Chapter 4: Enzymes 2024/2025 PDF
Document Details
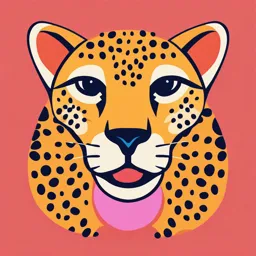
Uploaded by ConciliatoryChimera
Universiti Teknologi MARA
Tags
Summary
This document comprises lecture notes for a Biochemistry course, specifically Chapter 4 on Enzymes. It discusses topics such as catalysis, activation energy, enzyme mechanism, different types of enzyme inhibitors and enzyme kinetics, including the Lineweaver-Burk plot.
Full Transcript
CHAPTER 4: ENZYMES BIO091 Semester 1 2024 / 2025 1 SUBTOPICS 4.1 Catalysis and concept of activation energy 4.2 Mechanism of enzyme action 4.3 Factors affecting the rate of enzyme reactions 4.4 Enzyme kinetics 4.5 Cofactor...
CHAPTER 4: ENZYMES BIO091 Semester 1 2024 / 2025 1 SUBTOPICS 4.1 Catalysis and concept of activation energy 4.2 Mechanism of enzyme action 4.3 Factors affecting the rate of enzyme reactions 4.4 Enzyme kinetics 4.5 Cofactors 4.6 Enzyme inhibition 4.7 Regulation of enzyme activity 4.8 Classification of enzymes 4.9 Enzyme technology LEARNING OUTCOMES 4.1 Explain how an enzyme lowers activation energy of a reaction. 4.2 Explain the hypotheses related to the mechanism of enzyme action. 4.2 Explain the formation of the enzyme-substrate complex. 4.3 Explain factors that affect the enzymatic reaction. 4.4 Explain the models of enzyme kinetics. 4.4 Explain the double reciprocal plot of enzyme kinetics. LEARNING OUTCOMES 4.5 List the types of cofactors. 4.6 Discuss the mechanism of enzyme inhibition. 4.7 Explain the allosteric site in an enzyme. 4.7 Discuss how enzyme inhibitors and activators regulate enzyme activity in a cell. 4.8 List the classes of enzymes and explain their role. 4.9 Discuss the importance and the main techniques of immobilized enzymes. 4.1 CATALYSIS AND CONCEPT OF ACTIVATION ENERGY Enzyme: ⮚ An organic catalyst (usually a protein) that accelerates a specific chemical reaction by lowering the activation energy required for that reaction. - Solomon 11th edition Characteristics of enzymes Most are globular proteins. Act as catalysts Not permanently altered/ changed Not consumed by the reaction Can be reused Have 1 or more active sites where substrates bind to Lowers the activation energy (EA) needed to initiate a reaction 4.1 Catalysis and concept of activation energy Activation energy (EA) Also known as energy of activation. Activation energy (EA) is the initial energy needed to start a chemical reaction. Source of EA : Heat energy from surroundings. 4.1 Catalysis and concept of activation energy Enzyme lowers the activation energy (EA) by 1. Bringing the substrates together in the correct orientation. 2. Providing a favorable cellular microenvironment. 3. Stretching the bonds of substrate toward their transition state. 4. Forming temporary covalent bond with substrate. Enzyme lowers activation energy Fig. 8-15 Transition state Course of reaction EA without without enzyme EA with enzyme enzyme is lower 1. Reactants orange- Reactant 2. After bonds are broken, purple and green-blue must absorb enough s new bonds form, releasing energy from the surroundings to reach Course of ∆G is energy to the surroundings the unstable transition state, reaction unaffected where bonds can break. with by enzyme 3. An enzyme cannot enzyme change ΔG. Instead, it speeds up a reaction that would eventually Products occur. ΔG = the change in free Progress of the energy reaction 4.2 MECHANISM OF ENZYME ACTION LOCK and KEY MODEL The substrate is complementary to the active site of the enzyme. Substrate binds to the active site of the enzyme. Forms enzyme-substrate complex. product Breakage of old bonds and formation of new ones Substrates changed into product Product has different shape from the substrate. Product is released from enzyme. This mechanism is now considered as incorrect. Mechanism of enzyme action: INDUCED-FIT MODEL The substrate is not complementary to the active site of an enzyme. Binding of substrate induces a slight conformational change to the active site of the enzyme. The shape of the substrate also changes slightly. Catalytic groups on the enzyme are in the correct orientation with the substrate. Breakage of old bonds and formation of new ones. Substrate changed into product. The product has a different shape from the substrate. Product is released from enzyme Enzyme returns to its original shape Catalytic cycle of an enzyme 4.3 FACTORS AFFECTING THE RATE OF ENZYME REACTIONS I. Enzyme concentration II. Temperature III. pH IV. Substrate concentration 12 I. Enzyme concentration Unlimited substrate If substrate is not the limiting Rate of reaction factor, the rate of reaction is Substrate limited proportional to enzyme concentration. If substrate is the limiting factor, adding enzyme does not change the rate of reaction. II. Temperature The rate of reaction increases with increasing temperature. ○ Molecules move rapidly. ○ More collisions between substrates and active sites. ○ The rate of reaction doubles for every 10°C rise ii. Optimum temperature: in temperature. ⮚ enzymes are the most active. ⮚ Rate of reaction is the fastest ⮚ balance between enzyme stability and kinetic energy. i. At low temperature: ⮚ enzyme activity is reduced iii. Above optimum temperature: or does not occur at all, ⮚ High kinetic energy disrupts 3-D structure of active ⮚ because of a lack of sites. energy for the reaction to ⮚ Hydrogen bonds are broken. take place. ⮚ Enzyme becomes denatured. Temperature coefficient (Q10) is the factor by which the rate of reaction increases with every 10oC rise in temperature. t = temperature (oC) If Q10 is 2, the rate of reaction doubles with every 10oC rise in temperature. III. pH Optimum pH is the surrounding pH where an enzyme is most active. Optimal pH for pepsin Optimal pH for trypsin (stomach enzyme) (intestinal Changes in pH [H+ or OH-], Rate of reaction enzyme) ✓ change the ionic charges on the active site of enzymes. ✓ break weak bonds (hydrogen 0 2 4 5 6 7 8 9 10 bonds / ionic bonds). 1 3 pH ✓ alters 3-D shape of active site. Optimal pH for two enzymes ✓ substrate cannot fit into the active Stomach acidic environment.. Alkaline environment in mostly denatures other enzymes. the human intestine. site. ✓ less products produced. ✓ enzyme is denatured. IV. Substrate concentration (a) The rate of reaction increases with increasing substrate concentration. ⮚ due to more collision between enzyme and substrate (b) Rate of reaction increases but at a lower rate because fewer active sites are available. (c) Maximum rate of reaction (Vmax) because all active sites are saturated with substrates. 4.4 ENZYME KINETICS ⮚ is the study of enzyme reaction rate. In an enzyme-catalyzed reaction, a hyperbolic curve is obtained. Michaelis-Menten kinetics explains: how enzyme enhances the rate of reaction. how the rate of reaction depends on the concentration of enzyme and substrate. The Michaelis-Menten kinetics model is also known as the saturation plot. Michaelis-Menten Kinetics Rate of reaction (moles/time) 1. At low substrate concentrations, ○ the rate of reaction rises almost linearly with the increasing substrate concentration. 2. As the substrate concentration increases, ○ the rate of reaction reaches the maximum rate, Vmax ○ This is because the enzyme concentration becomes the limiting factor. ○ The addition of more substrates does not increase the reaction rate because all the active sites of the Substrate concentration (molar), [S] enzyme are saturated with substrate molecules Michaelis-Menten constant, Km Km = the substrate concentration required at which the rate of reaction (the reaction velocity) is half the maximum rate (½ Vmax). The curve can be used to estimate Vmax and Km. Rate of reaction (moles/time) Vmax = maximum rate of reaction Reveals the maximum number of substrate molecules converted into products per second. Km = substrate concentration at ½ Vmax. (or The concentration of substrate required to Substrate concentration (molar), [S] half saturate the enzyme ) Significance of Km Km value ○ varies from one enzyme to another. ○ measures of the affinity of an enzyme for its Rate of reaction (moles/time) substrate. ○ The smaller Km value, ✓ lower substrate concentration needed to reach ½ Vmax ✓ the greater the affinity of binding between the enzyme and its substrate (the more strongly the enzyme binds the substrate). ✓ indicates the more efficient the enzyme is at converting its substrate into products ✓ The more likely for the product to be formed Substrate concentration (molar), [S] Significance of Km Km value ○ can be used to compare the efficiency of different enzymes or the same enzyme from different sources. ○ depends on the identity of substrate, temperature, pH, and presence of inhibitors ○ Example: If an enzyme can catalyse a reaction with two similar substrates (e.g., glucose and fructose), it would prefer the substrate with the lower Km value. ○ High Km 🡪 The enzyme and substrate breaking apart more than staying together Lineweaver-Burk Plot Is a representation of the Michaelis-Menten kinetics. It plots the inverse of the reaction rate (1/V) against the inverse of the substrate concentration (1/[S]) Produces a straight line on a double-reciprocal graph Values (such as Vmax and Km) from a straight-line plot can be determined more accurately compared to the hyperbolic curve. ○ This is because plotting points to produce a hyperbolic curve requires a considerable number of data. ○ It is also more difficult to determine Vmax because of the gradual upward slope of the hyperbola. Use fewer data. Lineweaver-Burk Plot Transform the hyperbolic plot (Michealis-Menten kinetics) into a linear plot. 1 It is a double-reciprocal graph: V 1/V against 1/[S]. This provides a more precise way to determine Vmax and Km. -1 The x intercept = Km x The y intercept = 1 Vmax Km The slope = Vmax Exercise [S] velocity Draw graph velocity (V) vs [S] 0.02 0.025 0.04 0.041 0.06 0.052 Draw the Lineweaver-Burk plot 0.08 0.061 0.10 0.067 Calculate the values for Vmax and 0.20 0.085 KM from the Lineweaver-Burk plot. 4.5 COFACTORS Cofactor is a nonprotein molecule or ion needed for proper functioning of enzyme. Some enzymes consist of only protein. Some enzymes can only function when another chemical component is present. 4.5 Cofactors Apoenzyme is an inactive enzyme require a cofactor (additional chemical component) to be functional Holoenzyme is a complete and active enzyme. 4.5 Cofactors Cofactor modify the chemical structure of enzyme in certain ways that enables it to function more effectively. Can be an organic or inorganic molecule. Three (3) types of cofactors: 1. Coenzyme 2. Metal ions 3. Prosthetic groups 4.5 Cofactors : i) Coenzymes Coenzymes are small, organic and non-protein molecules. Binds loosely and temporarily to active site of enzymes. Most coenzymes are carrier molecules that transfers chemical group, atoms or electrons from one molecule to another. e.g.: NAD+, NADP+, Coenzyme A (CoA), and FAD. NAD+ is a coenzyme for several dehydrogenase enzymes & acts as a hydrogen acceptor. 4.5 Cofactors : ii) Metal Ions / Activators Metal ions or activators are inorganic cofactors. Bind temporarily to the enzyme and change the shape of the active site. Allowing chemical reactions to take place. Remove electrons from substrate molecules, making bonds less stable and easier to break. Required in very small amounts. Examples: Mg2+, Ca2+, Fe2+, Zn2+ e.g.: Ca2+ is needed to activate thrombokinase which converts prothrombin to thrombin 4.5 Cofactors : iii) Prosthetic Groups Prosthetic groups are organic molecules that bind permanently to enzyme. Example: i) Heme, an oxygen-binding protein Consists of a porphyrin ring and a central iron (Fe) atom. A prosthetic group in oxidoreductases. Acts as a hydrogen or electron donor and reduces a hydrogen or electron acceptor. 4.5 Cofactors : iii) Prosthetic Group Example: ii) Biotin i. Biotin carries a carboxylic group. Is a prosthetic group in carboxylases. Transfers CO2 to various target macromolecules. Necessary for metabolism and growth in human. Biotin 4.6 ENZYME INHIBITION Inhibitors are certain chemicals that selectively inhibit the action of specific enzymes. Reversible inhibitors Irreversible inhibitors Bind reversibly to enzymes Bind irreversibly to enzymes form weak/hydrogen bonds (with form strong/covalent bonds with enzymes) enzymes enzymes can revert to functional Enzymes unable to function structure Binds loosely to enzyme/bonds Binds tightly and permanently to break easily enzyme A) Reversible Inhibitors Reversible inhibitors i) competitive or ii) non-competitive ○ forms weak bonds (easily broken) such as hydrogen bond with the enzyme. ○ the inhibition only takes place whilst the inhibitor is attached to the enzyme. bind to the active site bind to the allosteric site Reversible inhibitors: I) Competitive inhibitors Similar shape to the natural substrate (close structural similarity). Compete with the substrate for the same active site. Slows down the rate of reaction. Less products produced. Can be overcome by increasing substrate concentration. Competitive Inhibitors Example: Enzyme - succinate dehydrogenase Substrate - succinate Product - fumarate Competitive inhibitor - malonate. Reversible inhibitors: II) Non-competitive inhibitors No structural similarities with substrate Bind to allosteric site Binding causes enzyme to change its conformation Substrate cannot bind to the active site Enzyme not functioning temporarily Slower rate of reaction Less products produced Competitive vs non-competitive inhibitors increasing the substrate concentration to overcome inhibition increasing the substrate concentrations doesn’t affect the rate of reaction B) Irreversible inhibitors ▪ Irreversible inhibitors / Non-reversible ⮚ bind tightly and permanently to active sites of enzymes ⮚ with strong covalent bonds ⮚ Inactivates or destroys enzyme ⮚ This can occur at low inhibitor concentrations. ⮚ Examples include: ⮚ heavy metals such as lead, mercury and silver. ⮚ poisons like sarin and cyanide. ⮚ antibiotics like penicillin. Irreversible inhibitors Many antibiotics are inhibitors of specific enzymes in bacteria. Example: Penicillin – blocks the active site of enzymes that many bacteria use to make cell walls. ⮚ The growth of bacterial cell wall slows. ⮚ Bacteria cannot survive. ⮚ Infection stops. 40 Irreversible inhibitors Toxins and poisons. Example: Sarin (a nerve gas) no odor, no taste, target the nervous system. binds covalently to the R group of amino group sarine, found in the active site of acetylcholinesterase. alters the shape of the active site. acetylcholine (a neurotransmitter) cannot bind to the active site and cannot be broken down. Therefore: ✔ acetylcholine molecules accumulate in the synaptic cleft. ✔ causes continuous transmission of nerve impulses. ✔ causes continuous contraction of muscles ✔ leads to convulsions, paralysis and eventually death Irreversible inhibitors Example: Cyanide Most commonly, cyanide toxicity is the result of smoke inhalation during domestic fires. Cyanide inhibits cytochrome c oxidase, the last enzyme in the electron transport chain (in cellular respiration). cytochrome c oxidase can no longer transfer electrons from its substrate to oxygen. leading to the cessation of oxidative phosphorylation (the production of ATP via the use of oxygen) Cyanide poisoning causes death. 42 4.7 REGULATION OF ENZYME ACTIVITY Regulation of enzyme activity is important to coordinate numerous metabolic processes. Cells can regulate its metabolic pathway by controlling when and where its various enzymes are active (by switching on and off the genes that encode specific enzymes). If all metabolic pathways operate simultaneously, there would be chemical chaos! How does regulation of enzyme activity help control metabolism? 1. Inactive enzyme – e.g. pepsinogen 2. Presence of inhibitor – i.e. reversible inhibition 3. Regulator molecule may activate or inhibit enzyme – i.e. allosteric regulator 4. End product may inhibit enzyme – i.e. feedback inhibition flux 1) Allosteric regulation Regulatory molecules bind briefly and reversibly to an allosteric site (a site other than the active site). Changes shape (conformational change) of the active site. Alters the activity/ function of the enzyme Allosteric activation Allosteric inhibition (a form of noncompetitive inhibition) Allosteric regulator (allosteric Allosteric regulator (allosteric activator) binds to enzyme inhibitor) binds to enzyme Enzyme is in its active form Enzyme is in its inactive form Regulatory site = allosteric site Alternation A shape change in 1 subunit, is transmitted to all others 46 2) Cooperativity A type of allosteric activation. The binding of a substrate molecule to the active site of one subunit, locks all subunits in its active conformation, causes the second and subsequent substrates to bind more readily. This amplifies the activity of enzymes for its substrates. 3) Feedback Inhibition Activity of an enzyme near the beginning of a metabolic pathway is inhibited by the end product. is an allosteric inhibition. The increasing concentration of end product serves as a signal for the enzyme to slow down and eventually stop functioning Importance: prevents the cell from wasting chemical resources Feedback inhibition Isoleucine synthesis Synthesis of isoleucine from threonine involves 5-steps Each step is catalyzed by different enzymes Threonine deaminase (Enzyme 1) catalyzes the first step When enough isoleucine accumulates in the cell, it binds to the allosteric site of threonine deaminase Inhibits activity of threonine deaminase Importance - prevents the cell from synthesizing more isoleucine than is necessary. 4.8 CLASSIFICATION OF ENZYMES Named - by adding the suffix ‘ase’ at the end of the name of the reaction it catalyses. SIX classes - based on the type of reaction the enzyme catalyzes 1. Oxidoreductase 2. Transferase 3. Hydrolase 4. Lyase 5. Isomerase 6. Ligase This classification is based on recommendations of the International Union of Biochemistry and Molecular Biology Classes of enzymes Class Chemical reaction catalyzed Example Oxidoreductase Catalyse oxidation-reduction NADH dehydrogenase reaction. Catalyse transfer of hydrogen or oxygen atoms, or electrons from one compound to another Transferase Catalyse the transfer of a Hexokinase functional group from one substrate to another Classes of enzymes Class Chemical reaction catalyzed Example Hydrolase Catalyse hydrolysis reaction Dipeptidase (addition of water) Lyase Catalyse formation or removal Fumarase, of a double bond with group PEP carboxylase transfer Classes of enzymes Class Chemical reaction catalyzed Example Isomerase Catalyse rearrangement of Triose phosphate isomerase atoms within a molecule (conversion of one isomer into another) Ligase Catalyse bond formation DNA ligase between two compounds (joins molecules) using energy derived from the breakdown of ATP 4.9 Enzyme technology Enzymes extracted from cells are more useful when not in solution. Important for industrial processes that manufacture products in bulk. ○ Use of elevated temperature to increase product yield. ○ Cost is minimized if the enzyme is not lost with the products. Immobilised enzyme: Enzymes attached to or trapped within insoluble material. Materials developed for binding enzymes include alginate, cellulose, porous glass, agar gel, nylon, and porous lamina. 4.9 Enzyme technology Advantages: ○ Enzymes can withstand high temperature ○ Enzymes more resistant to pH changes ○ Enzymes do not contaminate products ○ Enzymes can be re-used multiple times Disadvantages: ○ Possible (partial) loss of enzyme activity ○ Changes kinetics of the enzyme Methods of immobilizing enzyme i) Adsorption The first method of immobilization developed Enzyme is physically bonded onto the surface of insoluble support via weak bonds. ○ Hydrogen bond ○ Ionic interaction ○ Hydrophobic interaction ○ Van der Waals forces Examples of support: silica gel, porous glass Advantage: Relatively simple and cheap Disadvantage: Enzyme can easily be washed from the adsorptive material Methods of immobilizing enzyme ii) Entrapment Enzyme is retained/ trapped within 3-dimensional structure of matrix (porous gel matrix or polymer). Enzyme not chemically bound. Substrate and products can pass through the pore of the matrix, but the enzyme is retained. Example of matrix: alginate, silica gel, agar, gelatin Methods of immobilizing enzyme iii) Encapsulation Enzymes trapped within a semi-permeable membrane A type of entrapment. Examples of membrane: nylon, cellulose nitrate Methods of immobilizing enzyme iv) Covalent bonding Covalent bonding between enzyme and solid support. Example of support: cellulose, collagen 59 Methods of immobilizing enzyme v) Cross linking Enzyme molecules are covalently bonded to each other by cross-linking reagents. Example of reagent: glutaraldehyde Disadvantage: Enzymes can be denatured by the cross-linking reagent. Commercial uses of enzymes Application Enzyme Use Production of fruit juice Pectinase Breakdown of pectin in plant cell walls Breakdown of proteins in flour for biscuit Baking industry Protease manufacture Dairy industry Lactase Production of lactose-free milk Detergent industry α-amylase Removal of stain on fabrics Glucose- Food industry Manufacture of fructose syrup from glucose isomerase Paper industry Ligninase Removal of lignin from pulverized wood Medical Trypsin Removal of blood clots, and in wound cleaning Pharmaceutical Glucose oxidase Detection of glucose levels in blood Generation of Lactose-free Milk Using Immobilized Enzyme For people with lactose intolerance, drinking milk or eating dairy products can result in nausea, bloating, abdominal cramps. Lactase can be used to produce lactose- reduced milk. Lactase can be immobilised within alginate beads. Milk (substrate) is poured through a column containing immobilised lactase. Lactase hydrolyses lactose into ______ and _______. Lactose-free milk (product) contains simple sugars that can be absorbed directly into 63 the bloodstream. End of Chapter 4