Unit 1 SG (1) PDF - Carbohydrate Structure
Document Details
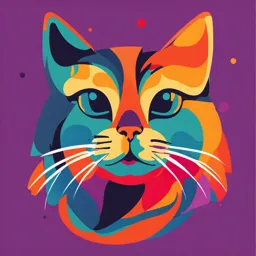
Uploaded by ContrastyFactorial
Clemson University
Tags
Summary
This document provides an overview of carbohydrate structure, including monosaccharides (e.g., D-glucose, D-fructose), disaccharides (e.g., lactose, sucrose), and oligosaccharides. It also mentions the difference between aldose and ketose sugars and various kinds of carbohydrates.
Full Transcript
Unit 1 - Carbohydrates Powerpoint #1 - Carbohydrate Structure Monosaccharides: D-Glyceraldehyde ○ Starting point for carbohydrate synthesis ○ Has one chiral carbon D-Glucose - Glcp ○ Aldose sugar ○ 4 chiral carbons ○ Main carbohydrate...
Unit 1 - Carbohydrates Powerpoint #1 - Carbohydrate Structure Monosaccharides: D-Glyceraldehyde ○ Starting point for carbohydrate synthesis ○ Has one chiral carbon D-Glucose - Glcp ○ Aldose sugar ○ 4 chiral carbons ○ Main carbohydrate we consume Glucose is 75% of the carbohydrates we consume Rice, grains, potatoes = glucose D-Galactose - Galp ○ Aldose sugar (6 carbons) ○ Found in dairy D-Erythrulose ○ Uncommon carbohydrate ○ One chiral carbon like glyceraldehyde D-Fructose - Fruf ○ 2nd most consumed carbohydrate ○ In sweeteners and added sugars like syrups ○ A ketose sugar ○ 3 chiral carbons Difference between glucose and fructose structure ○ Both glucose and fructose have 6 carbons but fructose has double bond to oxygen on 2nd carbon and glucose has double bond to oxygen on 1st carbon ○ When considering nutrients mostly consider the D form ○ In human metabolism we will digest the D version Aldose sugar vs ketose sugar in monosaccharides ○ Differ in location of carbonyl group ○ Aldose sugars (glucose, galactose) have double bond to oxygen at Carbon #1 whereas ketose sugars (fructose) have double bond to oxygen at Carbon #2 ○ Both mostly have 6 carbons ○ Powerpoint #2 - Carbohydrate Structure Other uncommon monosaccharides: Oxidized sugar derivatives ○ Uronic acids ○ Derivatives of other carbs ○ Not common in food Reduced sugar derivatives ○ Sugar alcohols Carbonyl is reduced to become an alcohol ○ Are mainly added to food products as reduced or no calorie sweeteners > ex: gum ○ Ex: D-xylitol D-Glucitol (D-Sorbitol) Disaccharides: Bonds between monosaccharides = glycosidic bonds Lactose ○ Galactose + glucose (monosaccharides) link to create the disaccharide, lactose ○ Beta 1 -4 bond Glycosidic bond that links the 2 disaccharides When glycosidic bond is up = beta formation Links 1st C of Galactose with 4th C of glucose ○ Found in dairy ○ Sucrose ○ Glucose + fructose ○ Alpha 1-2 glycosidic bond Bond pointing down = alpha 1,2 bc reversed Link 1st C of Glucose with 2nd C of Fructose ○ In table and added sugars ○ Maltose ○ Glucose + glucose ○ Alpha 1,4 bond Bond pointing down Link 1st C of glucose to 4th C of glucose ○ Product of digestion ○ Trehalose ○ Glucose + glucose ○ Alpha 1,1 bond Reversed ○ Found in mushrooms ○ Oligosaccharides (longer chain): Fructooligosaccharides ○ Non-digestible carbohydrates ○ Contain at least 3 monosaccharides and at least one fructose Raffinose Galactose > Glucose > Fructose Galactose linked to Glucose in alpha 1,6 bond Glucose linked to Fructose in alpha 1,2 bond Oligofructose Contains 3 fructose (only has fructose) 1st fructose linked to 2nd fructose via beta 2,1 bond 2nd fructose linked to 3rd fructose via beta 2,1 bond Starches (long polymers of glucose): Where most of our carbs come from ○ Rice, wheat, pasta, potatoes Plants store starches in long glucose molecules Two forms of starches: ○ Amylose Long polymer of glucose linked in alpha 1,4 bonds Glc-Glc-Glc-Glc ○ Amylopectin Has two types of glycosidic bonds Has beta 1,4 and beta 1,6 Alpha 1,4 bond holds glucose together Alpha 1,6 bond holds branches together Is different from amylose because it has a branch point (where you have the alpha 1,6 bond) When chains connect, increase compactness and have better storage Only one enzyme can break down alpha 1,6 bond unlike the alpha 1,4 bond ○ Glycogen What we store Store glycogen as a long polymer of glucose Structure is similar to amylopectin - has alpha 1,4 and alpha 1,6 bonds Powerpoint #2 - Digestion and Absorption Organ Overview: 1. Mouth a. Mechanical digestion via chewing 2. Salivary glands in the mouth a. Saliva has the enzyme salivary amylase to lubricates the esophagus 3. Parotid gland 4. Esophagus 5. Liver a. Makes bile 6. Gallbladder a. Stores bile 7. Stomach a. Have hydrochloric acid (HCl) acid to denature proteins so digestive enzymes can break apart proteins b. Also have digestive enzymes such as protease and gastric lipase 8. Pancreas a. Make most of our digestive enzymes like amylase 9. Small intestine - most digestion happens here a. Duodenum Proximal portion of SI Chyme comes in from stomach at a low pH and bicarbonate neutralizes it b. Jejunum Where most absorption occurs, do have some digestion continuing c. Ileum Mostly wrapped up digestion and absorption 10. Ascending colon a. Gut microbes interact with unabsorbed food - specifically fibers b. Water is mostly absorbed here 11. Anus Regions of SI 1. Lumen a. Hollow core of gastrointestinal tract b. Where digestive enzymes and bile mix before entering the SI 2. Epithelium a. Responsible for the uptake of nutrients into the SI b. Have digestive enzymes and transporters that move food from lumen into SI and also from SI into circulatory system 3. Lamina propria 4. Muscularis mucosa a. As the muscle layers contract, they do two things: i. Mix food with chyme and bile to allow for absorption into intestine 1. Mixes with digestive enzymes ii. Moves food from proximal to distal portion of SI and then LI or systems 1. Aids in peristalsis 5. Submucosa 6. Muscularis externa 7. Circular muscle 8. Longitudinal muscle 9. Serosa or adventitia The Stomach: - Limited absorption here, mostly focus on digestion 1. Esophagus 2. Gastroesophageal opening 3. Cardiac sphincter a. Regulates food from esophagus to stomach b. Also makes sure food doesn’t move backwards 4. Fundus 5. Body 6. Stomach has 3 muscle layers a. Muscle layers mix and move food through stomach b. Chewed food is mixed with HCl and digestive enzymes in the stomach 1. Longitudinal muscle layer 2. Circular muscle layer 3. Oblique muscle layer 7. Submucosa 8. Mucosa 9. Rugae 10. Pyloric antrum 11. Pyloric sphincter a. Regulates food aka chyme from stomach > SI 12. Duodenum Microscopic view of stomach - from lumen of stomach to epithelium to layers: Epithelial cells line pit of the stomach (gastric pits) Epithelial lining produces protective mucus and HCl in stomach ○ At the bottom of the gastric pits is where the line produces the mucus, HCl, and digestive enzymes to help move food into the stomach Have lymphatic vessels, venules, and arterioles to provide blood supply if there is any absorption 3 Regions of the Small Intestine: 1. Duodenum a. Where common pancreatic duct brings secretions from gallbladder and pancreas b. Neutralize contents from stomach to a more neutral pH and begin digestive processes from gallbladder 2. Jejunum a. Longest section of the SI b. Where most digestion and absorption occurs 3. Ileum a. Terminal portion of SI b. Low absorption of c. Connects to LI Microscopic view of SI - starting at lumen: Villi Epithelium ○ Enterocyte Each square that lines the villi is an enterocyte The epithelial cells that line the lumen of the SI are called enterocyte (only called that in intestines) Production The enterocytes are produced at the bottom of the villi aka the crypt and move upwards of the villi Epithelial cells are the peak of the villi are mature Produce digestive/pancreatic enzymes and transporters that function at the surface of the enterocyte to bring nutrients from lumen, across enterocyte and into SI Also have transporters to bring from lumen, into SI and then into circulation Crypt ○ Bottom of the villi ○ Basal portion where enterocytes are made 2 important vessels ○ Vessels project into villi and collect water soluble and fat soluble nutrients ○ Arteriole Hepatic portal vein Circulatory system Vessel that carries oxygenated blood to intestine and carries blood with water soluble nutrients to the liver ○ Lymphatic lacteal Central lacteal Part of the lymphatic system Carries lipid soluble nutrients aka triglycerides and fatty acids Bypasses the liver so we get lipids to the adipose and muscle cells to use or store as energy asap 2 layers of muscle in SI that move food along intestine ○ Circular muscle ○ Longitudinal muscle One enterocyte view: Enterocyte is a polarized cell ○ Has 2 different sides with 2 different functions - aka 2 membranes Apical membrane Responsible for uptake into SI Basolateral membrane Responsible for secreting nutrients into one of 2 systems (circulatory or lymphatic) Faces vessels such as arteriole (hepatic portal vein) or lacteal (central lacteal) Microvilli ○ Has microvilli which are specific to enterocytes ○ Microscopic hairlike extensions ○ Is called the brush border ○ Projections from plasma membrane Plasma membrane is continuous to form microvilli projections ○ Villi and microvilli allow for increased surface area and therefore more efficient at absorbing nutrients ○ Digestive enzymes and nutrient transporters produced by enterocyte are anchored to the brush border ○ Brush border creates a layer of unstirred water that acts as a barrier to everything outside of the body Water soluble nutrients move through easier than lipids or pathogens Pathogens typically have lipid based plasma membranes Lipids use bile to get through water layer Apical membrane ○ Portion of plasma membrane exposed to lumen ○ Is essentially the microvilli ○ The part above the tight junction Tight junction ○ A barrier between the plasma membranes of two touching cells Powerpoint #4 - Digestion & Absorption Accessory Organs: 1. Pancreas a. Exocrine function - Secretes 2 things into the small intestine: i. Bicarbonate 1. Neutralizes the low pH chyme from the stomach ii. Pancreatic digestive enzymes specific to the nutrient we are trying to break down (ex: fats, proteins, carbs) 1. Pancreatic enzymes function in the lumen of the small intestine UNLIKE the digestive enzymes produced by enterocytes which work at the surface of enterocytes aka the brush border b. The secretions from the pancreas flow through the pancreatic duct > common pancreatic duct > then into the SI c. Pancreas also has an endocrine function i. If you consume too much glucose… 1. Pancreas secretes insulin to decrease blood glucose levels ii. If you consume too little glucose… 1. Pancreas produces glucagon 2. Gallbladder a. Common bile duct i. Collects bile from gallbladder and/or liver ii. Pancreatic duct merges w common bile duct to form common pancreatic duct b. Collects bile from liver 3. Liver a. More optional but important if gallbladder is removed b. Liver secretes bile into the small intestine c. Bile components: i. Bile salts/acids ii. Cholesterol iii. Phospholipids d. Bile emulsifies dietary lipids to make micel so lipids can interact with digestive enzymes - Duodenum gets the secretions from the gallbladder and pancreas via common pancreatic duct - Common bile duct and pancreatic duct combine to form common pancreatic duct - Bile can come from gallbladder and/or liver - Common pancreatic duct mixes pancreatic juices, bile, and chyme Transport Processes: - Passive = does not require energy 1. Unmediated passive diffusion - Unmediated = do not use a transporter - Nutrients freely cross plasma membrane or go between cells - Most common with micronutrients a. Passive transcellular diffusion through lipid membrane i. Transcellular = going through cell ii. Goes through cell via apical lipid membrane and eventually out of enterocyte into circulatory system iii. Goes from higher concentration gradient in lumen to lower concentration in enterocyte b. Passive transcellular diffusion through aqueous pores i. Some small molecules can move through pores ii. Move from the higher concentration in the lumen of intestine through holes of the enterocyte into the enterocyte where there is a lower concentration c. Passive paracellular diffusion through paracellular pores i. Small molecules move through the space between 2 cells aka enterocytes ii. Tight junctions allow for cells to bind together but somme small molecules can get between cells 2. Carrier/receptor-mediated movement - Unlike unmediated, requires a protein to aid in movement across plasma membrane - There is one mediated passive process - does not require energy but requires a protein transporter a. Passive carrier-mediated uptake aka facilitated diffusion i. Is the same as pt. A (passive transcellular diffusion through lipid membrane) except that this one has a protein transporter ii. Nutrient is moving from high concentration in lumen to lower concentration in enterocyte but uses a transporter to move through plasma membrane iii. Because the nutrient is already moving in the right direction, does NOT use energy b. Active carrier-mediated transport i. Uses energy to move nutrients across plasma membrane ii. Primary 1. Involves direct input of energy such as ATP hydrolysis 2. Using that ATP with the transporter itself iii. Secondary 1. Involves symport or antiport systems that transport a nutrient against the gradient by using a separate primary active transport system a. Has a primary and secondary transporter i. Ex: SGLT binds to the nutrient (such as glucose) and also binds to sodium 1. Movement of glucose dependent on sodium concentration - moves from high conc of sodium in lumen SI to lower sodium conc in enterocyte ii. Same ex cont: sodium potassium (ATPase) pump is the secondary transporter 1. Moves sodium out of cell and potassium out at the expense of ATP 2. Is not directly transporting the nutrient, glucose 2. Is the most common way to take up carbs because it can move nutrients from lower to higher (against the gradient) which allows for the uptake of more nutrients - more efficient a. Can take up glucose even when there is a lower concentration in the small intestine and a high concentration in the enterocyte 3. Symport a. 2 moving together 4. Antiport a. 2 moving across plasma membrane in opposite directions c. Vesicular transport - such as endocytosis i. For very large molecules Large Intestine overview: Large intestine mostly focuses on GI bacteria that ferment leftover, non- absorbable food (such as fiber) to produce short FA chains that we can use for small amounts of energy or as signaling molecules Take up water to concentrate feces Both small intestine and large intestine have villi but LI has no microvilli Both have lymphatic vessels and muscle layers - both still have lymphatic sys and circulatory system Starch digestion (mouth to large intestine aka colon): - Diet consists of carbs, fats, proteins/amino acids - Specifically talking about starch digestion here 1. Mouth a. Begins digesting starches by mechanical digestion via chewing and by chemical digestion via using one digestive enzyme = salivary amylase in saliva i. Breaks alpha 1,4 bond b. Starch breaks down into (products of digestion): i. Scratch dextrins 1. Short polymers of glucose ii. Maltose 1. Disaccharide of glucose 2. Stomach a. No digestion of starches/carbs in stomach because low pH denatures salivary amylase b. Do begin digestion of proteins and fats i. HCl denatures proteins by removing some of the structure of protein so it can be further broken down by chemical enzymes ii. Have 2 digestive enzymes 1. Proteases a. Digestive enzymes that break down proteins 2. Lipases a. Digestive enzymes that break down fats 3. Small intestine a. Majority of digestion occurs here b. Breakdown via enzymes in SI i. Pancreatic amylase 1. Comes from pancreas into the small intestine where it breaks down starch into >> starch dextrins, maltotriose, and maltose 2. Also specific to alpha glycosidic 1,4 bond that’s in carbs so breaks that down a. Breaks down any 1,4 bonds left over after salivary amylase 3. Pancreatic amylase and salivary amylase are specific to that bond which is in carbs but in addition to pancreatic amylases there are also lipases and proteases that can break down fats and proteins - all of which come from the pancreas 4. All pancreatic enzymes function in lumen of SI ii. Sucrase-isomaltase and malatase glucoamylase 1. Breaks down starch dextrins, maltotriose and maltose into glucose iii. Surcase 1. Breaks down sucrose into glucose + fructose iv. Lactase 1. Breaks down lactose into glucose + galactose v. Trehalose 1. Breaks down trehalose into glucose + glucose c. Transportation of monosaccharides i. From lumen of SI into enterocyte (apical side) 1. SGLT1 a. Transports glucose and galactose from lumen of SI into the enterocytes via apical side of membrane 2. GLUT5 a. Transports fructose from lumen of SI into the enterocytes via apical side of membrane 3. SGLT1 and GLUT5 both use carrier-mediated uptake a. SGLT1 uses secondary active transport b. GLUT5 uses carrier mediated diffusion (no energy investment) ii. From enterocytes into portal blood plasma (basal side) 1. GLUT2 a. Single transporter on basolateral side b. Is non-specific c. Transports glucose, galactose, fructose from enterocyte into interstitial space via basal side of plasma membrane and then into hepatic portal vein and then into liver d. Carrier-mediated diffusion (no energy investment) e. Hepatic portal vein aka circulatory system is a vessel that goes directly from SI to liver i. One of the vessels that transports nutrients from intestine to a place in the body iii. Galactose, glucose, and fructose are the most absorbed 4. Colon a. Bacterial enzymes break down undigested food (aka fiber) into short chain FA via fermentation i. Non-digested carbs will either go through fermentation or be exerted via feces b. 3 short chain FA aka the 3 products from fermentation: i. Butyrate 1. 4 carbons long ii. Propionate 1. 3 carbons long iii. Acetate 1. 2 carbons long c. Take up short chain FA into blood to provide some energy or can act as signaling molecules Powerpoint #5 - Carb Digestion & Absorption Amylase structure and function: Pancreatic or salivary amylase can recognize starch ○ Pancreatic is functioning in lumen of intestine Amylase is specific to alpha 1,4 bond ○ Breaks the 1,4 bonds linking glucose molecules of starch Rectangular box = active site ○ This is where we are cleaving an alpha 1,4 bond ○ Amylase has to recognize at least 5 glucose molecules linked together Cleaves one alpha 1,4 bond Once it is shorter than 5 glucose residues long, use disaccharide-ases which are produced by enterocytes at wall of intestine Amylose is broken into maltotriose and maltose by amylase ○ The 2 end products of amylose digestion by amylase Amylopectin breakdown: Amylase only breaks down alpha 1,4 Amylase breaks the alpha 1,4 bond in amylopectin to create 3 products: ○ 2 products: maltotriose and maltose However not all of amylopectin is broken down into those 2 because the alpha 1,6 bond is still intact ○ Limit dextrins The remaining part of amylopectin with the alpha 1,6 bond intact Amylose and amylopectin breakdown: *still in lumen of SI* via amylase Amylose >>>>>>>>> maltotriose + maltose (2 products) via amylase Amylopectin >>>>>>>>> maltose + maltose and limit dextrins with alpha 1,6 bond still intact (3 products) Continuing breakdown of limit dextrins from amylopectin: *using the brush border enzymes, called the disaccharide-ases* - Glucoamylase or maltase - Isomaltase - Sucrase or maltase - These enzymes cut off one glucose at a time but amylase takes a large starch molecule and makes it much smaller Limit dextrins contains both alpha 1,4 and alpha 1,6 bonds ○ Pancreatic amylase breaks down alpha 1,4 but stops near 1,6 so usually leaves behind a few alpha 1,4 Glucoamylase or maltase breaks alpha 1,4 bonds left on limit dextrins ○ One glucose falls off each time cleaving bond Isomaltase breaks alpha 1,6 glycosidic bond (specific) ○ Left with maltotriose (3 glucose molecule) After isomaltase, maltotriose is broken down further into glucose by maltase ○ Broken down by enzyme twice *Can only absorb monosaccharides* - Intact starch molecule > salivary amylase in mouth > pancreatic amylase in lumen of SI > disaccharide-ases in brush border (glucoamylase or maltase > isomaltase > maltase) > glucose Disaccharide-ases: All function in brush border Maltase ○ Breaks alpha 1,4 bond Glucoamylase ○ Breaks the alpha 1,4 bond Sucrase ○ Breaks alpha 1,2 bond ○ Breaks sucrose (glucose + fructose) Isomaltase ○ Generally only get limit dextrins from starch digestion ○ Breaks alpha 1,6 bond Lactase ○ Digests lactose (glucose + galactose) ○ Breaks beta 1,4 bond After these enzymes break them down into monosaccharide in brush border, can be moved into enterocyte Carbohydrate uptake: Carrier-mediated transport mechanisms ○ Uptake of fructose into enterocyte GLUT5 transporter is specific to fructose (passive diffusion) Moves fructose into enterocyte via passive diffusion (higher conc of fructose in lumen of intestine to lower conc in enterocyte) ○ Uptake of glucose or galactose into enterocyte Uses SGLT1 as transporter (secondary active) Sodium-dependent glucose transporter First binds sodium since it is sodium dependent Then binds glucose or galactose SGLT1 functions based on conc gradient of sodium so as long as gradient is maintained (higher conc of sodium in lumen of SI and lower conc in enterocyte of SI), take up sodium and glucose or sodium and galactose at the same time ○ Called cotransport mechanism (2 nutrients at same time with same transporter) Using sodium-potassium ATPase to maintain sodium concentration (is the secondary transporter) ○ Moves sodium out of enterocyte into interstitial space and potassium in ○ At expense of ATP ○ Reduces sodium conc in enterocyte to maintain gradient between lumen of SI and enterocyte which drives SGLT1 ○ Taking monosaccharides out of enterocyte via transporter GLUT2 GLUT2 is passive Moves from high to low conc of nutrients Nonspecific transporter - transports all carb monosaccharides Transported across basolateral membrane into interstitial space and then into hepatic portal vein (carries water-soluble nutrients from intestine to liver) Insulin w carbohydrate consumption: As we consume carbs containing glucose, they are digested into glucose and then absorbed by entering bloodstream in which plasma glucose concentration increases ○ Pancreas senses that and secretes insulin to lower blood glucose conc As blood glucose increases so does insulin conc As blood glucose decreases so does insulin conc Takes about an hour after a meal for blood glucose conc to peak and very similar for insulin conc Takes about 2-3 hours to return baseline Digestion of all nutrients generally takes about 4 hours Fiber Metabolism Do not have enzymes to break down fiber so it’s a non-digested carb Done by bacteria in small and large intestine First is hydrolysis ○ Many fibers have beta 1,4 bonds and we lack that enzyme but our GI bacteria have an enzyme to break apart fiber into individual glucose molecules via hydrolysis After GI bacteria break down fibers into glucose, use that glucose in fermentation to produce energy ○ Ferment glucose ○ Byproduct of that fermentation is the short chain FA: butyrate, propionate, acetate ○ We can absorb short chain FA in large intestine and once taken up, we can get a small amount of energy Fibers are essentially non-digestible by human enzymes - must be digested by GI bacteria GI bacteria use most of the energy in a glucose molecule of fiber and we take up the rest as a short chain FA to produce a small amount of energy or to use as signaling molecules to decrease or increase metabolic pathways Powerpoint #6 - Carbohydrate Metabolism Intro to Carb Metabolism - Anabolic and Catabolic pathways: Mostly talking about catabolic pathways Catabolism ○ Sources of energy: Main sources are carbohydrates and lipids Only use proteins when necessary/as a last resort for energy ○ End products of using carbohydrates and lipids for energy by catabolism = CO2 + H2O ○ End products of using proteins (last resort) for energy by catabolism = urea OR NH4+ Anabolism ○ Simple building blocks for building large molecules aka nutrients Glucose Amino acids Acetyl-CoA Energy Release by Hydrolysis of ATP: Energy within phosphoanhydride bonds of ATP ATP has 2 phosphoanhydride bonds (high energy bonds) ○ Invest a lot of energy into making these bonds and capture a lot of energy when breaking these bonds 2 mechanisms to hydrolyze/break bonds in ATP 1. ATP > ADP > AMP a. 1st step: ATP + H2O >> ADP + Pi (inorganic phosphate) i. Remove first terminal phosphoanhydride bond b. 2nd step: ADP + H2O >> AMP + Pi i. Remove next phosphoanhydride bond from ADP just generated ii. Produce a 2nd inorganic phosphate 2. Straight from ATP > AMP a. Less common b. 1st step: ATP + H2O >> AMP + PPi (inorganic diphosphate aka pyrophosphate) c. 2nd step: PPi + H2O >> 2 Pi i. Splitting apart pyrophosphate into 2 molecules of inorganic phosphate d. Generates more energy kcal/mol than the 1st mechanism e. Why would we not use it more? i. 1st mechanism requires less energy to invest to reforming building black ii. More enzymes can do 1st mechanism (converting ATP to ADP) Metabolism catabolic overview Nutrients (highlighted): A. Protein metabolism a. Amino acid and carbon skeletons (everything in an amino acid with the nitrogen part removed) b. 3 options: i. Can be turned into pyruvate ii. Can be turned into directly acetyl-CoA iii. Can go into directly TCA cycle B. Carbohydrate metabolism a. Glucose or glucose 1-phosphate from glycogenolysis or lactate from anaerobic metabolism b. 1st step in metabolizing carbs: i. Go through glycolysis to produce pyruvate ii. Main metabolic pathway for glucose is glycolysis c. From pyruvate, have 2 options: i. Aerobic metabolism 1. Pyruvate will go from cytoplasm into mitochondria to continue on through TCA cycle and ETC ii. Anaerobic metabolism 1. Convert pyruvate to lactate iii. Choice to use pyruvate for aerobic vs anaerobic depends on the amount of energy we need and how fast we need the energy 1. If need a small amount of energy v quickly, use anaerobic metabolism 2. If have more time, can use aerobic metabolism (can produce more energy but takes more time) C. Fat metabolism (our other main energy nutrient) a. Glycerol and Fatty acids i. 2 molecules because we consume fats as triglycerides which have a glycerol backbone with 3 FA b. Specific to FA portion: i. FA turned into Acetyl-CoA by beta oxidation ii. Acetyl-CoA goes into TCA cycle All macronutrients eventually funnel into TCA cycle ○ TCA cycle is the common catabolic pathway for all nutrients Fatty acid, glucose, and amino acid metabolism: Glucose 1. Glucose >> 2 molecules of pyruvate by glycolysis a. 2 ATP used, 4 ATP produced so net ATP produced = 2 ATP b. 2 NADH also produced 2. 2 molecules of pyruvate >>>> 2 molecules of acetyl-CoA by pyruvate dehydrogenase complex a. Pyruvate dehydrogenase complex is the enzyme that converts pyruvate to acetyl-CoA (the molecule that enters TCA cycle) b. 2 NADH produced 3. Acetyl-CoA into TCA cycle a. Starting molecule of cycle: citrate b. Ending molecule of cycle: oxaloacetate c. After TCA cycle, get 6 NADH, 2 FADH2 and then using substrate level phosphorylation get 2 ATP i. Substrate level phosphorylation = enzyme directly phosphorylates ADP to ATP 4. Catabolic pathway of metabolizing carbohydrates produced 4 ATP and reducing equivalents (10 NADH and 2 FADH2) 5. Electron transport chain a. Utilize reducing equivalents (NADH or FADH2) b. Energy carried within equivalents, energy is donated to chain, and the mitochondria can then produce energy c. ETC leads to oxidative phosphorylation i. Oxidative phosphorylation = combine ETC and ATP synthase while using oxygen as terminal electron donor ii. Forming ATP aerobically Fatty acid 1. A fatty acid is converted into acetyl-CoA by beta oxidation During beta oxidation, produces many reducing equivalents so generate about half of the energy from FA through beta oxidation and the other half through the TCA cycle 2. Acetyl-CoA > TCA cycle a. Produces 6 NADH, 2 FADH2, and 2 ATP by substrate level phosphorylation per 2 pyruvate Amino acid (via transamination, deamination, proteolysis) 1. Break down amino acid in either: a. Pyruvate b. Acetyl-CoA c. A TCA cycle intermediate 2. Proceed through TCA cycle a. Produces 6 NADH, 2 FADH2, and 2 ATP by substrate level phosphorylation Most of our energy is made from ox phosphorylation and using oxygen - a small part is made anaerobically and with pyruvate Nutrient metabolism in the mitochondria: Glucose > pyruvate (glycolysis) occurs in cytoplasm Protein > amino acid metabolism occurs in cytoplasm Beta oxidation of FA occurs in mitochondria Mitochondria has 2 membranes (this makes the mitochondria semi- permeable): ○ Outer membrane ○ Inner membrane Is wavy so you have more surface area to have more proteins (enzymes) embedded in the inner membrane for the TCA cycle TCA cycle is near to ETC - helps efficiency Most of TCA cycle products are reducing equivalents for ETC Powerpoint #7 - Carbohydrate Metabolism pt 2 Pyruvate Dehydrogenase Complex: 3 enzymes that make up the complex: ○ E1, E2, E3 4 B Vitamins in complex ○ ThDP ○ Pantothenic acid - used to make coenzyme A ○ Riboflavin - used to make FAD ○ Niacin - used to make NAD Pyruvate dehydrogenase complex is the enzyme that converts pyruvate (substrate) into acetyl CoA (product) in 4 steps: 1. Step 1 a. Uses enzyme e1 b. Start with pyruvate (3 carbon molecule) c. By using E1, go through oxidative decarboxylation (removing a carbon dioxide, CO2) d. After CO2 is removed, the remaining part of pyruvate (2 carbon molecule) is picked up by ThDP (Thiamine diphosphate) e. ThDP acts a carrier for the 2 carbon molecule and transfers the 2 carbons next to lipoic acid (5 membrane ring group) f. 2. Step 2 a. Uses enzyme e2 b. Lipoic acid goes off to the right and 2 carbon molecule links up with coenzyme A (CoA) to form acetyl-CoA c. Reduce lipoic acid by adding 2 hydrogens (adds more energy) 3. Step 3 a. E2? And E3? b. Oxidize lipoic acid and reduce FAD to generate FADH2 i. 2 hydrogens from lipoic go to FAD 4. Step 4 a. Oxidize FADH2 (take off the 2 hydrogens aka protons and donate them to reduce NAD to NADH + H) i. NADH & H go onto ETC - E1 - Oxidative decarboxylation - E1 causes ThDP to pick 2 carbon molecule - E2 - Transfers 2 carbon group to lipoic acid - And esterifies acetyl group to coenzyme A - Lipoic acid reduces when acetyl group and coenzyme a come together - E3 TCA Cycle: Start with acetyl-CoA (entrypoint into TCA cycle 1st important step ○ Acetyl CoA joins with oxaloacetate to form citrate by using the enzyme citrate synthase ○ Substrate acetyl-CoA oxaloacetate ○ Product Citrate ○ Enzyme Citrate synthase 2nd important step ○ Substrates Isocitrate NAD+ ○ Products Alpha-ketoglutarate NADH & H+ ○ Enzyme Isocitrate dehydrogenase Isocitrate is converted to to alpha-ketoglutarate by isocitrate dehydrogenase (enzyme) ○ Also have NAD as a substrate which is reduced to form NADH & H+ (reduced form) NADH and H+ go onto ETC ○ Anytime you hear dehydrogenase = reducing equivalent NADH, FADH, NADPH 3rd important step - alpha ketoglutarate complex ○ Uses 3 different proteins in complex ○ Substrates Alpha-ketoglutarate NAD+ Reduced CoASH (Coenzyme A) ○ Products Succinyl-CoA NADH & H+ Goes onto ETC CO2 ○ Enzyme Alpha-ketoglutarate dehydrogenase complex 4th important step ○ Substrate Succinyl-CoA ○ Product Succinate ○ Enzyme Succinyl-CoA synthetase ○ One step where we make ATP via substrate-level phosphorylation 1 ADP > 1 ATP 5th important step ○ Substrate Succinate FAD Reduced ○ Product Fumarate FADH2 Goes onto ETC ○ Enzyme Succinate dehydrogenase 6th important step ○ Substrate Malate NAD+ ○ Product Oxaloacetate In order for TCA cycle to start, need oxaloacetate Regenerate it here NADH + H+ Enters ETC ○ Enzyme Malate dehydrogenase Products of 1 round of TCA cycle per one acetyl-CoA ○ 3 NADH ○ 1 FADH2 ○ 1 ATP ○ 2 CO2 Electron transport chain complexes: Take energy from reducing equivalents to power the ETC so it can provide energy to phosphorylate ADP to ATP Focusing on NADH in ETC: Complex 1 protein ○ This is where NADH can donates its protons and electrons ○ Protein allows for acceptance of electrons from NADH and H+ These are oxidized to produce NAD+ ○ Electrons flow through the rest of the proteins of ETC ○ 4 protons are moved from the matrix of the mitochondria to intermembrane space Intermembrane space = space between inner and outer mitochondrial membranes When protons are moved, create a gradient because have increased concentration of protons in intermembrane space Coenzyme Q ○ Intermediate between complexes of ETC ○ Coenzyme Q donates electrons from complex 1 to complex 3 Complex 3 ○ 4 protons moved into intermembrane space Cytochrome C ○ Intermediate between complexes of ETC ○ Transports the 8 total electrons (4 from complex 1, 4 from complex 3) to complex 4 Complex 4 ○ 2 protons moved into intermembrane space End with water Can move a total of 10 protons into intermembrane space per one NADH through the ETC Focusing on FAD in ETC: Complex 2 ○ Succinate dehydrogenase is in complex 2 converting succinate to fumarate While succinate dehydrogenase converts succinate to fumarate in the TCA cycle, it also produces FADH2 which goes into the ETC TCA cycle enzymes embed in intermitochondrial membrane - embed at succinate dehydrogenase or complex 2 The FADH2 which comes into the ETC at complex 2 then donates its electrons which move through coenzyme Q > complex 3 > cytochrome C > complex 4 > water At complex 3 we move 4 protons from the matrix into intermembrane space At complex 4, we move 2 protons into intermembrane space Can move 6 protons into intermembrane space from matrix with one FADH2 going through ETC ATP Synthase protein (enzyme): Protons in intermembrane space funnel down to ATP synthase ATP synthase has a hollow core which the protons flow through to move out from intermembrane space and into the matrix As protons come through ATP synthase, this provides energy for ATP synthase ○ Provides enough energy so ATP synthase can phosphorylate ADP and the inorganic phosphate to make ATP (ADP + Pi > ATP) ○ Happens in the matrix Math of ATP synthase ○ For roughly every 4 protons that move through ATP synthase, can phosphorylate one ADP into one ATP ○ If one NADH can move 10 protons through, get roughly 2.5 ATP per NADH 10/4=2.5 ○ If one FADH2 can move 6 protons through, get roughly 1.5 ATP per FADH2 6/4 = 1.5 ○ NADH produces slightly more energy in ETC Notes: Will not always be producing both NADH and FADH2 Protons in the intermembrane space are recycled between matrix and space NADH and FADH2 are produced during TCA cycle but begin interacting with ETC at complex 1 for NADH and complex 2 for FADH2 Shuttling systems for reducing equivalents: Transport from cytoplasm to mitochondria to be used in ETC This is how we get reducing equivalents made from glycolysis in the cytosol into the mitochondria for the ETC Bc glycolysis happens in cytosol need to get them in mitochondria unlike the NADH made in the TCA Malate-aspartate shuttle ○ Start and end with malate ○ Malate and alpha ketoglutarate move in opposite directions Antiport system 1. Oxaloacetate in the cytosol is reduced to malate and NADH is oxidized to NAD+ 2. Malate carries the electrons from NADH with it through the intermitochondrial membrane and into the matrix a. As malate goes into the mitochondria, alpha ketoglutarate moves out of the mitochondria and into the cytosol i. Antiport system b. Now the NADH can go into ETC 3. Malate is then oxidized to oxaloacetate and an NAD+ already in the matrix is reduced using the electrons carried over 4. Oxaloacetate is then converted to aspartate and aspartate moves across the membrane and into the intermembrane space/cytosol a. As aspartate moves out of the mitochondria, glutamate moves in i. Antiport system 5. Aspartate is converted back to oxaloacetate and the process repeats Glycerol-3-phosphate shuttle 1. Start off with dihydroxyacetone phosphate and NADH 2. Dihydroxyacetone phosphate picks up NADH and therefore dihydroxyacetone phosphate is converted to glycerol-3-phosphate and NADH is oxidized to NAD+ 3. Glycerol-3-phosphate carries electrons from NADH while coming from cytosol 4. At inner mitochondrial membrane, glycerol-3-phosphate donates the electrons and protons to the FAD 5. FAD is reduced to FADH2 6. FADH2 then transfers electrons and protons to coenzyme Q 7. When glycerol-3-phosphate donates the electrons it was carrying, regenerates dihydroxyacetone phosphate to For the malate-aspartate, start and end with NADH ○ Start with 2.5 and end with 2.5 For the glycerol-3-phosphate shuttle, take a NADH and turn it into a FADH2 ○ Lose a slight amount of energy ○ Start with 2.5 in cytoplasm, end with 1.5 in mitochondria Cataplerosis: Leaving the TCA cycle Removing an intermediate in the TCA cycle for another pathway Can also pull intermediates out of glycolytic pathway Anaplerosis: Regenerating intermediates of the TCA cycle Can also regenerate and add intermediates back in for the glycolytic pathway Powerpoint #8 - Carbohydrate Metabolism pt 3 Fed state of carbohydrate metabolism: 1. Consume dietary carbohydrate 2. Digestion in mouth and SI 3. Absorption of glucose and other monosaccharides (fructose, galactose) in SI 4. Glucose, galactose, and fructose absorbed via hepatic portal vein 5. Liver a. Energy Generation ATP - Liver will first make sure it has enough energy by doing: i. Glycolysis 1. Glycolysis = breakdown of glucose to pyruvate ii. Oxidation of pyruvate to CO2 + H2O 1. Converting pyruvate to acetyl CoA b. Energy Storage - Liver will then store energy by two ways: i. Glycogen synthesis 1. How we store carbohydrate 2. If we have excess carbohydrates, store them by glycogenesis ii. De novo lipogenesis/VLDL synthesis and secretion 1. De novo lipogenesis a. When consume excess carb, convert the carbohydrate to fat 2. VLDL synthesis and secretion a. How we transport the fat out of the liver c. Anything else that needs to happen i. Glucose metabolism by pentose phosphate pathway 1. Making pentose sugars ii. Conversion of lactate, glycerol, amino acids and monosaccharides to glycolytic intermediate - Ordering by what the liver will do first: - Supply energy to the liver - Glycolysis, TCA cycle, allowing liver to make ATP - Once liver has enough energy, will do one of two things: - Will store excess nutrients - stores carbs as glycogen - Or convert excess carb and convert to FA, triglyceride and send it out of the liver to be stored in adipose tissue 6. As we come out of the liver, blood glucose goes to the heart and the heart pumps blood to tissues a. Brain i. Energy metabolism 1. Oxidation to CO2 + H2O 2. Brain is mostly oxidative 3. Any metabolism of glucose in brain goes through glycolysis and TCA cycle 4. Aerobic b. Erythrocytes i. Energy metabolism for RBC 1. Metabolism to lactate 2. RBC lack mitochondria so proceed anaerobically 3. Glucose > pyruvate via glycolysis and convert pyruvate to lactate 4. Are primarily glycolytic ii. Oxidation by pentose phosphate pathway 1. Making pentose sugars c. Skeletal muscle and adipose tissue i. Energy metabolism 1. Oxidation to CO2 + H2O a. From glycolysis 2. Metabolism to lactate a. Anaerobic b. Produces a small amt of energy very quickly ii. Skeletal muscle stores carbohydrate as glycogen **adipose does not do this** 1. Is the only tissue other than the liver that can store carb as glycogen 2. Skeletal muscle glycogen can only be used in the cell its stored in iii. Synthesis of glycerol used to make TAG or IMTG iv. TAG synthesis 1. TAG = triglyceride 2. How we store excess carb 3. Most of it is stored in adipose tissue but do have some storage of fat in skeletal muscle as IMTG (intramuscular triglyceride) 7. Products being secreted from the cells of the tissues a. Lactate is secreted by the brain, RBC, skeletal muscle, and adipose tissue b. Lactate will eventually go back to the liver c. The liver is one of the only tissues that can use lactate i. Liver can take up lactate, convert it to pyruvate and reverse the glycolytic pathway via gluconeogenesis to regenerate glucose Starved state of carbohydrate metabolism: - Generally lasts from the time of last meal to 48 hours of no food consumption 1. Start in the liver a. Glycogen degradation i. Use the liver glycogen stores to maintain blood glucose concentrations 1. Break down glycogen b. Gluconeogenesis i. Making carbohydrate from a non-carbohydrate precursor ii. Like using lactate or amino acids iii. To maintain blood glucose 2. Out of the liver, to peripheral tissues a. Adipose tissue i. Lipolysis - breakdown of stored triglycerides into free FA and glycerol b. Skeletal muscle i. Switch to oxidation of FA 1. If not eating extends beyond 4 hours, use more FA as an energy source ii. Glycogen breakdown 1. Stores excess carb as glycogen but has to be used by that cell iii. Proteolysis and release of amino acids 1. Not common but is a way to produce precursors for gluconeogenesis c. RBC i. Metabolism to lactate ii. Oxidation by pentose phosphate pathway iii. Exactly the same as in fed state iv. RBC do not change metabolism when going from fed to fasted bc they consistently need a supply of glucose d. Brain i. Oxidation to CO2 + H2O ii. Still have blood glucose in circulation iii. Brain will use glucose as its primary fuel source until we get beyond 48 hours (into starvation) 3. Secrete lactate, amino acids, glycerol out of tissues which will go back to the liver to be used as precursors for gluconeogenesis 2 scenarios looking at our glucose metabolism, insulin regulation, and our responses: Fed state GLUT 4 ○ GLUT 4 = insulin-sensitive glucose transporter ○ When insulin is present, binds to a receptor on the cell and the receptor transports a message into the cell to tell it lower blood glucose Signals GLUT4 ○ When GLUT4 is signaled, produce more GLUT4 ○ Have more GLUT4 on the surface of the cell so GLUT4 can transport glucose from circulation into the cytoplasm of the cell GLUT4 gene ○ Gene makes GLUT4 RNA which makes GLUT4 proteins stored in vesicles ○ Vesicles store the proteins Vesicles move towards plasma membrane of the cell Insulin has moved from GLUT4 from inside the vesicle to cause it to embed itself in the plasma membrane of the cell GLUT4 moves glucose from the circulation/interstitial space into the cell GLUT4 is found in two tissues ○ Skeletal muscle and adipose tissue ○ These are the only tissues that have an insulin sensitive glucose transporter Starved state There is much less GLUT4 RNA in the starved state Want to conserve as much as possible by producing as few proteins as possible ○ If we don’t have much glucose, don’t need GLUT4 to transport that glucose ○ Minimize how much GLUT4 we produce GLUT4 still moves towards the plasma membrane and embedded in plasma membrane- there is just less of it during starved state ○ There is glucose coming into the cell, there is just less glucose and less GLUT4 If a person has been starving… ○ Do not want to give them a normal, full meal ○ When consume carbs again, get an exaggerated response of insulin ○ Do not have enough GLUT4 available at first Pancreas & Liver function with glucose: Liver: GLUT2 brings glucose into liver Glucokinase allows glucose to enter glycolysis - turns it into glucose–6- phosphate 3 pathways glucose-6-phosphate can go: ○ Can be turned into pyruvate via glycolysis and then go through TCA & ETC Our first option is always to make energy (second is to store) ○ Can be stored into glycogen to be stored ○ Converted to pyruvate and then turned into FA to be stored Do not want to store FA in the liver Sent out of liver to be stored in adipose or skeletal muscle Pancreas: Glucose sensor As blood glucose increases, the pancreas senses that GLUT2 takes glucose into pancreas Glucokinase enzyme allows glucose to go through glycolysis ○ Creates glucose-6-phosphate ○ Eventually becomes pyruvate ○ Get 2 ATP from glycolysis ○ We get an increased ATP > ADP ratio Tells us we are producing more energy in the pancreas The increased ATP > ADP ratio tells the pancreas to synthesize and secrete insulin Insulin leaves the pancreas and signals other cells to take up glucose These cells with either use for energy or store it as glycogen or triglyceride Metabolism for different cell types: RBC ○ Anaerobic - lacks a mitochondria ○ Glucose comes in > glucose-6-phosphate > pyruvate > lactate Glycolysis - make 2 ATP Small amount of energy v quickly Brain ○ Only aerobic ○ Glucose > glucose-6-phosphate > glycolysis > pyruvate > acetyl CoA > TCA > ETC ○ Make between 32-38 ATP per glucose for aerobic Skeletal muscle ○ Glucose comes in, goes to glucose-6-phosphate ○ Glucose 6-phosphate either: Goes to glycogen to be stored Is converted to pyruvate which has 2 options: Is turned into lactate by anaerobic metabolism Turns into acetyl CoA > TCA > ETC by aerobic metabolism ○ Can go through anaerobic metabolism, aerobic metabolism, or be stored in the cell Adipose tissue ○ Glucose > glucose-6-phosphate; glucose-6-phosphate has 2 options: Turned into glycerol which is used to make TAG Pyruvate can also be turned into acetyl-CoA and go through TCA > ETC aerobically Liver ○ Glucose > glucose-6-phosphate; has 3 options: Pentose phosphate pathway Be turned into pyruvate; pyruvate has 2 options Be turned into lactate by anaerobic glycolysis Be turned into acetyl CoA > TCA > ETC aerobically Be turned into glycogen to be stored Glycogen can be used to increase blood glucose during fasting - is specific to the liver because the only other place carbs are stored as glycogen is the skeletal muscle and if it is stored there, can only be used there and not in circulation ○ When skeletal muscle and other tissues secrete lactate, it goes back into the liver where it can be used by the liver or kidney where it is converted back into glucose via gluconeogenesis Powerpoint #9 - Carbohydrate Metabolism pt 4 Glycolysis First half 1. Hexokinase or glucokinase phosphorylate glucose into glucose-6- phosphate a. Invest ATP - ATP > ADP allows us to do phosphorylation 2. 6-phosphofructo-1-kinase phosphorylates fructose-6-phosphate into fructose 1,6-bisphosphate a. Invest another ATP; ATP is turned into ADP which allows fructose 6-phosphate to be phosphorylated b. By now 2 sugars have been added and have done that through the investment of 2 ATP (includes step 1) ○ This first half is the part of glycolysis where we invest energy - invest 2 ATP 2nd half ○ This is the half of glycolysis where we make energy and reducing equivalents 1. Fructose 1,6-bisphosphate is turned into one molecule of glyceraldehyde 3-phosphate AND one molecule of dihydroxyacetone phosphate a. Go from one 6 carbon molecule to two 3-carbon molecules b. Both of these go through the rest of glycolysis so each reaction is doubled 2. Have 2 glyceraldehyde 3-phosphate 3. Glyceraldehyde 3-phosphate dehydrogenase turns (2) glyceraldehyde 3-phosphate into (2) 1,3-Bisphosphoglycerate a. Also reduces (2) NAD > (2) NADH + H+ 4. Phosphoglycerate kinase phosphorylates (2) ADP into (2) ATP by transferring the phosphate group from (2) 1,3-bisphosphoglycerate to ADP and therefore also creating (2) 3 phosphoglycerate a. *Kinase = phosphorylates* b. Is substrate-level phosphorylation - phosphorylate ADP into ATP 5. Pyruvate kinases phosphorylates (2) ADP into (2) ATP by transferring the phosphate group from (2) phosphoenolpyruvate to ADP a. (2) phosphoenolpyruvate is then turned into (2) pyruvate b. Substrate-level phosphorylation of ADP 6. After glycolysis, continue with anaerobic metabolism - pyruvate can be turned into lactate anaerobically a. By anaerobic metabolism, use (2) NADH to turn (2) pyruvate into (2) lactate i. Also produce (2) NAD 1. This is the NAD we need to regenerate for glyceraldehyde-3-phosphate dehydrogenase ○ After anaerobic metabolism we have: After glycolysis Put in 2 ATP in the first half Generate 4 ATP and 2 NADH/H+ in 2nd half After converting (2) pyruvate to (2) lactate Use 2 NADH Overall, we end up with solely 2 ATP 7. After glycolysis, can continue with aerobic metabolism a. (2) pyruvate is converted to (2) acetyl-CoA via pyruvate dehydrogenase complex; also make (2) NADH/H+ since have (2) pyruvate b. acetyl-CoA go through TCA cycle i. For each round of TCA cycle, get 3 NADH, 1 FADH2, 1 ATP ii. But, since it is (2) acetyl CoA per glucose, overall get 6 NADH, 2 FADH2, 2 ATP ○ After aerobic metabolism, we have: After glycolysis 2 NADH/H+ ○ Get 5 ATP from this going thru ETC 2 ATP 2 pyruvate After pyruvate dehydrogenase complex 2 NADH/H+ ○ Get 5 ATP from this going thru ETC 2 acetyl-CoA After TCA 6 NADH/H+ ○ Get 15 ATP from this going thru ETC 2 FADH2 ○ Get 3 ATP from this going thru ETC 2 ATP Overall, get 28 ATP from oxidative phosphorylation aka reducing equivalents and 4 ATP from substrate-level phosphorylation to get 32 ATP How we use the other monosaccharides: Galactose ○ Very similar to glucose ○ Produce and invest same amount of energy Fructose ○ Has 2 ways it can go into glycolysis Path 1 *less preferred Fructose uses hexokinase to be converted to fructose 6-phosphate which is now same as glucose for the rest of glycolysis Same investment and production of energy to glucose Simplest way for fructose to enter glycolysis Path 2 Use ketohexokinase and 1 ATP to make fructose 1- phosphate Fructose 1-phosphate aldolase converts fructose 1- phosphate into glyceraldehyde and dihydroxyacetone phosphate Use triose kinase to phosphorylate glyceraldehyde into glyceraldehyde 3-phosphate using an ATP At this point have 2 molecules that are the same as they were for glucose - so will just go through rest of glycolysis Can use the other monosaccharides for energy metabolism. They can also be converted to a glycolytic intermediate, or be used for FA synthesis All monosaccharides that go through glycolysis invest 2 ATP, produce 2 NADH and 4 ATP *Synthesis of glycogen: Glucose comes in, is converted to to glucose-6-phosphate like in glycolysis Instead of going from glucose-6-phosphate to fructose-6-phosphate as we do in glycolysis, glucose-6-phosphate is converted to glucose 1-phosphate Synthesis of UTP-glucose ○ A substrate for glycogen synthase Glycogen synthase enzyme ○ Add one molecule of glucose to growing polymer of glucose ○ Use UDP-glucose as a substrate *Breakdown of glycogen: In a period of fasting Glycogen is a polymer of glucose ○ Bonds are alpha 1,4 and then alpha 1,6 at branch point Glycogen enzyme Glycogen phosphorylase enzyme ○ Breaks alpha 1,4 bond by cleaving off a glucose and phosphorylate the glucose to make glucose-1-phosphate + the remaining glycogen molecule ○ Glucose-1-phosphate is not an intermediate of glycolysis - must be converted back to glucose-6-phosphate where it can then enter glycolysis Debranching enzyme ○ Cleaves alpha 1,6 bond to create free glucose Pentose-phosphate pathway: Part 1 ○ Start off with glucose-6-phosphate ○ Glucose-6-phosphate dehydrogenase Reduce NADP to NADPH + H+ Convert glucose-6-phosphate to to 6-phosphogluconolactone ○ Phosphogluconate dehydrogenase Reduce NADP to NADPH + H+ Convert 6-phosphogluconate to a ribose sugar and CO2 Part 2 ○ Take excess sugars and return them to glycolysis ○ If made excess ribose sugars, converted back to glycolytic intermediates Powerpoint #10 - Carbohydrate Metabolism pt 5 Gluconeogenesis: Synthesis of a carbohydrate from a non-carbohydrate precursors ○ 3 non-carb precursors used Lactate Comes from anaerobic metabolism Have a few purely anaerobic tissues so they constantly produce lactate Alanine We can produce alanine from pyruvate Glycerol Backbone from triglyceride Get it back when breaking down triglycerides aka stored fats Lactate’s path through gluconeogenesis ○ Lactate begins outside of mitochondria (because produce from mostly anaerobic tissues) ○ Start with (2) lactate ○ Reduce (2) NAD to (2) NADH + H+ when converting (2) lactate to (2) pyruvate Use 2 NAD Produce 2 NADH/H+ ○ Pyruvate transferred from cytoplasm to mitochondria ○ When carboxylating pyruvate to oxaloacetate, use (2) ATP Use 2 ATP ○ Convert oxaloacetate to malate (similar to shuttle where only certain things can cross mitochondrial membrane) while oxidizing (2) NADH to (2) NAD Use 2 NADH ○ Malate crosses and is now in cytoplasm where it is converted back to oxaloacetate while (2) NAD are reduced to (2) NADH Use 2 NAD Produce 2 NADH ○ Use 1 ATP to convert oxaloacetate to phosphoenolpyruvate Use 1 ATP per oxaloacetate so technically 2 ○ (2) 3-Phosphoglycerate is converted to to (2) 1,3 Bisphosphoglycerate while using 2 ATP Use 2 ATP ○ (2) 1,3 bisphosphate is converted to glyceraldehyde 3-phosphate dehydrogenase while oxidizing 2 NADH/H+ to NAD Use 2 NADH ○ Glucose 6-phosphatase How we generate free glucose Removes the phosphate from glucose-6-phosphate to form glucose Glucose can now be secreted out of liver and into circulation to maintain blood glucose Allows glucose to be secreted out of liver Energy molecules involved w lactate going through gluconeogensis ○ Use 6 ATP to produce glucose from lactate ○ If we are not consuming enough carbs, have to make them but this requires energy Alanine through gluconeogenesis ○ Difference in using alanine is that it enters gluconeogensis at a different place ○ When we convert lactate to pyruvate, produce 2 NADH but when we convert alanine to pyruvate, we do not produce any reducing equivalents ○ Because we do not get the production of the 2 NADH, that is more energy we have to invest for alanine > glucose Because 2.5 ATP per NADH, that requires 5 more ATP than lactate > glucose and requires 11 ATP total Glycerol (from lipolysis which is the breakdown of stored fat) through gluconeogenesis ○ Consume 2 ATP, produce 2 NADH ○ Net positive of 3 ATP ○ When going through lipolysis, body is generally fasting Alanine and lactate are both energy intensive ○ Use between 6-11 ATP 2 organs that can go through gluconeogenesis = liver and kidney Tissues that produce precursors for gluconeogensis Adipose ○ Break down stored fat into glycerol and fatty acids Skeletal muscle ○ When we do anaerobic metabolism, will convert glucose > pyruvate which will go to lactate When exercising ○ Can break down proteins in times of need Is generally during starvation Two amino acids commonly broken down: glutamine and alanine RBC ○ Are purely anaerobic so always producing lactate 2 Substrate Cycles Quarry cycle ○ Any tissue that produces lactate can participate in this cycle ○ Start with glucose in the blood, take it up into a tissue (ex: skeletal muscle), converted to pyruvate and then to lactate via anaerobic metabolism ○ The lactate is then secreted out into the bloodstream where it will go back to the liver ○ The liver will take up lactate and convert it back to glucose via gluconeogensis ○ Start and end with glucose ○ Go from glucose > lactate and then from lactate > glucose Glucose-alanine cycle ○ Start with glucose in the blood, take it up into a tissue, convert the glucose to pyruvate, convert pyruvate to alanine ○ Can secrete alanine out of the tissue, into the blood, and back to the liver where the liver will take up the alanine, convert it to pyruvate and then send pyruvate through gluconeoegensis back to glucose Powerpoint #11 - Carbohydrate Regulation pt 1 Regulating glycolysis: Insulin - fed state ○ Regulate the step of 6 phosphofructo-1-kinase (6PF-1K) Enzyme that’s in glycolysis ○ Insulin suggests we are in the fed state Glucose comes in, signals pancreas to release insulin ○ 6PF-2K makes a metabolite (F2, 6BP) and this metabolite regulates 2 enzymes: How F2,6BP regulates 6PF-1K Up-regulates 6PF-1K so up-regulates glycolysis How F2,6BP regulates F1,6-Pase F1,6-Pase ○ Removes a phosphate Down-regulate fructose 1,6 bisphosphatase Attempting to slow down gluconeogenesis F26BP does allosteric to both ○ Insulin signals phosphorylation of ser-32 which causes 6PF-2K to make the metabolite F2,6BP from F6P ○ F2,6BP regulates 2 enzymes by allosteric regulation: How F2,6BP regulates 6PF-1K Up-regulates to upregulate glycolysis 6PF-1K is an enzyme in glycolysis and phosphorylates fructose-6-phosphate into fructose 1, 6-bisphosphate How F2,6BP regulates F1,6-Pase Downregulates because F1,6-Pase turns F1,6BP into F6P (gluconeogenesis and want to slow down gluconeogenesis) Glucagon - fasted state ○ Glucagon is opposing hormone to insulin Both glucagon and insulin are produced in the pancreas Insulin attempts to decrease blood glucose concentration and glucagon attempts to increase blood glucose concentration ○ Ser32 down-regulates 6PF-2K Produce less 6PF-2K so have less F2,6BP If we have less F2,6BP, it has less of an impact on 6PF-1K and F1,6-Pase Not going to up-regulate 6PF-1K Not going to down-regulate F1,6-Pase Both are in neutral state Glycogen synthesis: Glycogen synthase a (deP) (active) ○ Dephosphorylated ○ Active state ○ When glycogen synthase is dephosphorlyated, synthesize glycogen by adding glucose residues to polymer Glycogen synthase b (P) (less active) ○ Phosphorlyated ○ Down-regulate glycogen synthase to slow down glycogen synthesis Transitioning from phosphorlyated, less active state to the dephosphorlyated, active state ○ Fasted state - glucagon present If we are in a fasted state, want to inactivate glycogen synthase - attempting to increase blood glucose concentration so want to break down glycogen, not synthesize it When glucagon is present, signals cAMP which then regulates protein kinase A which phosphorlyates inhibitor-1 a (P) which then down-regulates protein phosphatase 1 (PP1) If we down-regulate PP1, keep glycogen synthase in its inactive, phosphorlyated state ○ Transitioning from fasted to fed state PP1 is up-regulated aka active Dephosphorlyate glycogen synthase making it active Fed to fasted - insulin present ○ When insulin is present, down-regulate glycogen synthase kinase Kinases phosphorlyate things so if glycogen synthase kinase does not phosphorlyate, keeps glycogen synthase dephosphorlyated aka active ○ Transition to a fasted state aka glucagon Anytime glucagon is present, proceeds through cAMP Glucagon activates cAMP which activates two kinases: phosphorylase kinase, protein kinase A Phosphorlyate glycogen synthase which deactivates it - When we are in a fasted state, glucagon is present - Glucagon is doing two things at once: - Inhibiting PP1 - Up-regulating the two kinases so we phosphorlyate glycogen synthase to inactive it Breakdown of glycogen: If we are breaking down glycogen, we are either in a fasted state, consuming no carbohydrates or consuming a diet with little carbohydrates Glycogen phosphorylase ○ When it is dephosphorylated is in its less active state ○ When is phosphorlyated, is in its active state ○ Is the opposite in glycogen synthesis Upregulate glycogen synthesis while down-regulating glycogen breakdown OR Downregulate glycogen synthesis while upregulating glycogen breakdown Go through glucagon, cAMP, and protein kinase A When we are fasting, glucagon signals the top and bottom halves at same time First half ○ Glucagon, cAMP, protein kinase ○ When phosphorylase kinase is active, phosphorlyates glycogen phosphorlyase so it becomes active and we begin to break down glycogen Break alpha 1,4 bond to get glucose 1,4 phosphate Substarte is glycogen, enzyme is glycogen phsophorylase, product is glucose 1,4 phosphate which Second half ○ Phosphorlyates inhibitor 1 which inhibits PP1 so we slow down the dephosphorylation of glycogen phosphorylase ○ Glucagon, cAMP, protein kinase A Ppt 12 - Carb Regulation pt 2 Glycolysis & Gluconeogenesis figure: Molecules or hormones that regulate the enzymatic steps of glycolysis ○ Main regulated step of glycolysis = 6PF-1K (uses energy aka ATP): ○ Up-regualtion of 6PF-1k (molecules that up-regulate 6PF-1K) 1. Insulin upregulates F2, 6BP a. F2,6BP regulates 6PF-1K (Upregulating 6PF-1K upregulates glycolysis) 2. AMP 3. Inorganic phosphate 4. Insulin itself ○ Down-regulation 6PF-1K 1. ATP 2. Citrate 3. Protons (H+) - Signals the cell we have ample energy when these are present so do not need to proceed through glycolysis and would instead synthesize glycogen ○ Other steps of glycolysis that are also regulated, regulated steps are those which are consuming or producing energy aka ATP: Glucokinase or hexokinase (uses energy aka ATP) Up-regulation ○ Glucose Glucose up-regulates hexokinase or glucokinase Want to convert glucose to glucose 6- phosphate so we can decrease free glucose conc in the cell so we can maintain the concentration gradient ○ Fructose 1-P Up-regulates glucokinase/hexokinase ○ Insulin itself Up-regulates glucokinase/hexokinase Increases the concentration of the enzyme glucokinase or hexokinase Pyruvate kinase Last step of glycolysis - where pyruvate is produced Produces ATP Up-regulation ○ Fructose 1,6 BP Intermediate product of glycolysis The more Fructose 1,6 BP we produce, the more it upregulates pyruvate kinase ○ Insulin dephosphorlyates the enzyme which increases its activity ○ Insulin increases the concentration of the enzyme Down-regulation - decreasing activity of enzyme pyruvate kinase (only want to produce how much ATP we need to produce) ○ ATP ○ Alanine Molecules or hormones that regulate the enzymatic steps of gluconeogenesis ○ Acetyl CoA Upregulates pryuavte carboxylase ○ Insulin decreases the concentration of phosphooenolpyruvate Downregulates ○ AMP Down regulates F 1,6BP AMP signals low energy status so wouldn’t want to go through gluconeogenesis ○ Insulin up-regulates F2,6BP F2, 6BP down-regulates gluconeogenesis ○ Insulin decreases the concentration of of glucose-6-phosphatase