Sample Preparation Guide PDF
Document Details
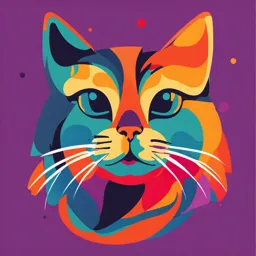
Uploaded by ReceptiveIdiom
Tags
Summary
This document explains different methods of sampling and preparation for various types of samples and provides information on sample stability and preservation. It also details techniques like cone and quartering and discusses how to avoid sample contamination during the procedure.
Full Transcript
Sample Collection and Preparation 1 Collecting Samples – Liquid or Gas Liquids and gases are very often homogeneous. Because of this, the gross samples collected do not need to be very large. If you are collecting a sample from a large container such as a ste...
Sample Collection and Preparation 1 Collecting Samples – Liquid or Gas Liquids and gases are very often homogeneous. Because of this, the gross samples collected do not need to be very large. If you are collecting a sample from a large container such as a steel drum, it may not be homogeneous. 2 Collecting Samples – Liquid or Gas In such a situation, you should take a few sampling units or sampling increments from different locations inside the drum. If you are collecting water samples from a lake or pond, to determine dissolved gases, the concentrations may vary significantly at different depths. You may want to collect samples at different depths. 3 Collecting Samples - Solids Solids are very often heterogeneous. To get a representative sample of soil, you usually need to take a larger gross sample than in the case of liquids. You may take sample increments at several locations and mix them together (composite them) to get a more representative gross sample. If this is done, you may need to reduce the sample mass before doing the analysis. 4 Soil Preparation There are several ways to accomplish this. A common homogenization and sample volume reduction method is the cone and quarter technique. This involves pouring the bulk sample into a cone, flattening the cone, dividing the flattened cone into 4 equal divisions and then removing two opposing quarters. The two remaining quarters are then recombined to form a new pile and the process is repeated until the desired sample size is obtained. 5 Cone and Quarter 6 Cone and Quarter Potential sources of error in using this protocol arise from unequal segregation of heavier materials during the flattening and coning process. Also, loss of fine material due to dust production or the ability of the sample to adhere to the work surface due to static charge are additional sources of error. Cone and quartering is typically used for sample masses greater than 50 g. 7 Cone and Quarter A variation on this method which does not include sample reduction provides homogeneity by dividing a cone of material into 4 approximately equal quarters. These are numbered clockwise from 1 through 4. The first quarter is removed and set aside; the third, second, and fourth quarters are piled sequentially on the first quarter. This process is repeated 6 times to ensure homogeneity. This would be subject to the same errors as noted above. 8 Sample Stability The perishable foods that we have at home in our kitchens (fruits, vegetables, meat, dairy, etc.) will begin to decompose or “go bad” after a period of time. Similarly, the chemical species (elements, ions, compounds) that exist in a sample will also begin to decompose after some time. The most common changes in a sample between sampling and analysis are a result of volatilization, adsorption, diffusion, precipitation, oxidation, photochemical changes, and microbiological degradation. 9 Ways that samples can change Volatilization refers to the physical process in which volatile species are lost to the atmosphere. This is eliminated by containing the sample in a bottle with no air or headspace. Metals can be irreversibly adsorbed onto glass surfaces. Oils are irreversibly adsorbed onto walls of plastic containers. Organic molecules such as plasticizers diffuse or migrate out through plastic walls or bottle caps. 10 Ways that samples can change Light can often degrade certain organic components such as polycyclic aromatic hydrocarbons (PAHs). Samples may contain organisms that may degrade organic components. For example, there are bacteria that decompose phenolic compounds. 11 Holding Time Because samples can change in many ways, there is a limit on the amount of time allowed between when a sample is collected and when it is analyzed. The maximum time that is allowed from sample collection until sample analysis begins is called the holding time. 12 Holding Time Different chemical species have different stabilities in samples. Some may not change significantly even after 3 or 4 weeks. Others may change a lot (decompose) in just a few days. Therefore the holding times are different for different analytes. 13 Typical Holding Times Electrical Conductivity 4 days Cyanide 7 days Formaldehyde 48 hours Residual chlorine immediately Methanol 7 days Nitrate 5 days Metals 60 days 14 Sample Preservation Preservation techniques often involve the addition of a chemical which ‘ties up’ the analyte in a form which is less affected by sample aging or else provides conditions unsuitable for further reaction to occur. In some cases, refrigeration or freezing to reduce reaction rates provides the best preservation, especially for those parameters which have a direct biological relationship (with respect to growth or decline). 15 Sample Containers Just as important as preservatives in minimizing analyte change is the sample or shipping container. Selection of sample container is also determined by the volume required for analysis. If containers are prepared by the laboratory, the sampler must ensure that the containers are clean and have been properly prepared. This may include pre-labeling, pre-charging with preservative, and capping tightly. 16 Sample Containers If the lab is not preparing sample bottles, the sampler must ensure that it is done. Sample containers, taken at random, should be analyzed to ensure that there is no contamination present from the container or preparation procedure (container proofing). 17 Sample Containers Some general guidelines to selection of containers: – Conventional parameters in water matrices (i.e., pH, hardness, nutrients) can be collected in 500 mL plastic containers. Avoid plastics which ‘breathe’ since parameters such as ammonia may change significantly in transit. Sludge samples should never be filled more than half way to allow for gas formation. Trace metals and cyanide are collected in the same type of plastic container. 18 Sample Containers Solid samples for any of the conventional or trace metal parameters may be collected in either wide mouth plastic or glass jars or even plastic bags. Some solid samples such as vegetation should be dried as quickly as possible to prevent decay. 19 Sample Containers Organic parameters are collected in glass containers regardless of the matrix. The lids of these containers should be lined with teflon or aluminum foil. Do not use plastic bottles for organic parameters since low level contamination may occur. As a worst case, plastic bottles containing organic solvents have actually dissolved before getting to the lab for analysis. Samples for volatile parameters must be collected and stored with no headspace (air) in the containers. 20 Grab Samples / Composite Samples There are 2 general types of samples: grab and composite. The selection of sample type is governed by the information sought, the purposes of the sampling, the analyses to be performed, and the flow pattern. A grab sample is defined as an individual discreet sample collected at one place and at one specific time. 21 Composite Samples A composite sample is defined as a sample formed by mixing discreet (grab) samples collected over periodic points in time or a continuous proportion of the flow. The number of grab samples depends on the variability of the concentration and flow. A composite sample represents average conditions for the given duration, usually 24 hours. This type of sample requires a greater amount of time and is often collected with an automated sampler. 22 Sample Preparation Many kinds of analysis require certain steps to be taken with samples before or besides the measurement step. These are collectively referred to as Sample Preparation. 23 Sample Preparation Sample preparation (sample pretreatment) is often the most time-consuming, most error-prone, and most labour-intensive part of an analysis. It requires a lot of expertise in chemistry. No matter how simple or complex the procedures appear, the same 5 general principles apply to all sample preparations. 24 Principles of Sample Preparation 1. The sample preparation should be done without losing any of the analytes. Material should not be lost in the preparation, but if it is, some way must be found to discover how much is being lost. The quantitative measure for the amount of the analyte that remains for the determination from the original sample is called the recovery of the procedure. 25 Principles of Sample Preparation 2. The sample preparation should include bringing the analyte(s) into the best chemical form for the analysis. Some analytical methods need solids, some need liquids, and some need gaseous forms. If the sample must be transformed, sometimes this must be done by the analyst (such as dissolving a salt). Sometimes the change is done by the instrument, such as when a flame is used to break up a sample matrix. It is not uncommon for the transformation step to be a major contribution to analytical error. Also, the specific chemical form may be crucial. 26 Principles of Sample Preparation 3. The sample preparation should, if necessary, include removing interferents in the matrix. Almost all analytical methods respond to a greater or lesser degree to interferents – species of atoms, ions, or molecules other than the desired analyte. A method that is less prone to interference is said to have greater specificity. If the specificity of a method is improved, the sample preparation steps can often be significantly simplified. 27 Principles of Sample Preparation 4. The sample preparation should be done without adding any new interferents. Conditions should prevent adding any new interferents from the reagents or reaction vessels. The most common problem is cross contamination, which happens when some material from one sample becomes included in another sample. Cross contamination is harder to eliminate when the levels of an analyte vary greatly between samples. The more the concentrations differ, the greater the effect. It can occur in any stage of the analysis.28 Principles of Sample Preparation 5. The sample preparation should include, if necessary, diluting or concentrating the analytes to bring their concentrations into the best range for the method used. Every method has an optimum concentration range. Few methods have the same precision over more than 3 orders of magnitude in concentration – where the high end is 1000 times the concentration of the low end. 29 Sample Preparation These 5 requirements often involve trade- offs. For example, a treatment that gives better recovery may also add interferents. There are two other issues that influence sample preparation: – Speed of analysis – Environmental impact and cost of chemicals Because of these factors, as well as the cost of sample preparation, a lot of research is being done to develop better methods for sample preparation. 30 Speed of Analysis Instruments are becoming faster all the time. If in one minute, the measurement is carried out, data posted to the LIMS, the next sample loaded from an autosampler, then, in order to fully utilize the instrument, someone has to prepare 60 samples per hour. Not long ago, when only wet chemistry methods were available, and all determinations were done by hand – such as manual titrations – an efficient person might do 10 to 15 analyses in an hour. Sample throughput has increased by 1 to 2 orders of magnitude since those days. 31 The use of chemicals There has been a rapid increase in costs associated with purchase and disposal of solvents and chemicals in general and a desire for less waste. The response to this has been to use smaller amounts of both organic solvents and acids or to find substitutes for the entire process. 32 The use of chemicals For example, it has been common practice to extract organic compounds from water samples by shaking the water in a separatory funnel with an organic solvent such as ether and then using the ether phase for further processing. Such ether extractions are commonly replaced by passing the water sample through a tube filled with a solid with a nonpolar surface. The organics adsorb on the nonpolar surface – they are stripped out of the sample. A small amount of organic solvent such as methanol can then be used to recover the adsorbed analyte, or the solid can be heated to desorb the organic compounds. 33 Sample Preparation - Solids Many types of solid samples may need crushing or grinding to reduce the particle size. This is needed to help make the sample homogeneous and to make it easier for reagents to react with it. Samples should not be ground any more than necessary because it can change the composition. The heat generated in grinding can cause loss of volatile components. 34 Crushing and Grinding Grinding will also increase the surface area of a solid. If the solid can react with the atmosphere, this would accelerate after grinding. The water content of a sample will often increase with grinding due to the larger surface area and the increased possibility of adsorption. For hydrated compounds, the water content may decrease with grinding due to frictional heating. 35 Crushing and Grinding After grinding, samples are usually screened (sieved) to ensure a consistent particle size. Grind → remove ground (softer) particles → grind some more → remove ground (harder) particles → repeat 36 Crushing and Grinding Occasionally, the sample may be contaminated by the abrasion of the grinding surfaces of the grinding apparatus. This would be particularly a problem when determining the concentration of trace components of the sample. 37 Tools to reduce particle size For large samples, a jaw crusher can be used. See the animation about 2/3 of the way down the page at the link. A Disk pulverizer can also be used. For medium size samples, a ball mill can be used. It consists of a container that can be sealed and rotated mechanically. The sample and an equal volume of flint or porcelain balls (2 to 5 cm diameter). For small samples, mortar and pestle. 38 Moisture in Solid Samples Many types of solid samples contain water that is in equilibrium with the atmosphere. The water content of the sample is very variable. If it is a soil sample, e.g., it would contain much more water if it was collected following rain. Also, the sample may lose an unpredictable amount of moisture between collection and analysis. The results of all analyses on the sample are affected by the water in the sample. 39 Moisture in Solid Samples For this reason, it is standard practice in analytical labs to report analytical results on a “dry weight” basis – as if the sample contained no water at all. To correct all the analytical results for water content, a separate portion of the sample is weighed before and after drying to determine its moisture content. A correction factor for the moisture content is then applied to all the other analytical results. 40 Forms of Water in Solids: Essential Water Essential water is an integral part of the molecular or crystalline structure of a solid compound. Two types: – Water of crystallization in a hydrate, e.g., CoCl2·6H2O – Water of constitution is water that is formed when a pure solid is decomposed by heat or other chemical treatment, e.g., calcium hydroxide when heated Ca(OH)2(s) ⇄ CaO(s) + H2O(g) Essential water is in stoichiometric proportion. 41 Forms of Water in Solids: Nonessential Water Nonessential water is the water that is physically held by a solid. It does not occur in stoichiometric proportion. Three types: – Adsorbed water is water held on the surface of solids. Its amount depends on temperature, humidity, and surface area. – Sorbed water is seen in colloids, such as starch, protein, silica gel. It is held in the interstices of the colloidal solid. Its amount also depends greatly on temperature and humidity. – Occluded water is water trapped in irregular microscopic pockets in solid crystals. 42 Effect of Temperature and Humidity on Water Content The concentration of water in a solid generally decreases with increasing temperature and decreasing humidity. How this happens depends on what kind of water is in the solid. Essential water: Some compounds can form multiple hydrates, e.g., BaCl2. Which one of the hydrates exists at any time may depend on the relative humidity. 43 Effect of Humidity on Water Content This diagram shows how the amount of adsorbed water on a solid increases as the humidity increases. The amount of water adsorbed on a solid decreases as the temperature increases and approaches zero when the solid is heated above 100°C. 44 Effect of Humidity on Water Content The diagram also shows the effect of humidity on sorbed water. The plot shows that much more water can be sorbed than adsorbed. Solids might adsorb a few tenths of a percent of water. Colloids can sorb up to about 20% of their weight in water. Adsorption is rapid. It may reach equilibrium in a few minutes. Sorption is much slower and may take days or weeks to equilibrate. 45 Effect of Temperature on Sorbed Water This plot shows the effect of drying temperature on the removal of water that is sorbed on a colloid. Note that at 105°C, a constant weight is achieved, but not all the moisture has been removed. 46 Occluded Water Occluded water is water trapped inside some crystals. Since there is no way for the water to get out, it is not in equilibrium with the atmosphere. Therefore, the amount of occluded water does not depend on humidity. Sometimes, a crystal that has occluded water may undergo decrepitation when it is heated, i.e., it may explode due to the buildup of steam pressure. 47 Dissolving and Decomposing For most kinds of inorganic analysis, the sample must be in aqueous solution for the measurement to be done. Some samples may be in aqueous solution to start with, but many (including solid samples) are not. Samples may need to be treated with reagents in order to get them into solution. 48 Errors in Decomposing and Dissolving The steps involved in decomposing and dissolving samples involve potential errors 1. Incomplete dissolution. It is possible that not all of the sample gets dissolved, or that not all of the analyte goes into solution. 2. Loss of analyte by volatilization. Some part of the analyte may volatilize. CO2, SO2, H2S, H2Se, H2Te are often lost when samples are dissolved in strong acid. NH3 may volatilize when base is used. Some elements form volatile chlorides that may be lost from hot hydrochloric acid solutions (e.g., chlorides of Sn(IV), Ge(IV), Sb(III), As(III), and Hg(II). Boric acid, nitric acid, and the halogen acids can be lost from boiling aqueous solutions. 49 Errors in Decomposing and Dissolving 3. Introduction of the analyte as a solvent contaminant. Usually, the amount of solvent used to dissolve a sample is much larger than the sample itself. If the solvent contains some of the analyte as an impurity, this would lead to a positive bias. 4. Introduction of contaminants from reaction of the solvent with vessel walls. This sometimes happens with high temperature sample treatments. 50 Decomposing with Acid or Base Samples are often treated with mineral acids or solutions of alkali metal hydroxides. A suspension of the sample is heated at the boiling point of the liquid until it is dissolved. 51 Hydrochloric Acid Concentrated hydrochloric acid is widely used to dissolve inorganic samples. It is not as useful to decompose organic samples. It can be used to dissolve many metal oxides and metals that are oxidized more easily than hydrogen. Concentrated HCl is about 12 M. When it is heated, HCl gas is lost, and it forms a constant boiling mixture that is 6 M (b.p. 110°C). 52 Nitric Acid Hot concentrated nitric acid is a strong oxidizing agent that dissolves common metals except for Al and Cr. Organic samples can be treated with hot nitric acid alone or combined with other acids or oxidizing agents like H2O2 to determine their trace metal content. This decomposition process is called wet ashing. Its purpose is to destroy the organic part of a sample prior to determining inorganic constituents. 53 Sulfuric Acid Concentrated sulfuric acid has a high b.p. (~340°C). This allows it to be used to dissolve and decompose many materials. Most organic compounds are decomposed (converted to carbon dioxide and water). Many metals dissolve in the hot acid. 54 Perchloric Acid Hot concentrated perchloric acid will dissolve some iron alloys and stainless steels that are not dissolved by other mineral acids. It is sold in concentrations of 60 to 72%. Warning: Hot concentrated perchloric acid can explode if it comes in contact with organic materials. 55 Oxidizing Mixtures Combinations of acids or mixtures of acids with oxidizing agents can accelerate wet ashing procedures. Aqua regia is a mixture of 3 volumes of hydrochloric acid and 1 volume of nitric acid. Bromine or hydrogen peroxide are sometimes combined with mineral acids to improve oxidation of organic materials. 56 Hydrofluoric Acid Hydrofluoric acid is useful to decompose silicate rocks and minerals (to determine species other than silica). The silicon is converted to SiF4. After the decomposition, the excess hydrofluoric acid is driven off by evaporation with sulfuric acid or perchloric acid. It is important to completely remove the excess because F- ion forms very stable complexes with many cations. This can interfere with the determination of the cations. 57