Unit 2 First Law of Thermodynamics PDF
Document Details
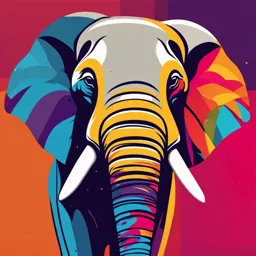
Uploaded by AstonishingObsidian
Maharaja Sayajirao University of Baroda
Tags
Summary
This document presents a detailed discussion and explanation of the First Law of Thermodynamics, including various concepts like Joule's experiments, the conservation of energy, and practical applications to different processes. It covers the principles of thermodynamics for both closed and steady flow systems.
Full Transcript
Unit 2 First law of Thermodynamics: JOULE’S EXPERIMENT SET-UP & ITS SIGNIFICANCE Fig. 4.1 Heat and work. LAW OF CONSERVATION OF ENERGY In the early part of nineteenth century the scientists developed the concept of energy and hypothesis that it can be neither created...
Unit 2 First law of Thermodynamics: JOULE’S EXPERIMENT SET-UP & ITS SIGNIFICANCE Fig. 4.1 Heat and work. LAW OF CONSERVATION OF ENERGY In the early part of nineteenth century the scientists developed the concept of energy and hypothesis that it can be neither created nor destroyed; this came to be known as the law of the conservation of energy. The first law of thermodynamics is merely one statement of this general law/principle with particular reference to heat energy and mechanical energy i.e., work. The first law of thermodynamics, also known as the conservation of energy principle, provides a sound basis for studying the relationships among the various forms of energy and energy interactions. Based on experimental observations, the first law of thermodynamics states that energy can be neither created nor destroyed during a process; it can only change forms. Therefore, every bit of energy should be accounted for during a process. FIRST LAW FOR A CLOSED SYSTEM UNDERGOING A CYCLE Consider a cycle in which net-work is done by the system. Since energy cannot be created, this mechanical energy must have been supplied from some source of energy. Now the system has been returned to its initial state: Therefore, its intrinsic energy is unchanged, and hence the mechanical energy has not been provided by the system itself. The only other energy involved in the cycle is the heat which was supplied and rejected in various processes. Hence, by the law of conservation of energy, the net work done by the system is equal to the net heat supplied to the system. The First Law of Thermodynamics can, therefore, be stated as follows: “When a system undergoes a thermodynamic cycle then the net heat supplied to the system from the surroundings is equal to net work done by the system on its surroundings. The first law of Thermodynamics cannot be proved analytically, but experimental evidence has repeatedly confirmed its validity, and since no phenomenon has been shown to contradict it, the first law is accepted as a law of nature. It may be remarked that no restriction was imposed which limited the application of first law to reversible energy transformation. Hence the first law applies to reversible as well as irreversible transformations: For non-cyclic process, a more general formulation of first law of thermodynamics is required. A new concept which involves a term called internal energy fulfills this need. — The First Law of Thermodynamics may also be stated as follows: “Heat and work are mutually convertible but since energy can neither be created nor destroyed, the total energy associated with an energy conversion remains constant”. Or — “No machine can produce energy without corresponding expenditure of energy, i.e., it is impossible to construct a perpetual motion machine of first kind”. FIRST LAW OF THERMODYNAMICS FOR A CLOSED SYSTEM UNDERGOING A CHANGE OF STATE If there are more energy transfer quantities involved in the process, as shown in figure 4.4, the first law gives, APPLICATION OF FIRST LAW TO A PROCESS When a process is executed by a system, the change in stored energy of the system is numerically equal to the net heat interactions minus the net-work interaction during the process. If the electric, magnetic and chemical energies are absent and changes in potential and kinetic energy for a closed system are neglected, the above equation can be written as Generally, when heat is added to a system its temperature rises and external work is performed due to increase in volume of the system. The rise in temperature is an indication of increase of internal energy. Heat added to the system will be considered as positive and the heat removed or rejected, from the system, as negative. PERPETUAL MOTION MACHINE OF THE FIRST KIND—PMM 1 The first law of thermodynamics states the general principle of the conservation of energy. Energy is neither created nor destroyed, but only gets transformed from one form to another. There can be no machine which would continuously supply mechanical work without some form of energy disappearing simultaneously (Fig. 2.4). Such a fictitious machine is called a perpetual motion machine of the first kind, or in brief, PMM 1. A PMM 1 is thus impossible. Fig. 2.4. A PPM 1. Fig. 2.5. The converse of PMM 1. — The converse of the above statement is also true, i.e., there can be no machine which would continuously consume work without some other form of energy appearing simultaneously (Fig.2.5). FIRST LAW OF THERMODYNAMICS FOR STEADY FLOW PROCESS In many practical problems, the rate at which the fluid flows through a machine or piece of apparatus are constant. This type of flow is called steady flow. Assumptions: The following assumptions are made in the system analysis: 1. The mass flow through the system remains constant. 2. Fluid is uniform in composition. 3. The only interaction between the system and surroundings are work and heat. 4. The state of fluid at any point remains constant with time. 5. In the analysis only potential, kinetic and flow energies are considered. Fig. 2.6 A This equation is applicable to any medium in any steady flow. It is applicable not only to rotary machines such as centrifugal fans, pumps and compressors but also to reciprocating machines such as steam engines. In a steady flow the rate of mass flow of fluid at any section is the same as at any other section. Consider any section of cross-sectional area A, where the fluid velocity is C, the rate of volume flow past the section is CA. Also, since mass flow is volume flow divided by specific volume, ENERGY RELATIONS FOR FLOW PROCESS The energy equation (m kg of fluid) for a steady flow system is given as follows: ENGINEERING APPLICATIONS OF STEADY FLOW ENERGY EQUATION (S.F.E.E.) Following are the list where we can apply SFEE with various conditions and assumptions: - 1. Nozzle, 2. Diffuser, 3. Boiler, 4. Turbine, 5. Compressor, 6. Pump, 7. Heat exchanger (Condensor and Evaporator Also) Now step by step we will apply for each case.... 1. Steam Nozzle and Diffuser In case of a nozzle and diffuser as the enthalpy of the fluid decreases and pressure drops simultaneously the flow of fluid is accelerated. This is generally used to convert the part of the energy of steam into kinetic energy of steam supplied to the turbine. Fig.2.7 Steam Nozzle Fig.2.7 A shows a commonly used convergent-divergent nozzle. For this system, 2. APPLICATIONS OF STEADY FLOW ENERGY EQUATION (S.F.E.E.) for Boiler A boiler transfers heat to the incoming water and generates the steam. The system is shown in Fig. 2.3 B Fig. 2.8 Boiler The steady flow equation can be expressed as follows: 3. APPLICATIONS OF STEADY FLOW ENERGY EQUATION (S.F.E.E.) for Boiler A. Water Turbine Refer to Fig. 2.9 A. In a water turbine, water is supplied from a height. The potential energy of water is converted into kinetic energy when it enters into the turbine and part of it is converted into useful work which is used to generate electricity. Considering Centre of turbine shaft as datum, the energy equation can be written as follows : Fig. 2.9 A Water Turbine W is positive because work is done by the system (or work comes out of the boundary). B Steam or Gas Turbine In a steam or gas turbine steam or gas is passed through the turbine and part of its energy is converted into work in the turbine. This output of the turbine runs a generator to produce electricity as shown in Fig. 2.9 B. The steam or gas leaves the turbine at lower pressure or temperature. Fig. 2.9 B Steam Turbine Applying energy equation to the system. The sign of Q is negative because heat is rejected (or comes out of the boundary). The sign of W is positive because work is done by the system (or work comes out of the boundary). 4. APPLICATIONS OF STEADY FLOW ENERGY EQUATION (S.F.E.E.) for Compressor A. Centrifugal Compressor Refer to Fig. 2.10 A. A centrifugal compressor compresses air and supplies the same at moderate pressure and in large quantity. Fig. 2.10 A. Centrifugal compressor Applying energy equation to the system (Fig. 2.10 A) Δ Z = 0 (generally taken) The Q is taken as negative as heat is lost from the system and W is taken as negative as work is supplied to the system. B. Reciprocating Compressor Refer Fig. 2.10 B The reciprocating compressor draws in air from atmosphere and supplies at a considerable higher pressure in small quantities (compared with centrifugal compressor). The reciprocating compressor can be considered as steady flow system provided the control volume includes the receiver which reduces the fluctuations of flow considerably. Refer Fig. 2.10 B Reciprocating Compressor Applying energy equation to the system, we have: ΔPE = 0 and ΔKE = 0 since these changes are negligible compared with other energies. 5. APPLICATIONS OF STEADY FLOW ENERGY EQUATION (S.F.E.E.) for Pump Fig 2.11 Centrifugal Water Pump A centrifugal water pump draws water from a lower level and pumps to higher level as shown in Fig. 2.11. Work is required to run the pump and this may be supplied from an external source such as an electric motor or a diesel engine. Here Q = 0 and Δ u = 0 as there is no change in temperature of water; v1 = v2 = v. Applying the energy equation to the system The sign of W is negative because work is done on the system (or work enters the boundary). 6. APPLICATIONS OF STEADY FLOW ENERGY EQUATION (S.F.E.E.) for Heat Exchanger A. Condenser (One type of heat exchanger) The condenser is used to condense the steam in case of steam power plant and condense the refrigerant vapour in the refrigeration system using water or air as cooling medium. Fig. 2.12 A shows the system. Fig. 2.11 A Condensor For this system: ΔPE = 0, ΔKE = 0 (as their values are very small compared with enthalpies) W = 0 (since neither any work is developed nor absorbed) Using energy equation to steam flow Assuming there are no other heat interactions except the heat transfer between steam and water, then Q = Heat gained by water passing through the condenser B. Evaporator (One type of heat exchanger) An evaporator is an equipment used in refrigeration plant to carry heat from the refrigerator to maintain the low temperature. Here the refrigerant liquid is passed through the evaporator and it comes out as vapour absorbing its latent heat from the surroundings of the evaporator. Fig. 2.11 B shows the system. For this system Fig. 2.11 B Evaporator ΔPE = 0, ΔKE = 0 W = 0 [ As No work is absorbed or supplied] Applying the energy equation to the system Q is taken as + ve because heat flows from the surroundings to the system as the temperature in the system is lower than the surroundings. LIMITATIONS OF FIRST LAW OF THERMODYNAMICS The limitation of the first law of thermodynamics is that it does not say anything about the direction of flow of heat. It does not say anything whether the process is a spontaneous process or not. The reverse process is not possible. In actual practice, the heat doesn’t convert completely into work. If it would have been possible to convert the whole heat into work, then we could drive ships across the ocean by extracting heat from the water of the ocean.