W9 Synaptic Transmission & Neuromuscular Junction PDF
Document Details
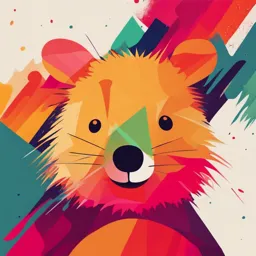
Uploaded by SafeCoral
Ross University
Jennifer Connolly
Tags
Related
- YR1 Lecture 1H - Skeletal Muscle 2021 PDF
- Presbyterian University College Physician Assistantship PDF
- Chapter 8: Synaptic Transmission and the Neuromuscular Junction PDF
- Chapter 10: Structure and Function of Muscles PDF
- Synaptic Transmission & Neuromuscular Junction PDF
- Synaptic and Neuromuscular Junction Transmission 2024 PDF
Summary
These notes on synaptic transmission and the neuromuscular junction cover learning objectives, defining synapses, and describing chemical neurotransmission in detail. They also cover the recycling of presynaptic vesicles and the formation of acetylcholine. Ideal for undergraduate physiology students.
Full Transcript
SYNAPTIC TRANSMISSION & NEUROMUSCULAR JUNCTION (NMJ) Jennifer Connolly, PhD Email: [email protected] Abbreviations: ACh: acetylcholine AChE: acetylcholinesterase AChR: acetylcholine receptor AP: action potential ChAT: Choline Acetyltransferase EPP: end plate potential GJ: gap junction MEPP...
SYNAPTIC TRANSMISSION & NEUROMUSCULAR JUNCTION (NMJ) Jennifer Connolly, PhD Email: [email protected] Abbreviations: ACh: acetylcholine AChE: acetylcholinesterase AChR: acetylcholine receptor AP: action potential ChAT: Choline Acetyltransferase EPP: end plate potential GJ: gap junction MEPP: miniature end plate potential NMJ: neuromuscular Junction NT: neurotransmitters 1 LEARNING OBJECTIVES 1. Define the terms synapse and synaptic transmission and describe the sequence of events which occur during neuromuscular transmission. 2. Describe chemical neurotransmission; list and describe in the correct temporal sequence of events beginning with arrival of a wave of depolarization at the presynaptic membrane and ending with a graded potential generated at the postsynaptic membrane. 3. Describe the recycling of presynaptic vesicles and the formation of acetylcholine (ACh). 4. Define the terms motor end plate and end plate potential. 5. Distinguish between an end plate potential and an action potential. 6. List the possible sites for altering neuromuscular transmission in skeletal muscle and provide examples of agents that could be used therapeutically at the NMJ. Review the following lectures before this series of physiology lectures: • • • • • Gross Anatomy: Introduction to the Nervous System Histology: Nervous Tissue Histology: Muscle Physiology: Membrane Potential Physiology: Action Potential 2 Synapses: Points of communication between electrically active cells • • • A synapse is a functional connection between two neurons or between a neuron & another cell type (e.g., a muscle cell, gland cell) Here, nerve impulses (i.e., action potentials) are transmitted from the pre-synaptic cell to the post-synaptic cell across the synapse. This process is called Synaptic Transmission & involves a number of steps. • Synapses are points of communication between electrically active cells. The axon of a neuron extends to a target cell (another neuron, a muscle cell, a gland cell) and the functional connection that forms between the two cells is termed a synapse. Here, nerve impulses (action potentials) are transmitted from the first cell (pre-synaptic cell) to the next cell (post-synaptic cell). This process of communication between the pre- and post-synaptic cells across the synapse is termed synaptic transmission and involves a number of steps. 3 The most common type of synapse is a chemical synapse • Here, the pre-synaptic cell and post-synaptic cell are not in contact • Action potential in presynaptic cell propagates to the axon terminal • Causes release of a chemical (neurotransmitter) into the synapse. • Neurotransmitter diffuses across the synapse & binds to its receptor on the post-synaptic cell. • Neurotransmitter receptors are often ion channels – neurotransmitter binding opens (or closes) ion channels altering movement of ions into/out of post-synaptic cell. • Depolarization of the post-synaptic cell membrane can lead to opening of voltage gated Na+ & K+ channels → action potential in the post synaptic • Chemical synapses, the most common type of synapse, involve the transmission of chemical information from one cell to the next. Here, electrical activity in the presynaptic neuron stimulates release of a chemical (a neurotransmitter) that binds to receptors located in the plasma membrane of the postsynaptic cell. • At chemical synapses, there is no intercellular continuity, and thus no direct flow of current from pre- to postsynaptic cell. Synaptic current flows across the postsynaptic membrane only in response to the release of neurotransmitters, which open or close postsynaptic ion channels after binding to receptor molecules. Signal transmission across a chemical synapse is delayed by about 0.5 ms - the time required for release and diffusion of neurotransmitter and the response of the postsynaptic cell to it. 4 A chemical synapse in more detail Major functional components of a chemical synapse include: • Pre-synaptic cell/neuron: A neuron (nerve cell) that releases a neurotransmitter as a result of an action potential entering its axon terminal. • Synapse/synaptic cleft: The junction across which a nerve impulse is transmitted from an axon terminal to a neuron, muscle cell or a gland • Post-synaptic cell: A cell (neuron/muscle cell/gland cell) that receives the neurotransmitter after it has crossed the synapse. • Axon terminal: Also called synaptic boutons or terminal boutons, axon terminals are small swellings that are found at the terminal ends of axons. They are typically the sites where synapses with other neurons are found, and neurotransmitters are stored there to communicate with other neurons/cells via these synapses. • Neurotransmitter: a chemical substance that is released at the end of a nerve fiber by the arrival of a nerve impulse and, by diffusing across the synapse or junction, causes the transfer of the impulse to another nerve fiber, a muscle fiber, or some other structure. Examples of neurotransmitters include acetylcholine, dopamine, norepinephrine, histamine, serotonin, gamma-aminobutyric acid (GABA). • Receptor for neurotransmitter: On the post-synaptic membrane are receptors for neurotransmitter. These are typically ligand-gated ion channels, binding of neurotransmitter (ligand) causing opening or closing of the ion channel and altering the flow of ions across the post-synaptic membrane, • Synaptic vesicles: Small electron dense vesicles that are clustered in the pre-synaptic terminals. They store neurotransmitters and release them by calcium-triggered exocytosis. • Voltage-gated calcium channels: Voltage-gated ion channels found in the membrane of excitable cells (neurons, muscle cells) with a permeability to calcium ions (Ca2+). At resting membrane potential, these channels are closed. They are activated (i.e., opened) at depolarized membrane potentials. When these channels open, calcium moves into the cell (influx) down a huge concentration gradient. Increased intracellular calcium concentration in the pre-synaptic neuron stimulates vesicle exocytosis. 5 STEPS IN SIGNAL TRANSMISSION AT CHEMICAL SYNAPSES Transmission at the chemical synapse is initiated when an action potential (AP) arrives at the terminal of the presynaptic neuron. The change in membrane potential caused by arrival of the AP leads to the opening of voltage-gated calcium (Ca2+) channels in the presynaptic membrane permitting a rapid influx of Ca2+. Elevation of presynaptic Ca2+ concentration stimulates fusion of neurotransmitter-containing synaptic vesicles with the pre-synaptic plasma membrane and release of vesicle contents into the synaptic cleft. Following exocytosis, neurotransmitter diffuses across the synaptic cleft and binds to specific receptors on the membrane of the postsynaptic cell. Binding of neurotransmitter to its receptors causes channels in the postsynaptic membrane to open (or sometimes to close), thus changing the ability of ions to flow into (or out of) the postsynaptic cells. The resulting neurotransmitterinduced current flow alters conductance and the membrane potential of the postsynaptic neuron, increasing or decreasing the probability that the neuron will fire an action potential. In this way information is transmitted from one neuron to another neuron or cell. 6 SYNAPSE OF A SOMATIC MOTOR NEURON AXON WITH A SKELETAL MUSCLE FIBER: NEUROMUSCULAR JUNCTION (NMJ) • The neurons that stimulate skeletal muscles to contract are somatic motor neurons. • These neurons project their axons to skeletal muscles & axons innervate individual muscle fibers (cells). • The point of communication between a motor neuron and a skeletal muscle fiber is called a Neuromuscular Junction (NMJ). An example of a chemical synapse is the Neuromuscular Junction (NMJ) - the synapse of a somatic motor neuron with a skeletal muscle fiber. The skeletal muscle fibers are innervated by large, myelinated nerve fibers that originate from large motor neurons in the anterior horns of the spinal cord. Each nerve fiber after entering the muscle belly, normally branches and innervates from three to several hundred skeletal muscle fibers. Each nerve ending makes a junction with the muscle fiber near its midpoint. With the exception of about 2% of the muscle fibers, there is only one such junction per muscle fiber. 7 The NMJ has three major components: Axon terminal of pre-synaptic neuron 1. Pre-synaptic axon terminal • • 2. Synaptic cleft • Myelin sheath Contains acetylcholine-filled synaptic vesicles & mitochondria Pre-synaptic membrane lined with voltagegated Na+, K+ & Ca2+ channels & proteins involved with vesicle fusion – active zone. Filled with fibrous matrix that contains acetylcholine (ACh) deactivating enzyme acetylcholinesterase (AChE) Membrane of post-synaptic cell (muscle fiber) 3. Postsynaptic membrane of the skeletal muscle fiber • • • Called the Motor End Plate Folded membrane (sarcolemma) lined with acetylcholine (ACh) receptors Voltage-gated Na+ & K+ channels in adjacent (neighboring) membrane. Like all other synapses, the Neuromuscular Junction (NMJ) has three components: (1) the motor nerve fiber’s presynaptic axon terminal filled with synaptic vesicles and mitochondria (2) the synaptic cleft, and (3) the postsynaptic membrane of the skeletal muscle fiber. In the axon terminal are many mitochondria that supply ATP for synthesis of excitatory neurotransmitter Acetylcholine (ACh). Acetylcholine is synthesized in the cytoplasm of the terminal & stored in synaptic vesicles. On the postsynaptic side of the NMJ, the muscle cell membrane (sarcolemma) that lies opposite the axon terminal is modified into a Motor End Plate, a series of folds (junctional folds). Along the upper edge of each fold, acetylcholine receptors (AChRs) cluster together. Between the axon and the muscle, the synaptic cleft is filled with a fibrous matrix whose collagen fibers hold the axon terminal and the motor end plate in the proper alignment. The matrix contains large quantities of the enzyme acetylcholinesterase (AChE) that rapidly deactivates acetylcholine by degrading it into acetate and choline after it has been released from the synaptic vesicles. 8 STEPS IN NEUROMUSCULAR TRANSMISSION 1. Action potential in a MOTOR NEURON is propagated to the presynaptic terminal. 2. Depolarization of the presynaptic membrane triggers opening of VOLTAGE-GATED Ca2+ CHANNELS & Ca2+ entry. 3. Ca2+ triggers exocytosis of ACETYLCHOLINE (ACh) from synaptic vesicles. 4. ACh diffuses across the space between the nerve & muscle cell and binds to ACh RECEPTORS located in the MOTOR END PLATE of the muscle cell membrane. 5. ACh binding triggers opening of these LIGAND-GATED ION CHANNELS, leading to Na+ ENTRY into the muscle cell & K+ EXIT. 6. Local current flow occurs between the depolarized motor end plate & the adjacent muscle membrane. 7. This local current flow alters membrane potential to threshold and opens VOLTAGE-GATED Na+ CHANNELS in the adjacent muscle membrane. 8. This initiates an action potential which is propagated along the muscle fiber membrane. 9. ACh is degraded by ACETYLCHOLINESTERASE terminating the muscle cell’s response. Like the sequence of events that occurs at a chemical synapse, the major steps involved with neuromuscular transmission are listed here. Note: • An increase in pre-synaptic Ca2+ concentration is the key stimulator of vesicle release & thus, neuromuscular transmission. Ca2+ entry is facilitated by the steep concentration gradient of Ca2+ across the presynaptic membrane (the external Ca2+ concentration is approximately 10-3M, whereas the internal Ca2+ concentration is approximately 10-7M). Thus, opening of voltage-gated Ca2+ causes a rapid influx of Ca2+ into the presynaptic terminal, with the result that the Ca2+ concentration of the cytoplasm in the terminal transiently rises to a much higher level. • ACh binding to muscle membrane acetylcholine receptors triggers opening of these nonspecific cation channels, leading to a relatively large movement of Na+ into the muscle cell compared to a smaller movement of K+ outward (into the synapse). • ACh is degraded to choline and acetate by acetylcholinesterase (AChE), thus terminating the signal transduction. 9 1. Vesicles synthesized in the neuronal cell body & transported to the nerve terminals. SYNAPTIC VESICLE CYCLE IN THE PRESYNAPTIC NERVE TERMINALS 2. Vesicles filled with neurotransmitter (NT) & translocate to the release site (active zone) on pre-synaptic plasma membrane. 3. Vesicle docks & becomes primed. 4. Ca2+ enters the axon terminal triggering vesicle fusion with pre-synaptic membrane & exocytosis. clathrin 5. Vesicle wall is coated with clathrin facilitates endocytosis. 6. Vesicle becomes uncoated & fuses with early endosome. 7. Vesicle is reformed and buds off early endosome – recycled. Within the presynaptic terminal of the NMJ are many small, clear synaptic vesicles filled with the neurotransmitter acetylcholine (ACh). The vesicles and the proteins contained in their walls are synthesized in the neuronal cell body & transported to the nerve terminals. On arrival at the nerve terminal, ACh (which is produced locally) is taken up into the vesicles. These synaptic vesicles translocate to & become docked at specific release sites on the pre-synaptic membrane, called the active zone. Ca2+ triggers fusion and exocytosis. After exocytosis, endocytosis via clathrin-coating of the vesicles recovers membrane components and recycles them to an endosome compartment in the terminal (clathrin-mediated endocytosis). Synaptic vesicles may then be reformed within the terminal by budding from an endosome and re-used, or they may be transported back to the cell body for turnover and degradation. 10 PROCESS OF VESICLE FUSION WITH THE PRESYNAPTIC MEMBRANE 1. Docking ACh-filled vesicle aligns with presynaptic plasma membrane at the active zone. 2. Priming 3. Fusion Vesicle membrane protein SYNAPTOBREVIN & presynaptic plasma membrane proteins SYNTAXIN & SNAP-25 bind to each other forming a SNARE COMPLEX. This brings the vesicle membrane into close proximity with the presynaptic plasma membrane. Ca2+ enters the presynaptic terminal via voltage-gated Ca2+ channels & stimulates vesicle fusion with the plasma membrane. Ca2+ binds to synaptic vesicle protein SYNAPTOTAGMIN allowing it to insert into the membrane and bind with the SNARE complex. The membranes are permitted to fuse. 4. Exocytosis Once the vesicle and plasma membranes fuse, ACh is released into the synaptic cleft. A motor neuron AP stimulates release of ~100200 synaptic vesicles, each containing ~10,000 molecules of ACh. Botulinum toxin, a neurotoxin of Clostridium botulinum is considered one of the most toxic substances. It cleaves SNARE proteins, eliminating vesicle exocytosis & inhibiting neuromuscular transmission. Causes muscle paralysis, weakness & death. Commercial preparations (BOTOX®) smooth wrinkles by paralysis of facial muscles. Many proteins are involved in the fusion and recycling of synaptic vesicles. The SNARE proteins comprise the force-generating molecular machinery for membranemembrane fusion. After docking of the vesicle near the presynaptic membrane, the vesicle fusion process begins. SNARE (SNAP REceptor) proteins on the synaptic vesicle (synapatobrevin) and pre-synaptic plasma membrane (syntaxin & SNAP-25) coil around each other forming a complex (SNARE complex) that brings together the two membranes. Ca2+ then binds to vesicle protein synaptotagmin, causing the cytoplasmic region of this protein to insert into the plasma membrane, bind to SNAREs and catalyze membrane fusion. Note: Toxins that cleave the SNARE proteins block NT release (e.g., botulinum toxin) 11 ACETYLCHOLINE SYNTHESIS & RECYCLING 1. Acetylcholine (ACh) is synthesized from Acetyl Coenzyme A & Choline in the presence of enzyme Choline Acetyltransferase (ChAT). Choline Acetyltransferase Na+ 2. In presynaptic neurons, acetyl-CoA is synthesized from pyruvate in mitochondria & choline enters via a Na+-dependent transporter. 3. Once formed, ACh is transported into & concentrated in vesicles. 4. ACh released into the synaptic cleft is enzymatically degraded by Acetylcholinesterase (AChE) in the synaptic cleft. Acetylcholinesterase (AChE) ACh is the chemical messenger which carries the signal between a motor neuron terminal and the skeletal muscle fiber. Acetylcholine is formed from acetyl CoA and choline by the action of the enzyme choline acetyltransferase (ChAT). Choline is a compound that is obtained by dietary means. It can readily cross the blood brain barrier from the circulation and is taken up by cholinergic (ACh releasing) nerve terminals. Acetyl CoA is synthesized in the mitochondria of all cells as a by-product of the pyruvate dehydrogenase complex. Much of the acetyl CoA produced by this process is used to generate ATP in the TCA/Krebs cycle. However, in cholinergic cells, some of the acetyl CoA is transported across the mitochondrial membrane into the cytoplasm for use in the synthesis of ACh. ACh is stored in vesicles for subsequent release. Effective transmission across chemical synapses requires not only release of neurotransmitter and activation of the receptor on the postsynaptic membrane, but also a rapid and efficient mechanism for removal of the transmitter. ACh removal is accomplished by enzymatic destruction of the neurotransmitter by acetylcholinesterase (AChE) present in the synaptic cleft. AChE rapidly hydrolyzes ACh to choline and acetate. Choline is taken back into the presynaptic terminal on a Na+-choline cotransporter & recycled. Note: Acetylcholinesterase inhibitors prolong ACh action (e.g., neostigmine). 12 ACETYLCHOLINE RECEPTORS IN THE MOTOR END PLATE & VOLTAGE-GATED ION CHANNELS IN THE ADJACENT SARCOLEMMA Voltage-gated Na+ channel Voltage-gated K+ channel Acetylcholine Receptor Binding of Acetylcholine (ACh) to the ACh receptors (AChR) in the motor end plate triggers the opening of these ligand-gated ion channels. Change in membrane potential to threshold causes opening of voltage-gated Na+ channels and voltagegated K+ channels in the muscle cell membrane (sarcolemma) adjacent to the motor end plate 13 NICOTINIC ACETYLCHOLINE RECEPTORS • There are two subtypes of nicotinic AChR, Nn & Nm • Nn are found in autonomic ganglia, Nm in the NMJ. Nicotinic Acetylcholine Receptor • Nn & Nm are both ligand-gated non-specific cation channels that permit passage of both Na+ & K+ on binding of ACh. • Permeability of the post-synaptic membrane to Na+ & K+ are essentially equal; relative movement of these ions depends on electrochemical driving forces. • Net driving force for Na+ >>> K+ The nicotinic acetylcholine receptor (nAChR) on the post-synaptic membrane binds ACh, opening a non-specific cation channel. Net Na+ influx depolarizes the post-synaptic membrane. ➢ Considerably more Na+ moves inward than K+ moves outward, bringing about a local depolarization in the post-synaptic membrane. Acetylcholine receptors (AChRs) are divided into 2 main types based on their pharmacologic properties: muscarinic AChRs & nicotinic AChRs. There are 5 subtypes of muscarinic receptor (M1-M5) and two subtypes of nicotinic receptor (Nn & Nm). Nicotinic acetylcholine receptors Nn & Nm are both ligand-gated ion channels which are activated by binding of acetylcholine. Nm nicotinic AChRs are found in the NMJ. When acetylcholine binds to the receptor, the channel gate opens and allows monovalent cations (Na+ and K+) to flow through. Net Na+ influx through this nonspecific cation channel dominates K+ efflux, and the resulting increase in Na+ conductance produces a depolarizing potential in the post-synaptic membrane. 14 MINIATURE END PLATE POTENTIAL (MEPP) & END PLATE POTENTIAL (EPP) Membrane potential (mV) Nicotinic ACh Receptor Miniature End Plate Potentials (MEPPs) -89 AT REST NO AP IN ALPHA MOTOR NEURON -90 -91 STIMULATED AP IN ALPHA MOTOR NEURON Membrane potential (mV) -50 -60 -70 -80 -90 End Plate Potential (EPP) • Release of ACh from 1 synaptic vesicle. • Slight depolarization (~0.5 mV) = MEPP. • Occurs spontaneously, unstimulated. • AP in alpha motor neuron stimulates release of 100-200 vesicles of ACh. • More sodium enters the post-synaptic cell leading to a larger depolarization (~40 mV) = EPP. Experiments in which an intracellular microelectrode is used to record the membrane potential of a muscle cell have shown that at rest (i.e. not stimulated) individual vesicles of ACh are released spontaneously at the NMJ. The amount of ACh released from one vesicle is sufficient to produces a slight depolarization in the motor end plate called a Miniature End Plate Potential (MEPP), which is about 0.5 mV in amplitude. This means that one vesicle of ACh activates enough nAChRs to allow enough sodium influx/potassium efflux to change post-synaptic resting membrane potential from about -90 mV to about -89.5 mV. When a nerve impulse reaches the axon terminal, ~100-200 vesicles are released simultaneously or in rapid series. This results in opening of more nicotinic ACh receptors and more sodium entry into the muscle cell resulting in a larger depolarization in the motor end plate called an End Plate Potential (EPP). The magnitude of a typical EPP is around 40mV, meaning that post-synaptic resting membrane potential changes from about -90mV to about -50mV. • Depolarization: movement of a cell’s membrane potential to a more positive value (i.e., movement of membrane potential closer to zero from resting membrane potential of -70 mV). 15 END PLATE POTENTIALS ARE GRADED POTENTIALS • EPPs are local, brief changes in the end plate membrane potential (-90 mV to -50 mV depolarization). • EPPs result from Na+ entry (and K+ efflux) through nicotinic acetylcholine receptors • Na+ ions diffuse into the muscle from the site of origin in all directions. • The magnitude of voltage change generated by EPPs decreases gradually with distance from the site of origin, eventually disappearing. • EPPs are therefore, GRADED POTENTIALS. When a motor nerve axon innervating a muscle fiber is stimulated, a depolarization in the muscle membrane in the region of the motor end plate is observed after a few milliseconds delay. The delay represents the time required for the release of ACh, its diffusion across the synapse, and activation of the postsynaptic AChRs. This depolarization, known as the End Plate Potential (EPP) is an example of an excitatory post-synaptic potential. It is produced by transient opening of ion channels which are activated by ACh and selectively permeable to monovalent cations such as Na + and K+. The increase in Na+ conductance drives membrane potential to a more positive value in the motor end plate region. If membrane potential is measured at distances away from the active area in the motor end plate where Na+ and K+ are moving across the plasma membrane, the amplitude of the potential change gradually diminishes. This decrement with distance occurs because the EPP originates at the end plate region and spreads away from this site. Thus, the EPP is an example of a graded potential. 16 ACTIVATION OF VOLTAGE-GATED SODIUM & POTASSIUM CHANNELS IN THE SARCOLEMMA (ADJACENT TO THE MOTOR END PLATE) • Voltage-gated channels open & close in response to changes in membrane potential. Voltage-gated Na+ channel Voltage-gated K+ channel • EPP depolarizes the membrane potential from -90 mV to threshold (-55 mV) causing opening of voltage-gated channels in the adjacent sarcolemma. • Voltage-gated Na+ channels open more quickly, resulting in Na+ influx and depolarization. Voltage-gated K+ channels open slowly resulting in K+ efflux and repolarization. • Once initiated, the Action Potential propagates along the sarcolemma and T-tubules. ➢ Action potential in alpha motor neuron is transmitted via a chemical synapse (NMJ) to the skeletal muscle fiber. If the EPP depolarizes the cell membrane to a threshold level, it will fully activate voltage-gated sodium & potassium channels in the adjacent membrane and produce an action potential in the muscle cell membrane. The action potential will then stimulate the muscle cell to contract There is a pronounced delay between the time that the presynaptic motor neuron is stimulated and when the EPP occurs in the postsynaptic muscle cell. Such a delay is characteristic of all chemical synapses and relates to the time taken for neurotransmitter release and diffusion across the synaptic cleft. 17 SUMMARY - NEUROMUSCULAR TRANSMISSION 1. Action potential in a MOTOR NEURON propagated to the axon terminal. 2. Presynaptic membrane depolarization opens VOLTAGE-GATED Ca2+ CHANNELS & Ca2+ entry. 3. Ca2+ binds to vesicle protein synaptotagmin, stimulating its interaction with SNARE proteins. Vesicles fuse with the presynaptic membrane stimulating exocytosis of ACETYLCHOLINE (ACh) from synaptic vesicles. 4. ACh diffuses across the synaptic cleft & binds to NICOTINIC ACETYLCHOLINE RECEPTORS located in the MOTOR END PLATE of the muscle cell membrane. 5. ACh binding triggers opening of these NON-SPECIFIC CATION CHANNELS, leading to Na+ ENTRY into the muscle cell & K+ EFFLUX. 6. Na+ entry through these LIGAND-GATED ION CHANNELS exceeds K+ efflux resulting in local change in membrane potential of ~40mV – End Plate Potential (EPP) 7. This local current flow alters membrane potential to threshold (-55mV) and opens VOLTAGE-GATED Na+ & K+ CHANNELS located in the adjacent muscle membrane. 8. This initiates an action potential which is propagated along the muscle fiber membrane. 9. ACh is degraded by ACETYLCHOLINESTERASE terminating the muscle cell’s response. 18 Neuronal Na+ channel Tetrodotoxin (TTX) blocks Na+ channels inhibiting NMJ transmission. Ca2+ channel ω-Conotoxin is an irreversible blocker of pre-synaptic voltage-gated Ca2+ channels. Muscle Na+ channel µ-conotoxin & Tetrodotoxin (TTX) block voltage-gated Na+ channels in the muscle membrane adjacent to the motor-end plate. K+ channel Dendrotoxin (DTX) blocks voltage-gated K+ channels inhibiting repolarization of the presynaptic membrane. This prolongs AP duration, increasing Ca2+ entry & enhancing ACh release. ACh release Botulinum toxin blocks vesicle fusion & exocytosis of ACh by cleaving proteins of the SNARE complex. AChR Channel Succinylcholine is an AChR agonist. Not metabolized by AChE. Prolonged depolarization of the membrane. Post-synaptic voltage-gated Na+ channels inactivate - cannot reopen until membrane repolarizes. Muscle relaxes. D-Tubocurarine is an AChR antagonist. Blocks ACh action by reversibly binding & blocking AChR. EPP is diminished. Muscle AP cannot occur. Acetylcholinesterase (AChE) inhibitors Inhibit AChE and prolong the duration of the post-synaptic response to ACh. Extremely potent toxins (e.g., sarin gas). Reversible inhibitors (e.g., neostigmine) used in therapeutic treatment of Myasthenia Gravis. Used clinically to relax skeletal muscle during anesthesia. AGENTS AFFECTING NEUROMUSCULAR SIGNAL TRANSMISSION 1. Neuronal Na+ channel blockers e.g.,. pufferfish neurotoxin tetrodotoxin, TTX) block Na+ entry via voltage-gated Na+ channels, inhibiting depolarization and AP propagation. 2. Neuronal K+ channel blockers (e.g., mamba snake toxin, DTX) block K+ efflux via voltage-gated K+ channels, inhibiting repolarization, prolonging the duration of the AP. 3. Neuronal Ca2+ channel blockers (e.g., cone sail ω-conotoxin) inhibit vesicle fusion and ACh release. 4. Inhibition of ACh release: Certain toxins (e.g., Clostridium botulinum) cleave SNARE proteins preventing fusion of synaptic vesicles & NT exocytosis. 5. Muscle Na+ channel blockers (e.g., cone snail µ-conotoxin) block Na+ entry through muscle membrane voltage-gated Na+ channels, inhibiting muscle AP generation. 6. AChR agonist e.g., succinylcholine is used clinically for facilitating endotracheal intubation and to relax skeletal muscle during surgery. It binds to nicotinic ACh receptors in the same manner as ACh but is resistant to AChE. As such, succinylcholine permits prolonged Na+ entry and K+ efflux (prolonged depolarizations) at the motor end plate. Threshold is reached and voltage-gated Na+ channels in the adjacent membrane open, stimulating APs in the muscle membrane. After an initial opening, muscle membrane voltage-gated Na+ channels close and will not reopen until the membrane is repolarized. As such, succinylcholine administration manifests in muscle as a brief period of repetitive excitation that may elicit transient & repetitive muscle excitation (twitches/fasciculations) followed by blocking of neuromuscular transmission and flaccid paralysis. AChR antagonist e.g., D-tubocurarin binds the nicotinic ACh receptor and blocks the binding of ACh resulting in flaccid paralysis of skeletal muscle. 7. Inhibition of AChE leads to an accumulation of ACh in the synaptic cleft, causing sustained activation of the nicotinic AChR and sustained depolarization of the postsynaptic membrane. Although the initial depolarization would lead to an AP and muscle contraction, sustained depolarization would prevent voltage-gated Na+ channels from recovering from inactivation. The result is a flaccid muscle paralysis. Reversible AChE inhibitors are used therapeutically in Myasthenia Gravis to enhance ACh levels in the synapse and improve muscle tone. 19 By the end of this lecture, you should be able to answer the following questions: • What neurotransmitter is released at the neuromuscular junction? • What ion stimulates synaptic vesicle fusion with the pre-synaptic membrane? • By what process are synaptic vesicles recycled? • What does acetylcholine esterase do? Where is it found? • What stimulates opening of nicotinic acetylcholine receptors? • What ions preferentially move through nAChRs? In what direction do these ions flow? • What is a major function of an End Plate Potential in the NMJ? • What is the motor end plate? What is the active zone? • Nicotinic acetylcholine receptors are ligand-gated ion channels – what does “ligand-gated” mean? • Where are nicotinic acetylcholine receptors located? • What does choline acetyltransferase do? Where is it found? • What happens to acetylcholine once it is released into the synaptic cleft? • What controls opening of the sodium and potassium channels located in the adjacent region of the muscle plasma membrane (i.e., the region outside the motor end plate )? • What is the stimulus for opening voltage-gated calcium channels located in the pre-synaptic membrane? • To what synaptic vesicle protein does calcium bind to stimulate vesicle exocytosis? • Nicotinic acetylcholine receptors are non-specific cation channels – what does this mean? • What are the SNARE proteins? Where are they located? • Relating to neuromuscular transmission, what does BOTOX do? • How does an end plate potential differ from an action potential (think about the types of channels involved, the properties of each potential)? 20