Eutrophication and Acid Deposition Explained - PDF
Document Details
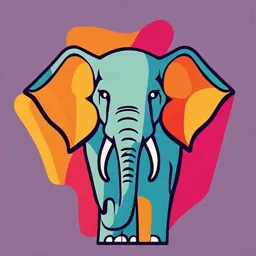
Uploaded by EnergySavingHoneysuckle
Tags
Summary
This document discusses the processes of eutrophication and acid deposition, highlighting their causes, effects on ecosystems, and the impact of human activities. It explains how the addition of nitrates and phosphates, as well as emissions from various sources, contribute to these environmental issues, with a strong emphasis on understanding and mitigation.
Full Transcript
All biogeochemical cycles are susceptible to perturbations by human activity. In fact humans are now the main cause of some transfers. Some of the most difficult environmental challenges faced by society spring from these transfers. The purpose of this section is to discuss two of them in more detai...
All biogeochemical cycles are susceptible to perturbations by human activity. In fact humans are now the main cause of some transfers. Some of the most difficult environmental challenges faced by society spring from these transfers. The purpose of this section is to discuss two of them in more detail: eutrophication and acid deposition. A third example, global climatic change—largely resulting from disruptions in the carbon and nitrogen cycles—is of such significance that Chapter 8 is devoted to it. Eutrophication Eutrophication is a natural process of nutrient enrichment of water bodies that leads to greater productivity. The process is accelerated by human activities that mobilize nitrates and phosphates. Phosphorus and nitrogen are often the two main limiting factors for plant growth in aquatic ecosystems. Systems with relatively low nutrient levels, oligotrophic ecosystems, have quite different characteristics from those with high nutrient levels (eutrophic), as summarized in Table 5.3. Mesotrophic bodies have characteristics between these two extremes. The progression from an oligotrophic to eutrophic condition, through the process of succession discussed in Chapter 4, may take thousands of years. This rate is influenced by the geological makeup of the catchment area and the depth of the receiving waters. Catchments with fertile soils will progress more quickly than those with soils lacking in nutrients. Depth is important, because shallower lakes tend to recycle nutrients more efficiently. TABLE 5.3 Characteristics of Oligotrophic and Eutrophic Water Bodies Characteristic Oligotrophic Eutrophic Nutrient cycling low high Productivity (total biomass) low high Species diversity high* low Relative numbers of “undesirable” species low high Water quality high low *Lakes that are extremely non-productive (e.g., high mountain lakes) will have low species diversity What Causes Eutrophication? Cultural eutrophication (eutrophication caused by human activity) speeds up the natural eutrophication process through the addition of phosphates and nitrates to the water body. As lakes become shallower as a result of this input, nutrients are used more efficiently, productivity increases, and eutrophication progresses. This is a classic example of a positive feedback loop, with change in the system promoting even more change in the same direction. Additional phosphates and nitrates come from many different sources (Table 5.4), and in accordance with the law of conservation of matter, they do not disappear but accumulate in aquatic ecosystems. In total, between 8.5 and 9.5 tonnes of phosphorus find their way into the ocean from the 20 million tonnes mined in the world each year. This is approximately eight times the natural amount. TABLE 5.4 Main Nutrient Sources Contributing to Cultural Eutrophication Runoff from fertilizers (N and P) feedlots (N and P) land use change, such as cultivation, construction, mining natural sources Discharge of detergents (P) untreated sewage (N and P) primary and secondary treated sewage (N and P) Emissions from internal combustion engines (dissolved nitrogen oxides) What Are the Effects? This enrichment promotes increased growth of aquatic plants, particularly favouring the growth of floating phytoplankton over benthic plants rooted in the substrate. As the benthic plants become out-competed for light by the phytoplankton, they produce less oxygen at depth. Oxygen is critical for the maintenance of more diverse, oxygen-demanding fish species such as trout and other members of the salmonid family, which also start to decline in number. The oxygen produced by photosynthesis by the phytoplankton tends to stay in the shallower water, escaping back to the atmosphere rather than replenishing supplies at greater depths. Oxygen depletion is further exacerbated by the decay of the large mass of phytoplankton produced. Dead matter filters to the bottom of the lake where it is consumed by oxygen-demanding decomposers. Once broken down, nutrients may be returned to the surface through convection currents and provide more food for more phytoplankton and algae. Blue-green algae replace green algae in eutrophic lakes, which further exacerbates the problem, since most blue-green algae are not consumed by the next trophic level, the zooplankton. These effects of oxygen depletion in a water body also occur with the addition of excess organic matter. Under natural conditions, water is able to absorb and break down small amounts of organic matter, with the amount depending on the size, flow, and temperature of the receiving water body. The greater the size and flow and the lower the temperature, the greater the ability to absorb organic materials and retain oxygen levels. When organic wastes are added to a body of water, the oxygen levels fall as the number of bacteria rises to help break down the waste. This is the oxygen sag curve (Figure 5.14) and is measured by the biological oxygen demand (BOD), the amount of dissolved oxygen needed by aerobic decomposers to break down the organic material in a given volume of water at a certain temperature over a given period. At the discharge source, the oxygen sag curve starts to fall, and there is a corresponding rise in the BOD. As distance from the input source increases and the bacteria digest the wastes, then the oxygen content returns to normal, and the BOD falls. FIGURE 5.14 Oxygen sag curve and biological oxygen demand (BOD). Major sources of nitrates and phosphates, such as runoff from feedlots and sewage discharge, also contain large amounts of oxygen-demanding wastes. Heat is another source of oxygen stress. The overall result is a progression to a less useful and less healthy water body. The composition of the fish species changes to those less dependent on high oxygen levels, species generally less desirable for human purposes. Populations of waterfowl may fall as aquatic plants die off. The water becomes infested with algae, aquatic weeds, and phytoplankton, making swimming and boating unpleasant and giving off unpleasant odours. Water treatment for domestic or industrial purposes becomes more expensive. What Can We Do about It? The main way to control eutrophication is to limit the input of nutrients into the water body (Table 5.4). Domestic and animal wastes must be treated to remove phosphates. Advanced treatment can remove up to 90 per cent of these wastes. More difficult problems occur with diffuse, non-point sources, such as runoff from urban areas and agricultural land. Such flows really have to be controlled at the source, since they enter the water body, by definition, in so many different locations. In the past, measures to control water pollution have been largely directed toward point sources of pollution, or single discharge points, such as effluent discharges from sewage plants or industrial processes. By and large, because of the high visibility of such sources, they are easy to identify and monitor, and pollution from such sources has fallen as a result. Increasing attention is now being directed toward the non-point sources (see Chapter 12). Eutrophication used to be considered a problem of smaller water bodies, but now entire areas of the world’s largest water bodies, the oceans, are becoming so oxygen deficient (hypoxic) that they are being described as “dead zones.” More than 400 such zones have been recognized (up from 49 in the 1960s), some as large as 70,000 km2. One of the largest and best-known areas is in the Gulf of Mexico, which receives all the excess fertilizers brought down from the Mississippi catchment. However, similar areas now occur in the waters off every continent, and researchers expect that their number and size will increase as global climatic change generates greater rainfall and greater runoff in many areas. Eutrophication was thought to have been largely addressed through pollution control some 30 years ago. Now it is apparent that the challenge is still there, but at a larger scale. The next section explores one of Canada’s most notable eutrophication challenges, Lake Erie, and notes some ways to address the problem. Lake Erie: An Example of Eutrophication Control In all parts of the country, many examples exist of eutrophic lakes. The best known are the Great Lakes, where phosphorus levels are an issue in the open waters of three of the four Canadian Great Lakes. Lake Erie has the most severe conditions, and phosphorus levels continue to increase. Lake Erie is a well-known case because of its size and importance. It is the second-smallest and also the shallowest of the Great Lakes, which collectively contain almost 20 per cent of the world’s fresh water. Erie has experienced considerable changes in fish species composition. Gone are the lake sturgeon, cisco, blue pike, and lake whitefish as the human population in the basin increased and water quality declined. Some 11 million people live in the Erie drainage basin, and 39 per cent of the Canadian and 44 per cent of the American shore is taken up by urban development. There is also considerable industrial use around the shore and intensive agricultural use throughout the basin. In the past, up to 90 per cent of the bottom layer of the central zone of the lake became oxygen deficient in the summer. Huge algae mats more than 20 metres long and a metre deep became common. Beaches were closed. The natural eutrophication that might have taken thousands of years was superseded by cultural eutrophication within the space of 50 years. In 1972, Canada and the US signed the Great Lakes Water Quality Agreement to try to come to grips with this problem. The signing of the 1985 Great Lakes Charter, in which the two countries agreed to take a cooperative and ecosystem-based approach to the lakes, further strengthened international efforts (See also Chapter 12). Since the 1970s, phosphorus controls, implemented under the Canada Water Act, have led to significant reductions in the phosphorus concentration of the water. Phosphate-based detergents were banned and municipal waste treatment plants upgraded. These measures led to improvement of water quality, but significant problems still remain. The controls are largely on point-source pollution, discharges that have a readily identifiable source, such as waste treatment plants and industrial complexes. However, much of the remaining nutrient load comes from non-point sources, such as runoff from agricultural fields, lawns, and construction sites, which are much more difficult to regulate. Phosphorus levels exceed objective levels in the western, eastern, and central basins. The levels of nitrite and nitrate concentrations are also rising, leading to increased eutrophication as a result of other nutrients. Synergistic effects between increasing phosphorus loads and invasive species exacerbate the problem. For example, zebra mussels (Chapter 4) are filter-feeders that remove the phosphorus from the water and convert it into a form easily usable by aquatic plants and algae. They selectively feed on edible algae, giving opportunity for expanded blooms of the toxic blue-green algae Microcystis. The latter can be toxic to animals, including humans, and also is not a preferred food for zooplankton and therefore affects the base of the food chain, particularly for fish larvae (Vanderploeg et al., 2009). This has led to the interesting situation in which some areas in the centre of the Great Lakes, particularly in Huron and Ontario, are phosphorus deficient and hence limit fish growth, whereas there is an excess of phosphorus in the littoral area related to the concentrating effect of the zebra mussels and growth of Microcystis. Animal feedlots are a major source of nutrients, such as phosphates and nitrates, which speed up eutrophication. Scientists have also found that the amount of water flowing into Lake Erie is a major influence on the size and severity of the dead, oxygen-deficient zone that forms in the lake every summer (Zhou et al., 2015). The extreme drought of 2012, for example, led to the largest dead zone ever recorded. Previously, it had been thought that phosphorus levels were primarily responsible, but now it seems that meteorological factors, particularly precipitation, can have a major effect. Very large inflows are associated with large algae blooms, whereas small inflows create larger dead zones, calling for management that pays greater attention to these factors. Lake Erie continues to be in trouble. Nowhere was this more clear than in Toledo, Ohio. In August 2014 citizens were not only unable to drink from their water supply from the lake but also advised not to shower. Hospitals limited admissions, stores closed, restaurants were empty. In response the city, following New Zealand’s lead, asked voters whether Lake Erie should have the same legal rights as a human being—the voters said “yes.” This gives organizations the right to sue a polluting entity on behalf of the lake. There are many legal ramifications of the initiative, but perhaps, most importantly it signifies a change in thinking regarding human use, and abuse, of the natural world. A fuller description of the implications of granting legal rights to rivers can be found in a policy brief by the International Water Resources Association (IWRA, 2019). Acid Deposition In 1966, fisheries researcher Harold Harvey was puzzled to find that the 4,000 pink salmon he had introduced to Lumsden Lake in the La Cloche Mountains southwest of Sudbury, Ontario, the previous year had all disappeared. Their passage upstream and downstream of the lake had been blocked. To unravel the mystery, he began to take more measurements of the lake and look into its history. The results were startling—not only had the salmon disappeared but many other species of fish indigenous to the lake were missing (Table 5.5). The reason soon became apparent. Between 1961 and 1971, Lumsden Lake had experienced a 100-fold increase in the acidity of its waters, as had many other lakes in the same region (Table 5.6). The changes had shifted the lakes outside the limits of tolerance of the species, as discussed in Chapter 3. The indigenous fish species, and many of the species upon which they depended for food, simply could not tolerate the new conditions and perished. They were victims of the effects of acid deposition. TABLE 5.5 Disappearance of Fish from Lumsden Lake 1950s Eight species present 1960 Last report of yellow perch 1960 Last report of burbot 1960–5 Sport fishery fails 1967 Last capture of lake trout 1967 Last capture of slimy sculpin 1968 White sucker suddenly rare 1969 Last capture of trout and perch 1969 Last capture of lake herring 1969 Last capture of white sucker 1970 One fish species present 1971 Lake chub very rare Source: H. Harvey, unpublished speech, based on Beamish and Harvey (1972). Reprinted with the author’s permission. TABLE 5.6 Lake Acidification in the La Cloche Mountains, 1961–71 Lake pH 1961 pH 1971 Broker6.8 4.7 David 5.2 4.3 George 6.5 4.7 Johnnie 6.8 4.8 Lumsden 6.8 4.4 Mahzenazing 6.8 5.3 O.A.S.5.5 4.3 Spoon 6.8 5.6 Sunfish 6.8 5.6 Grey 5.6 (1959) 4.1 Tyson 7.4 (1955) 4.9 Source: H. Harvey, unpublished speech, based on Beamish and Harvey (1972). What Is Acid Deposition? Acids are chemicals that release hydrogen ions (H+) when dissolved in water, whereas a base is a chemical that releases hydroxyl ions (OH−). When in contact, acids and bases neutralize each other as they come together to form water (H2O). Acidity is a measure of the concentration of hydrogen ions in a solution and is measured on the pH scale, which goes from 0 to 14 (Figure 5.15). The midpoint of the scale, pH 7, represents a neutral balance between the presence of acidic hydrogen ions and basic hydroxyl ions. The pH scale is logarithmic. A decrease in value from pH 6 to pH 5 means that the solution has become 10 times more acidic. If the number drops to pH 4 from pH 6, then the solution is 100 times more acidic. FIGURE 5.15 The acid (pH) scale. Precipitation, as either snow or rain, tends to be slightly acidic, even without human interference, because carbon dioxide in the atmosphere combines with water to form carbonic acid. Generally, “clean” rain has a pH value of 5.6. Acid rain is defined as deposition that is more acidic than this, and in Canada rainfall has been recorded with pH levels much lower. Acid deposition is a more generic term that includes not only rainfall but also snow, fog, and dry deposition from dust. What Causes Acid Deposition? The increases in acidity reflected in the pH levels of the lakes listed in Table 5.6 are due to human interference in the sulphur and nitrogen cycles. The largest sources are the smelting of sulphur-rich metal ores and the burning of fossil fuels for energy. These processes change the distribution of the elements among the various sources shown in Figures 5.3 and 5.4, and natural processes cannot deal with the buildup of matter. Increased amounts of sulphur and various forms of nitrogen are ejected into the atmosphere, where they may travel thousands of kilometres before being returned to the lithosphere as a result of depositional processes. Human activities account for more than 90 per cent of the sulphur dioxide and nitrogen oxide emissions in North America. Excessive sulphur is produced when ore bodies, such as copper and nickel, are roasted (smelted) at high temperatures to release the metal, with sulphur released into the atmosphere as a waste product of the process. A similar effect is created when sulphur-containing coal is burned as the energy source in power plants. In Canada, the smelting of metal ores accounts for most sulphur emissions (Figure 5.16). In the US, electrical utilities are the largest source, accounting for 70 per cent of emissions. The effects of these emissions became obvious fairly obvious early on around smelting plants such as those at Inco in Sudbury, Ontario, and in Trail, BC. Trees were destroyed over large areas. Now there are very encouraging signs of rehabilitation. FIGURE 5.16 Sulphur oxide emissions and their sources, 1990–2015. Source: Environment and Climate Change Canada (2017). © Her Majesty the Queen in Right of Canada, as represented by the Minister of the Environment, 2020. Note: The indicator reports air pollutant emissions from human activities only. The category “other sources” includes emissions from transportation (road, rail, air, and marine), off-road and mobile equipment, home firewood burning, incineration and waste, agriculture (livestock, crop production, and fertilizer), dust and fires, paints and solvents, building heating and energy generation, manufacturing, and other miscellaneous sources. These obvious signs of ecological damage were ignored for many years. However, as the implications became better known, industry began to build higher stacks to eject the waste farther into the atmosphere. Inco, for example, built a 381-metre “Superstack” in 1972. At the local scale, this improved matters somewhat but created problems elsewhere, especially in Quebec, as entire air masses became acidified and dropped their acid burdens over a larger area. Weather patterns are not random, and so acidified air masses tend to travel in the same kinds of patterns. In central and eastern Canada, as air masses travel from southwest to northeast, they bring a heavy pollution burden from the heavily industrialized Ohio Valley in the US, which falls mostly in Canada. Estimates suggest that approximately half of the sulphate falling in Canada originates in the US. These point sources of pollution are, however, easier to monitor and control than the other main source of acids—nitrogen emissions. The highest emissions come from various means of transport as a result of high-temperature combustion, followed closely by emissions from the oil and gas industry (Figure 5.17). Two main factors account for the decline in nitrogen emissions: a reduction in emissions from transportation, due to cleaner technology and fuels, and a reduction in emissions from electricity generation as a result of regulation and domestic and international agreements. FIGURE 5.17 Nitrogen oxide emissions and their sources, 1990–2015. Source: Environment and Climate Change Canada (2017). © Her Majesty the Queen in Right of Canada, as represented by the Minister of the Environment, 2020. Note: The indicator reports air pollutant emissions from human activities only. The category “other sources” includes emissions from ore and mineral industries, manufacturing, building heating and energy generation, home firewood burning, incineration and waste, agriculture (livestock, crop production, and fertilizer), dust and fires, paints and solvents, and other miscellaneous sources. What Are the Effects of Acid Deposition? Aquatic Effects The fate of the fish of Lumsden Lake and other aquatic ecosystems is one visible sign of some of the impacts of acid deposition. Other species are also affected as the pH of the water body declines. Indeed, as can be seen in Figure 5.18, fish are often not the most sensitive species and are really more of an indicator of the damage that has already occurred. As insects such as mayflies are eliminated, species higher in the food chain that feed on them become affected through food depletion. The same is true of fish-eating birds such as loons, whose young have a lower chance of survival on acidified lakes because of starvation. FIGURE 5.18 Sensitivity of various aquatic organisms to pH level. Source: Environment Canada (1991). Unfortunately, some of these impacts appear permanent. Examination of historical angling records in Nova Scotia, for example, indicates that of 60 main salmon rivers, 13 runs of salmon are extinct and a further 18 are virtually extinct. It is estimated that more than half of the total salmon stock has been lost as a result of declining pH levels. Atlantic salmon populations in rivers of the Southern Upland region of Nova Scotia continue to be negatively affected and will likely become extinct if adult survival rates remain at current low levels and pH recovery continues to be delayed. In northern Nova Scotia, where acid deposition is much less, salmon numbers have increased. Even where fish manage to survive, they may be grossly disfigured, with twisted backbones and flattened heads, because their bones have been deprived of necessary nutrients for strength. Reproductive capacities may be sufficiently impaired to lead to eventual population declines. Generally, the time of reproduction is the most sensitive part of the life cycle. The critical factor is often the lower pH level of the water as the snow melts. At this time, the buildup of acids over the winter can result in even higher acidity than experienced through the rest of the year. This pulse of acidity is called acid shock, and it may also be one of the causes of stress on amphibious creatures, such as frogs, that often use small temporary pools of water for breeding in the spring following runoff. At Lumsden Lake, for example, spring runoff produced a pH as low as 3.3, over 100 times more acidic than 1961 levels. A handful of the jelly-like Holopedium species that are becoming the dominant plankton in some lakes in eastern Canada as a result of calcium deficiencies caused by acid rain. More acidic water and the subsequent food chain effects are not the only concern. Other chemical changes occur. The increased acidity, for example, releases large amounts of aluminum from the terrestrial ecosystem into the rivers and lakes. Here it forms a toxic scum, lethal to many forms of aquatic life. The full implications of what some of these changes mean over the long term are only just coming to light. In eastern Canada, for example, acid rain has removed many nutrients from already nutrient-deficient catchments. Calcium is one such nutrient, and it turns out that a dominant species of the zooplankton and subsequent food chain, Daphnia, requires calcium in large supplies to be able to grow its protective exoskeleton. In the new calcium-deficient environment, it can no longer compete against another species, Holopedium, with lower calcium requirements, which is now displacing Daphnia. Unfortunately, Holopedium relies upon a slimy gel to repel predators, and some of Ontario’s lakes are becoming “jellified” as a result of the doubling in abundance of the species since the 1980s (Jeziorski et al., 2014). Not only does this provide an unpleasant swimming experience, but there are fears that the plankton will start to clog filtration systems on water supply intakes. In addition, Holopedium has relatively low nutrient content compared with Daphnia and therefore fewer nutrients will be passed along to the next trophic level. Terrestrial Effects Terrestrial effects of acid deposition first became visible around emission sources, such as at Trail, BC, and Sudbury, Ontario, as trees began to die. Before joining the soil, the acids eat away at the sensitive photosynthetic surfaces of the leaves. Broad-leaved trees, such as sugar maples, the source of Quebec’s $400-million annual maple syrup industry, are particularly susceptible because of the large surface area of their leaves. Once in the soil, the acids leach away the nutrients required for plant growth, leading to nutrient deficiencies. The high levels of aluminum released by the acids also inhibit the uptake of nutrients. The bacteria so critical to the workings of many biogeochemical cycles are also adversely affected and cause changes in natural soil processes. Decomposition and humus formation are retarded. Soil contains many organisms involved in the critical ecological functions of biogeochemical cycling and energy flow. There may be more than 100 million bacteria and kilometres of fungal hyphae in a single gram of healthy soil. Mycorrhizal fungi are very important for the growth of many plants because they help to transport nutrients from the soil water into the roots. Research on Jack pine, undertaken to understand the potential impact of increased acidity on these mutualistic relationships, found that changes in calcium-to-aluminum ratios caused by increased acidity influenced the succession of mycorrhizal fungi on tree root systems. The actual physical contact of the acids with the plant roots can also inhibit growth and lower resistance to disease. The long-term effects of these kinds of changes on tree growth, the ecological health of the community, and forest yields are still highly uncertain. Damage from acid deposition is now visible over much wider areas than those surrounding sources of high emissions. There are extensive areas of damage in Europe and the eastern United States. However, it is often difficult to single out one cause, such as acid deposition. It is likely that other factors—climate change and other pollutants, such as high levels of ozone brought about by excessive nitrogen oxides—are also important in placing stress on these communities in synergistic reactions. The smelter at Trail, BC, caused extensive damage to the surrounding vegetation. The impact on plant life is not restricted to natural ecosystems. Significant changes can also occur on agricultural lands as direct damage to crops or more indirect changes through changes to soil chemistry. The growth of crops such as beets, radishes, tomatoes, beans, and lettuce is inhibited, and biological nitrogen fixation is diminished at a pH of 4. In central and eastern Canada, 84 per cent of the most productive agricultural lands receive more than the 20 kilograms of acid deposition per hectare. In some areas, the application of lime to the soil has become a routine agricultural procedure in an attempt to neutralize the acids by adding more basic ions. Heterotrophs can also be affected. As the forest cover diminishes, so does the habitat for many species. Toxic metals such as cadmium, zinc, and mercury, released from the soil by the acids, may be concentrated by certain species of plants and lichens and accumulated in the livers of species eating them, such as moose and caribou. Loons, with their beautiful coloration and haunting cries, are a wilderness symbol for many Canadians, but their breeding success has been reduced by the effects of acid rain. Ecosystem Sensitivity Not all ecosystems are equally sensitive to the effects of acid deposition. The critical load is the maximum level of acid deposition that can be sustained in an area without compromising ecological integrity. Some areas have a high capacity to neutralize excess acids because of the high base capacity of the bedrock and soils. For example, the Prairies are less sensitive to the effects of acid deposition, because underlying carbonate-rich rocks, deep soils, and other factors combine to provide high buffering capacity. On the other hand, areas with difficult-to-weather rocks with low nutrient content (e.g., granite) and with thin soils following glaciation often have very low buffering capacities. Much of central and eastern Canada and coastal British Columbia fit within this category (Figure 5.19). The provinces most vulnerable to acid deposition in terms of amount of area ranked as highly sensitive are Quebec (82 per cent), Newfoundland and Labrador (56 per cent), and Nova Scotia (45 per cent). The spatial coincidence of low buffering capacities and high deposition rates explains why most attention in Canada has centred on the central and eastern parts of the country. FIGURE 5.19 Sensitivity of terrain to acidity. Critical load index, 2008; light green through red categories are considered acid-sensitive terrain. Source: Federal, Provincial, and Territorial Governments of Canada (2010: 68). © Her Majesty the Queen in Right of Canada, as represented by the Minister of the Environment, 2020. Socio-economic Effects The environmental effects described above have socio-economic implications, and there are also direct effects of acid deposition on human health. In aquatic ecosystems, for example, declines in fish populations have implications for those involved in fishing. The impacts of acid deposition on tree growth and the forest industry are also of considerable concern. Some studies indicate that tree growth reductions of up to 20 per cent are possible. Estimates of the market value of lost wood production resulting from acid rain are in the hundreds of millions of dollars in Nova Scotia and New Brunswick. The full effects, however, are likely to be much greater. Research indicates that a time lag of some 20 to 30 years is likely before the effects of acid rain are reflected in reduced tree growth. Many values, however, are difficult to express in monetary terms. Thousands of Canadians, for example, maintain lakeside cottages. It is challenging to ascribe a value to the changes that might occur as the lakes become devoid of life. Common loons, for example, are for many the quintessential symbol of the Canadian wilderness, yet populations are very sensitive to acidification. Below pH levels of 4.5, loons are unable to find enough food for their young. However, as a result of emissions regulations in Canada and the US, overall improvements in the capacity of many lakes to support aquatic biota are being observed. For instance, a general increase in the number of breeding fish-eating water birds (such as loons) has been observed in lakes in Ontario, Quebec, and Newfoundland, particularly those near reduced emission sources. At the same time, algae, invertebrates, and water-bird food chains in many lakes in this region continue to show acidification impacts (i.e., direct effects of acidification, metal toxicity, loss of prey species, and reduced nutritional value of remaining prey), particularly in lakes and rivers where fish communities have been affected. The human-built environment, as well as the natural environment, is damaged by acid deposition. The acids eat away at certain building materials. The effects have been most damaging in Europe, with its many old monuments, but such effects can also be seen on the Houses of Parliament and nearby statues in Ottawa. Estimates suggest that acid rain causes over $3 billion worth of damage to the human-built environment in Canada every year. The most direct impact on human health may arise from inhalation of airborne acidified particles, which can impair respiratory processes and lead to lung damage. There are significant relationships between air pollution levels in southwestern Ontario, for example, and hospital admissions for respiratory illnesses. Comparative studies between heavily polluted areas in Ontario and less polluted areas in Manitoba found diminished lung capacity in about 2 per cent of the children in the more polluted areas. Further studies have confirmed this relationship, although whether high sulphate or ozone levels are responsible has yet to be conclusively determined. Calculations by the US government suggest that southern Ontario has been saving more than $1 billion per year in health costs since 2010 as a result of efforts to reduce emissions in the US. Humans can also be affected by ingesting some of the products of acid rain. Regarding drinking water, for example, in some areas where older delivery systems are still in place, the increased acidity of water can corrode pipes and fittings and result in elevated levels of lead in the water supply. This is one reason why in certain areas, such as Victoria, BC, it is recommended that schools flush their water fountains early in the morning to spill the water that has been held overnight and that might show elevated lead levels. Acidified water may also hold other substances in solution that are deleterious to human health. Excess levels of aluminum have already been noted. When metals such as mercury, chromium, and nickel are leached from the substrate into water, they may be taken up and concentrated along the food chain and eventually cause a human health problem. What Can We Do about It? One of the main challenges associated with acid deposition is that it is not limited to areas generating the emissions and causing the problem. In Canada, more than half of the acid deposition originates in the US. To address such problems, international efforts are required, as is a greater concern on the part of individuals (see Box 5.11). Environment in Focus BOX 5.11 What You Can Do: Taking Individual Action Every Day Many challenges and problems discussed in this book are international in scope and require the coordinated efforts of different levels of government, industry, and individuals. Matter cycles are a relatively easy way for individuals to reduce their environmental impacts through the day-to-day decisions that we all make regarding food, water consumption, shopping, and many other activities. The following are some ways individuals can have a positive influence. 1.Recycle your wastes. In BC, just by recycling beverage containers, consumers contributed to the reduction of 135,000 tonnes of carbon dioxide equivalent in 2010. This is equivalent to taking 39,000 cars off the road for a year. 2.Acid deposition is profoundly influenced by the personal decisions we each make regarding use of fossil fuels in transport and electricity consumption. Think about your decisions and how you can minimize use. 3.Many consumer items, such as TVs, cellphones, computers, and other electronic products, contain materials such as lead and nickel that contain sulphur. As you buy and dispose of these items, you are helping to increase acid deposition. Only buy items that you really need and always dispose of them correctly. 4.Use chemical fertilizers sparingly on your gardens to reduce impacts of excessive nutrients on water bodies. 5.Eat less meat. This reduces the demand for livestock, and livestock are major contributors to eutrophication. 6.Let your political representatives know that you are in favour of mandatory measures to curb sulphur emissions and treat livestock wastes, even if this costs you more money. Canada has addressed the problem through national, bilateral, and multilateral efforts. In 1983, the Canadian Council of Resource and Environment Ministers agreed on an annual target deposition or critical load of 20 kilograms per hectare as an acceptable goal, taking political and economic costs into account. This is an important qualification. The critical load represents a policy target value (PTV) set by politicians. At the time, scientists warned that a further 75 per cent reduction in SO2 emissions would be needed to address the situation adequately. This is a scientific target value (STV), and subsequent experience demonstrates that the scientists were correct in their assessment. Many of the environmental problems you will read about in this text represent instances in which PTVs were established that conflicted with STVs. Total allowable catch in fisheries (Chapter 9) and annual allowable cut in forestry (Chapter 10), as well as designation of endangered species (Chapter 15), are good examples of this conflict. Advances in science have allowed more comprehensive assessments of critical loads to be defined that combine sulphates and nitrogen oxides (NOX) for both aquatic and terrestrial ecosystems. They are now expressed as ionic charge balance in terms of equivalent/hectare/year, and the old load of 20 kilograms/hectare/year is represented by 416 equivalent/hectare/year. Current critical loads for the Boreal Shield are shown in Figure 5.20, with between 21 and 75 per cent of eastern Canada still receiving deposits in excess of the critical load. The very wide range represents the uncertainty regarding long-term effects of nitrogen and the degree of future absorption in soils. FIGURE 5.20 Areas where the critical load has been exceeded in the Boreal Shield. Number of units above critical load, 2009. Source: Federal, Provincial, and Territorial Governments of Canada (2010: 68). © Her Majesty the Queen in Right of Canada, as represented by the Minister of the Environment, 2020. Between 1990 and 2015, sulphur oxide (SOX) emissions fell about 66 per cent in Canada (Environment and Climate Change Canada, 2017). However, in 2014, Canada still ranked fourth-highest in emissions among OECD member countries and had the fourth-highest ratio of SOX emissions to gross domestic product. Significant differences exist among the provinces (Figure 5.21) with Prince Edward Island having the lowest emissions per capita (although still significantly more than most European countries) and Manitoba the highest, and one of the highest in the world, driven by the ore smelting in that province. FIGURE 5.21 Sulphur oxide emissions, 2014. Source: Conference Board of Canada (2016b). Efforts elsewhere show that significant reductions can be made over relatively short periods. In 1990 both Germany and the UK had annual SOX emissions significantly in excess of Canada’s. However, 20 years later, both showed emissions well below Canada’s and below every province except PEI. Canada has also made substantial but inadequate reductions in NOX emissions per capita with reductions of 47 per cent between 1990 and 2014. Again, this is significantly poorer than most other peer countries and large differences exist among provinces (Figure 5.22). FIGURE 5.22 Nitrogen oxide emissions, 2014. Source: Conference Board of Canada (2016a). Mobile emission reductions are being sought by introducing more stringent performance standards on exhaust emissions from new vehicles. However, since 2011, Canada has synchronized regulations with the US, and the current political leadership in the US is looking to relax, not tighten, standards. In eastern Canada there have been reductions in NOX emissions, largely the result of coal-fired power plant closures. In the West, NOX emissions continue to increase, driven by the oil sands developments (Chapter 13). Alberta accounts for over one-third of national NOX emissions. Federal regulations require reductions costing some $300 million in emissions from industrial activities by 2029. Many feel that a 10-year adjustment phase is unnecessary and more urgent action is needed. However, some provinces are now challenging the right of the federal government to regulate in this manner—actions that emphasize the importance of the subtitle of this text, Change and Challenge. An analysis of the US Acid Rain Program estimated annual benefits of the program to both Canada and the United States at $122 billion in 2010 and costs for that year at $3 billion (in 2000 dollars)—a 40-to-1 benefit–cost ratio. These benefits flow from such factors as improved air quality prolonging lives, reducing heart attacks and other cardiovascular and respiratory problems, and improving visibility. Canada has met all its goals and commitments, some with considerable time to spare, in terms of acid deposition reductions. There has been a decreasing trend in lake sulphate levels in southeastern Canada in response to reductions in SO2 emissions, and this has resulted in improved pH levels. For example, Table 5.7 shows changes in pH levels over time in the two lakes that provide the drinking water for Halifax, Nova Scotia. However, these changes have led to increased algal activity and higher water treatment costs for Halifax (Anderson et al., 2016). TABLE 5.7 pH Levels in Lakes Supplying Halifax’s Drinking Water Days of pH below 5 2002 2010–2015 Lake Major 153 10 Pockwock Lake 162 7 Source: Anderson et al. (2016). Nevertheless, many lakes are still acidified, many at levels below pH 6, a key threshold for the sustenance of fish and other aquatic biota. Acid deposition remains a significant problem in Canada and will remain so for years to come. To some extent, the issue has been overshadowed by public and political interest in global climate change, but many causes for concern persist. Emission controls have focused largely on point-source control of sulphate emissions. It is more difficult to address the more diffuse nitrogen derivatives coming mainly from the transportation sector. The pattern of wet nitrate deposition has changed little over the last decade. Automobile emissions reduce the air quality in many urban areas. Concern has focused on eastern Canada, but pockets of acidity exist across the country. The presence of acid-sensitive geology and increasing emissions of SO2 and NOX from oil and gas developments suggest that monitoring should expand into the western provinces to assess acid deposition impacts on ecosystems in that regio