness and brittleness and at the same time decreases the ductility. The tensile strength of 0.87% carbon steel is a approximate 55 tons/sq.in (85 Hbar). The increase in strength of steel will increase in carbon content in limited to the amount of carbon which will remain combined with the iron after any normal heat treatment. Above 1.7% carbon, the excess carbon is in the form of free (uncombined) carbon or graphite, which has very low strength. The carbon content of plain carbon steel does not often exceed 1.5%. The internal structure of steel may be varied by heating and cooling. To produce certain properties in the steel, it is heat treated. It is not always possible to produce all the desired properties in the metal and a compromise has to be made. For ease of manufacture the metal may require to be soft. In use, great strength and considerable hardness may be required. Thus if a steel is required to be hard and tough, the maximum degree of hardness and toughness cannot be obtained together. Maximum hardness makes the metal brittle and maximum toughness generally reduces hardness. **MICRO-STRUCTURE** The constituents of carbon steel are [pure iron (ferrite)], [and a chemical compound of carbon in iron called iron carbide (cementite]). In low carbon steel, these constituents, when viewed through a microscope, appear as a laminated structure (pearlite), surrounded by free ferrite. With increasing carbon content, the proportion of pearlite to ferrite increases until, with 0.87% carbon content, the steel is entirely pearlite. Above 0.87% carbon content, a microscopic examination shows pearlite surrounded by free cementite. Ferrite is soft, ductile and not very strong. Pearlite is strong and tough, but soft enough to be worked. Cementite is very hard and brittle. As the carbon content is increased up to 0.87% the steel gets tougher and stronger. When the carbon content exceeds 0.87%, increasing cementite content, the steel becomes very hard and more brittle. **MICRO-STRUCTURE CARBON STEELS** When steel is heated, the following changes will occur to the microstructure:- The temperature at which the change starts (lower critical point) is the same for all steels and is about 723 ⁰C. At this temperature, the pearlite disappears and the ferrite and cementite of which it is composed, dissolves and forms a solid solution known as austenite which is non-magnetic. The finishing point of the transformation (the upper critical point) varies according to the steel. In steel containing 0.87% carbon (wholly pearlite) there is only one critical point, the whole transformation taking place at about 723 ⁰C. Therefore, steel containing a lower or higher carbon content than 0.87% must be subjected to increase in temperature (upper critical point) to bring the free ferrite or cementite into solid solution. See Fig. 5. - Ferrite --- pure iron (below 0.87% carbon) - Cementite --- iron carbide (above 0.87% carbon) - Pearlite --- a mixture of ferrite and cementite (0.87% carbon) - Austenite ---pearlite in solid solution. Forms at lower critical point. It's non-magnetic. When steel is allowed to cool slowly these changes occur in the reverse order. **[HEAT TREATMENTS]** The following heat treatment methods apply to plain carbon steels only, they do not apply to the alloy steels. Steel should be heated slowly and uniformly. It is preferable to use heating equipment which allows close control of the temperature. Electric muffle furnaces and salt bath furnaces can be used. The steel must remain in the furnace until it is heated right through and this takes time. There are four factors controlling in heat treatment: - The temperature to which the metal is heated. - The time the metal is held at this temperature - The temperature to which the metal is cooled. - The rate at which the metal is cooled. **[ANNEALING]** (Softens metal by heating and then allowing it to be cooled slowly in the furnace) - Stress Relieving - Recrystallization - Grain Growth A steel, in a hardened or tempered condition, containing up to 0.87% carbon, can be restored to its softest state by heating it to its upper critical point, thus bring it to its austenitic condition. The steel is then allowed to cool very slowly by leaving it in the furnace after the source of heat has been removed. The slow cooling allows the normal structural changes to take place and the steel returns to its pearlite and ferrite or pearlite condition. Steels containing more than 0.87% carbon are heated to below the upper critical point. **NORMALISING** (Relieves internal stresses and strain by heating slowly in its upper critical point then allowing it to air cool) When steel is cold worked, hammered, bent, rolled, etc., its crystal structure is distorted. Stresses and strains are set up and the metal may become brittle and weakened. Also when steel is kept for considerable time well above the upper critical point (during forging) an increase in the grain size takes place. When the metal is cool it may be found to have lost its toughness. Normalising restores the crystalline structure to a normal condition and relieves stresses and strains. Normalising is carried out by heating the steel slowly to its upper critical point and then allowing it to cool freely in the air. This more rapid rate of cooling, as compared with the cooling rate in the annealing process, gives the steel a finer grain structure. **HARDENING** (Heating to above its critical point then rapid cooling by water or oil) When high carbon steel is heated to a temperature a little above its upper critical point then cooled by rapid quenching, the normal structural change does not occur. Instead the austenite is changed into martensite which is extremely hard and brittle. This hardening effect only occurs in high carbon steels, attaining its full value in 0.87% carbon steel. The degree of hardness of steel depends upon its carbon content. Steel is normally quenches in water or oil. Oil gives a slower quench which reduce the hardness of the part but makes it tougher and reduces the risk of cracking. **TEMPERING** (Reduces brittleness after hardening) Steel is tempered to reduce the brittleness which is caused by hardening. It is however very important that sufficient hardness is retained by the steel. The steel is reheated to a fairly low temperature, and then quenched in water. The temperature depends on the purpose for the tool. The higher the tempering temperature, the less the hardness but the greater the toughness. Thus the purpose of the tool or article must be considered. The temperature required may be judged from the temper colours which appear on the bright surface of steel which is heated slowly. Each colour indicates a temperature. A short table of articles and suitable temper temperatures is given here. **ARTICLE** --------------------------------- -- ----- Scrapers, scribers Punches, reamers, taps and dies Drills, woodworking tools 260 Cold chisels, screwdrivers Springs Where a large amount of tempering is carried out, ovens or baths, of molten salts having accurate temperature control, give better results. The colour method is used for single tools. **REFINING** Prolonged heating at temperatures well above the upper critical point followed by slow cooling (as in case hardening) causes the grain structure of steel to coarsen. This reduces the toughness and strength of the steel. The refining process has the effect of reducing the size of the crystalline structure. It consists of reheating the steel to between 840oC and 900oC, then quenching either in water or oil. **[SURFACE HARDENING]** (Hardening the surface while retaining a tough, shock resisting core) Very often a component must have a hard, wear resisting surface that is supported by a tough, shock resisting core. This combination of different properties can be obtained in a single piece of steel by surface hardening. Methods of surface hardening are as follows:- - **[CASE HARDENING OR CARBURISING]** In this process the component is usually made from a low carbon steel so that it will not respond to direct hardening treatment. The component is case hardened or carburised to increase the carbon content of the surface to about 0.83%. This part is then heat treated to refine the grain of the core and harden the case. The process is carried out at a temperature of about 900^o^C to 950^o^C. The depth of the case will depend on the time held at this temperature. - **[THE BOX PROCESS]** The parts are thoroughly cleaned and packed in a steel box along with a material rich in carbon. The lid is sealed to prevent gas escaping during carburising and to prevent gases from the furnace entering. The box is placed in the furnace and heated to a temperature of 900^o^C. This temperature is maintained for sufficient time to give the required thickness of case. Carburising at this temperature for four hours gives a case approximately one millimetre thick. This permits final machining of the part. The parts are allowed to cool down slowly in the box in order to anneal the newly formed case. The grain of the core is refined by heating to a temperature of about 850^o^C then quenching in water or oil. Finally the case is hardened by heating the part to a temperature of about 750^o^C, then quenching in water or oil. Surfaces of the part not requiring case hardening can be protected by electro-plating these areas with copper. - **[CYANIDE HARDENING]** The part is placed in molten salts consisting mainly of sodium cyanide at a temperature of approximately 900^o^C, which provides both the necessary heat and the material for carburising. This process is used where a hardened layer of 0.1 to 0.25 mm may be sufficient. Articles carburised by this method are then subjected to the normal refining and hardening heat treatment. **Note:** that sodium cyanide is deadly poisonous and strict safety precautions must be observed in workshops where it is used. - **[NITRIDING]** This is a process in which certain alloy steels assume an intensely hard surface when heated in an atmosphere rich in ammonia gas. These steels, generally known as nitriding steels, contain aluminium and chromium. The process consists of heating the pats in a box at a temperature of 500^o^C, and passing a steady stream of dry ammonia gas through the box for a period of 10 to 90 hours. During this time the steel absorbs nitrogen from the ammonia gas. The hardened layer produced is from 0.15mm to 0.8mm thick. The fairly low temperature of the process and because there is no need to quench the parts, means there is less chance of the parts being distorted. Nitriding is used to harden the bearing surfaces of piston engine crankshaft. It is also used to harden the cylinders of piston engines. Any areas of the surface which are required to be left soft are protected by coating with tin or by nickel plating. **[ALLOY STEEL]** Alloy steels are combinations of iron and carbon with some other element or elements added in varied proportions. Such additional elements give the steel specific properties, unobtainable to the same degree in plain carbon steel. To develop the desired properties, more varied heat treatment is required. This may require the use of special equipment and skills. Alloy steels are used for the following purposes:- a\) When it is desired that the steel should have great strength, resistance to wear, springiness or resistance to corrosion. b\) To obtain mechanical properties by less drastic heat treatment than would be required in a plain carbon steel. c\) To enable the effect of heat treatment to penetrate uniformly throughout a large mass of steel. The most common of these alloying metals are:- **NICKEL** -- With varying percentage of nickel, the following effects are produced:- a\) Prevents grain growth -- used in case hardening steels. b\) Increases strength and toughness. c\) Improves resistance to corrosion. d\) Lowers the critical temperatures so that hardening requires a less severe quench. e\) With 25% nickel added, the steel is austenitic at room temperature and thus non-magnetic. f\) With 35% nickel added, the steel will have a coefficient of expansion of nearly zero. g\) A higher percentage of nickel gives higher magnetic permeability than obtained with soft iron. **CHROMIUM** a\) Increases hardness and strength. b\) Increases rate of grain growth, so nickel, which has the opposite effect, is often added with chromium to control the grain size. c\) Increases corrosion resistance, used to make ball and roller bearings. **NICKEL/CHROME STEELS** a\) Low nickel/chrome steels (3% Nickel/1% Chromium) are used for piston engine crankshafts, connecting rods and similar applications. b\) High nickel/chrome steels (18% Chromium/8% Nickel) are the stainless steels. **MANGANESE** a\) Increases strength, toughness, shock and wear resistance. b\) High Manganese steels (about 12% manganese) are very soft when produced but any cold work by rubbing, cutting, etc., causes the surface to become very hard and wear resistant. Used for rock crusher parts. **VANADIUM** -- Percentage required is very low. a\) Increases tensile strength and elastic limit. b\) Increases shock resistance and fatigue resistance. Used for valve spring, dies. **COBALT** a\) Used in the production of cutting tool materials. b\) Used in the production of permanent magnets. **MOLYBDENUM** -- Percentage required is very low. (crank shaft for piston engine) a\) Increases resistance to creep at high temperatures. b\) Increases tensile strength and ductility. c\) Prevents brittleness experienced by some nickel/chrome steels when they are heated. **TUNGSTEN** a\) Improves heat and wear resistance. b\) Used in the production of many cutting tool materials. Tungsten Steel is used in undercarriages, engine mountings, joint plates (at wing roots etc.) door latches, for bolts and fasteners etc., where high strength is required. Steel is also used to make many engine parts. **NOTE: -** Metals which contain mostly iron are known as Ferrous Metals. Other metals are known as Non-ferrous. **[IRON AND STEEL]** **Ferrous** metals are those whose principal content is iron (Latin, *ferrum*), such as cast iron, steels and similar products. Because of the vast number of different steels and steel alloys, we shall not attempt to describe more than a few of the more commonly used types. A large percentage of the steels used for general aircraft work are of the wrought type and are designated as shown in Table 1. In addition to the standard group of wrought-carbon and alloy steels, a substantial number of heat- and corrosion-resistant steels are used in aircraft and missiles. The principal designations for these steels are given in Table 2. In Table 1, the first digit of each number indicates the general classification of the steel, that is, carbon, nickel, etc. The number 1 indicates a carbon steel. The second digit of the number indicates the approximate percentage of the principal alloying element; for example, a 2330 steel contains more than 3 per cent nickel. The last two digits of the number indicate the approximate amount of carbon in hundredths of 1 percent. **TABLE 1 SAE Identification for Wrought Steels** **Carbon steels** 10xx Nonsulfurized carbon steel (plain-carbon) 11xx Resulfurized carbon steel (free machining) 12xx Resulfurized and rephosphorized carbon steel **Alloy steels** 13xx Manganese 1.75% (1.60-1.90%) 23xx Nickel 3.50% 25xx Nickel 5.0% 31xx Nickel-chromium (Ni 1.25%, Cr 0.65%) 32xx Nickel-chromium (Ni 1.75%, Cr 1.00%) 33xx Nickel-chromium (Ni 3.50%, Cr 1.50%) 40xx Molybdenum 0.25% 41xx Chromium-molybdenum (Cr 0.50 or 0.95%, Mo 0.12 or 0.20%) 43xx Nickel-chromium-molybdenum(Ni 1.80%,Cr 0.50 or 0.80%, Mo 0.25%) 46xx Nickel-molybdenum (Ni 1.75%, Mo 0.25%) 47xx Nickel-chromium-molybdenum (Ni 1.05%, Cr 0.45%, Mo. 0.20%) 48xx Nickel-molybdenum (Ni 3.50%, Mo. 0.25%) 50xx Chromium 0.28 or 0.40% 51xx Chromium 0.80, 0.90, 0.95, 1.00 or 1.05% 5xxx Chromium 0.50, 1.00 or 1.45%, Carbon 1.00% 61xx Chromium-vanadium (Cr 0.80 or 0.95%, V 0.10 or 0.15%) 86xx Nickel-chromium-molybdenum (Ni 0.55 or 0.05 or 0.65%, Mo 0.20%) 87xx Nickel-chromium-molybdenum (Ni 0.55%, Cr 0.50%, Mo 0.25%) 92xx Manganese-silicon (Mn 0.85%, Si 2.00%) 93xx Nickel-chromium-molybdenum (Ni 3.25%, Cr 1.20%, Mo 0.12%) 98xx Nickel-chromium-molybdenum (Ni 1.00%, Cr 0.80%, Mo 0.25%) **TABLE 2 AISI Identification for Heat- and Corrosion-resistant Steels** 2xx Chromium-nickel-manganese (nonhardenable, austenitic, non-magnetic) 3xx Chromium-nickel (nonhardenable, austenitic, non-magnetic) 4xx Chromium (hardenable, martensitic, magnetic) 4xx Chromium (hardenable, ferritic, magnetic) 5xx Chromium (low chromium, heat-resisting) One of the most important considerations for ordinary carbon steel is the quantity of carbon it contains. Low-carbon steel contains 0.10 to 0.15 percent carbon. Medium-carbon steels contain 0.20 to 0.30 percent carbon. The higher the carbon content of steel, the greater its hardness and also its brittleness. High-carbon steels are used for cutting tools, springs, etc. For general purposes, low or medium steels are best because they are more easily worked, they are tougher and they have a much greater impact resistance. Such steels were used for many years in the manufacture of aircraft structures and fittings. Probably the most commonly used steel for general aircraft structural purposes today is **SAE (Society Automotive Engineering) 4130 chromium-molybdenum (chrome-moly) steel.** When properly heat-treated, it is approximately four times as strong as 1025 mild-carbon steel. SAE 4130 chrome-molybdenum steel is easily worked, readily weldable by any method, hardenable, heat-treatable, easily machined and well adapted to high-temperature conditions of service. The technician should remember the name and number of this steel because it is commonly used for the tubular steel structures of aircraft. Nickel steels, SAE 23xx and 25xx, contain from 3.5 to 5 percent nickel and a small percentage of carbon. The nickel increases the strength, hardness and elasticity of the steel without appreciably affecting the ductility. Nickel steel is used for making various aircraft hardware including nuts, bolts, clevis pins and screws. Nickel-chromium and chromium-vanadium steels are used where still greater strength, hardness and toughness are required. Such steels are often found in highly stressed machine parts such as gears, shafts, springs and bearings. It is not essential that technicians know the type of steel used in a particular factory-made part. However, if they need to fabricate a steel part which is no longer available, they must make sure that it is made from a material as good or better than the original and that it is properly stress-relieved and heat-treated if such treatment is necessary to provide the correct degree of hardness and strength. The nature and temper of the original part can often be determined by means of a Rockwell or Brinnell hardness tester. **[CORROSION-RESISTANT (STAINLESS) STEELS]** Since the 1940s the term **stainless steel,** also designated corrosion-resistant steel (CRES), has become a household word because of its many applications in consumer items as well as in aircraft and missiles. The development of stainless steel has made possible many of the outstanding advances in aircraft, gas-turbine engines and rockets. The most important characteristics of stainless steels are corrosion resistance, strength, toughness and resistance to high temperatures. Stainless steels can be divided into three general groups based on their structures: **austenitic, ferritic and martensitic.** The austenitic steels are chromium (Cr)-nickel (Ni) and chromium-nickel-manganese alloys. They can be hardened only by cold working and heat treatment serves only to anneal them. They are non-magnetic in the annealed condition, although some may be slightly magnetic after cold working. **Austenitic** steels are formed by heating the steel mixture above the critical range and holding to form a structure called **austenite.** A controlled period of partial cooling is allowed followed by a rapid quench just above the critical range. **Ferritic** steels contain no carbon; hence they do not respond to heat treatment. They contain a substantial amount of chromium and may have a small amount of aluminium. They are always magnetic. **Martensitic** steels are straight chromium alloys that harden intensely if they are allowed to cool rapidly from high temperatures. They differ from the two preceding groups because they can be hardened by heat treatment. Has a needle-like structure under a microscope. The most widely used stainless steels for general use are those in the 300 series, called 18-8 because they contain approximately 18 per cent chromium and 8 per cent nickel. Typical of these types are 301, 302, 321 and 347. Although stainless steels have many advantages, there are certain disadvantages that must be faced by the fabricator and designer: (1) Stainless steels are more difficult to cut and form than many materials. (2) Stainless steels have a much greater expansion coefficient than other steels and they conduct heat at a lower rate; this makes welding more difficult. (3) Many of the stainless steels lose their corrosion resistance under high temperatures. In the use of corrosion-resistant steels for aircraft, the technician must assure that the proper type is selected for the part of the aircraft involved. In most cases a damaged part can be replaced by a factory-made part identified by the part number; however, there are situations where it is preferable to repair a part by patching or welding. In these cases, the correct type of corrosion-resistant steel (CRES) must be chosen. In welding CRES, inert-gas arc welding is preferred, because this process causes less deformation due to heat expansion of the metal and it prevents oxidization. The expansion of stainless steel due to temperature increases may be more than twice that of ordinary carbon steels. Because of its toughness, stainless steel is more difficult to cut, form, shear, machine or drill than is ordinary steel. For this reason the technician who is to work with this material successfully must be experienced in the necessary processes or must be directed by an experienced technician. 2. **Testing of Metals** The tensile strength of a material is the stress required to cause fracture of a test piece in tension. The results of a tensile test will give tensile strength, proof stress, and Young's Modulus of Elasticity. A test piece of know cross sectional area is gripped in the jaws of a testing machine. A tensile force is then applied. This is increased by suitable amounts. For each increase of force applied, the amount by which the "gauge length" on the test piece increases, is measured using an extensometer. When the test piece begins to stretch rapidly, the extensometer is removed; otherwise, it may be damaged. The maximum force applied to the test piece before it fractures is measured. Using the results obtained during the test, a stress/strain diagram can be plotted, see Fig. below. Graph of stress against strain for mild steel At first the amount of strain is very small, and is directly proportional to the applied stress, therefore the line is straight. If the stress is released at any point before the curve is reached, the test piece will return to its original length. Thus the extension between the straight-line-area is elastic and within the elastic limit the strain is proportional to the stress producing it. Stress = a constant x strain (divide both sides by strain) Stress = constant E. a constant for the material Strain This constant E is known as Young's Modulus of elasticity for the material and is: E = Stress \[where stress = load /force Strain area Strain is the ratio or fraction:- Strain = Final length -- Original length Original length Its value indicates the ability of the material to resist tensile or compressive loading without deforming. If the test piece is stressed past the point on the graph (known as the elastic limit or limit of proportionality), the material suddenly 'gives' i.e. it shows a sudden extension for very little increase in stress. This is called the yield point, and if the stress is now removed, a small permanent extension will remain in the material. Any extension of the material which occurs beyond this point is of plastic nature. As the stress is increased the material stretches rapidly. At first along its entire length, and then locally to form a neck. Necking occurs just after the maximum stress has been applied at. After this point, the test piece will go on stretching when some of the force has been removed until it breaks at the end of graph line. This is not the real breaking stress, because if the load is divided by the reduced area the graph will show maximum stress at some point past the yield point, i.e. the highest part of the curve on the graph. In practice a nominal value of the tensile strength of a material is calculated, using the maximum force (at the highest part of the curve on the graph) and the original cross sectional area of the test piece, thus:- Tensile strength = maximum force used (ultimate strength) original area of cross section PROOF STRESS The stress/strain diagram in Figs. below is typical of a low carbon steel in the normalized condition. Stress/strain diagrams for high strength steels and for most other alloys do not often show a well defined yield point, and the elastic portion of the curve merges into the plastic section as shown in Figs. below. Figs. above (Typical stress/strain diagrams for both carbon steels and non-ferrous metals) For design purposes it is desirable to know the highest stress that a material can withstand without deformation, and since the graphs do not show a yield point, the yield stress is replaced by a value known as proof stress which will produce a permanent extension of 0.1% in the gauge length of the test piece. Fig. below shows the 0.1% proof stress of a material is derived. The relevant part of the stress/strain diagram is plotted as described previously. A distance equal to 0.1% of the gauge length of the test piece, is marked along the horizontal axis. A line is then drawn from that axis, parallel to the straight line portion of the graph. The line intersects the curve at a point, and this indicates the proof stress which would produce a permanent extension of 0.1% in the gauge length of the test piece. TENSILE TESTING MACHINES Tensile testing machines vary both in design and capacity. Large machines capable of applying forces up to 1 MN are in use. The small Hounsfield tensiometer has a capacity of 20 kN. EXTENSOMETERS Extensometers are used for measuring the extension over the gauge length of a test piece during a tensile test. Strains within the elastic limit are extremely small, and during the routine testing of materials it is necessary to take measurements as small as 0.0025mm (0.0001 in). HARDNESS TESTS Hardness is the resistance of a material to indentation, and by pressing a suitably shaped indenter into the surface of the material being tested; a comparison of hardness values can be made. Hardness values are normally required by the material specification, and tests are also carried out to check case hardening and hardening and tempering at the various stages of manufacture. 1. THE BRINELL TEST In this test a hardened steel ball is forced into the surface of a test piece by means of a suitable standard load. The diameter of the impression is then measured using a calibrated microscope and the Brinell Hardness Number (H) is found from: H = Load (P) Area of curved surface of the impression Knowing D the diameter of the ball and d the diameter of the impression, as in above, the hardness number can be calculated. To make calculations unnecessary, the hardness number H is found by reference to British Standard tables, where H is read directly by entering the diameter of the impression d. The greater the number, the harder the material. 2. THE VICKERS PYRAMID HARDNESS TEST The Vickers hardness test is similar to the Brinell in that an impression is made by applying a load to an indentor. The hardness number is then obtained from measurement of the impression. The indentor is a diamond in the shape of a square based pyramid and the measurement is made across the diagonal of the impression. An advantage of the Vickers hardness test is that hardness values for very hard materials are more accurate than the corresponding Brinell numbers. This is because a diamond does not deform under high pressure, as does a steel ball, and so the result will be more accurate. MEASUREMENT The diagonal length of the square impression is measured by means of a microscope which has a variable slit built into the eyepiece. See Fig. below. The width of the slip is adjusted so that its edges line up with the corners of the impression. The diagonal length of the impression is then obtained from a digital counter geared to the movement of the slip. The reading is converted to Vickers Pyramid Hardness Number (VPN) by reference to tables. The higher the number the harder the material. The specified time of contact between the indentor and the test piece in both Vickers and Brinell hardness tests is 15 seconds. 3. THE ROCKWELL TEST The Rockwell Test was developed in the U.S.A. It is used mainly for the rapid routine testing of finished materials, the hardness number being indicated directly on a dial, no measurement of the diameter of the impression is required. THE ROCKWELL HARDNESS TESTER 4. THE SHORE SCHEROSCOPE TEST IMPACT TEST Impact tests are used to indicate the toughness of a material and most importantly its ability to resist mechanical shock, to ensure that temper brittleness has not been introduced during heat treatments. There are two types of machine used for testing aircraft materials, both of which use a pendulum weight to fracture the specimen. The energy absorbed by the specimen is measured from the angle through which the pendulum swings after causing the fracture. The IZOD test is required by most of the British material specifications, but where the test piece must be tested at high or low temperatures the CHARPY test is used. This completes the test within the required 6 seconds after removal of the test piece from the heating or cooling bath. Machines are available which carry out both the IZOD and Charpy tests and FIG. 8 shows such a machine. Impact testing machine FATIGUE TESTING Fatigue Testing Machine CYCLIC STRESSING What do we mean by cyclic stressing? Cycles are a series of changes which restore a system to its original state. This is the flexing of a material until it breaks. If you were given a strip of metal or plastic and asked to break it in half, and the material was so far too strong to break in half simply by pulling it apart, you would probably flex the material from side to side until it weakened and eventually failed. This is called **cyclic stressing** and the failure mode is **fatigue failure**. Below are all the ways in which aircraft structures and components are cyclically stressed: - Pressurization - Turbulence -- vibration caused by varying flight loads, especially through bad weather. - Change in temperature -- especially in the engine. This is known as thermal expansion. - Landing and takeoff -- especially the wings and landing gear. - Flight control rods and cables -- tension and compression. 3. **Practical Test (Ferrous Metals)**