Treatment of Dairy Processing Wastewaters PDF
Document Details
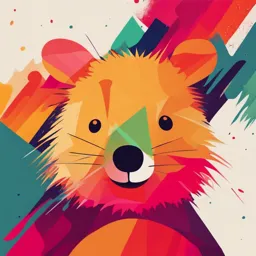
Uploaded by PrincipledMaracas
Stellenbosch University
2006
Trevor J. Britz, Corné van Schalkwyk, Yung-Tse Hung
Tags
Summary
This document explores the treatment of dairy processing wastewaters. It examines the pollution potential of various dairy products, such as whole milk, skim milk, buttermilk and cream, and the different production processes involved. It highlights the environmental challenges and cost implications associated with waste disposal.
Full Transcript
1 Treatment of Dairy Processing Wastewaters Trevor J. Britz and Corné van Schalkwyk University of Stellenbosch, Matieland, South Africa Yung-Tse Hung Cleveland State University, Cleveland, Ohio, U.S.A. 1.1 INTRODUCTION The dairy industry is generally considered to be the largest source of fo...
1 Treatment of Dairy Processing Wastewaters Trevor J. Britz and Corné van Schalkwyk University of Stellenbosch, Matieland, South Africa Yung-Tse Hung Cleveland State University, Cleveland, Ohio, U.S.A. 1.1 INTRODUCTION The dairy industry is generally considered to be the largest source of food processing wastewater in many countries. As awareness of the importance of improved standards of wastewater treatment grows, process requirements have become increasingly stringent. Although the dairy industry is not commonly associated with severe environmental problems, it must continually consider its environmental impact — particularly as dairy pollutants are mainly of organic origin. For dairy companies with good effluent management systems in place , treatment is not a major problem, but when accidents happen, the resulting publicity can be embarrassing and very costly. All steps in the dairy chain, including production, processing, packaging, transportation, storage, distribution, and marketing, impact the environment. Owing to the highly diversified nature of this industry, various product processing, handling, and packaging operations create wastes of different quality and quantity, which, if not treated, could lead to increased disposal and severe pollution problems. In general, wastes from the dairy processing industry contain high concentrations of organic material such as proteins, carbohydrates, and lipids, high concentrations of suspended solids, high biological oxygen demand (BOD) and chemical oxygen demand (COD), high nitrogen concentrations, high suspended oil and/or grease contents, and large variations in pH, which necessitates “specialty” treatment so as to prevent or minimize environmental problems. The dairy waste streams are also characterized by wide fluctuations in flow rates, which are related to discontinuity in the production cycles of the different products. All these aspects work to increase the complexity of wastewater treatment. The problem for most dairy plants is that waste treatment is perceived to be a necessary evil ; it ties up valuable capital, which could be better utilized for core business activity. Dairy wastewater disposal usually results in one of three problems: (a) high treatment levies being charged by local authorities for industrial wastewater; (b) pollution might be caused when untreated wastewater is either discharged into the environment or used directly as irrigation water; and (c) dairy plants that have already installed an aerobic biological system are faced with the problem of sludge disposal. To enable the dairy industry to contribute to water conservation, an efficient and cost-effective wastewater treatment technology is critical. 1 © 2006 by Taylor & Francis Group, LLC 2 Britz et al. Presently, plant managers may choose from a wide variety of technologies to treat their wastes. More stringent environmental legislation as well as escalating costs for the purchase of fresh water and effluent treatment has increased the impetus to improve waste control. The level of treatment is normally dictated by environmental regulations applicable to the specific area. While most larger dairy factories have installed treatment plants or, if available, dispose of their wastewater into municipal sewers, cases of wastewater disposal into the sea or disposal by means of land irrigation do occur. In contrast, most smaller dairy factories dispose of their wastewater by irrigation onto lands or pastures. Surface and groundwater pollution is, therefore, a potential threat posed by these practices. Downloaded by [Universidade de Sao Paulo (USP) (CRUESP)] at 13:22 16 August 2016 Because the dairy industry is a major user and generator of water, it is a candidate for wastewater reuse. Even if the purified wastewater is initially not reused, the dairy industry will still benefit from in-house wastewater treatment management, because reducing waste at the source can only help in reducing costs or improving the performance of any downstream treatment facility. 1.2 DAIRY PROCESSES AND COMPOSITION OF DAIRY PRODUCTS Before the methods of treatment of dairy processing wastewater can be appreciated, it is important to be acquainted with the various production processes involved in dairy product manufacturing and the pollution potential of different dairy products (Table 1.1). A brief summary of the most common processes is presented below. 1.2.1 Pasteurized Milk The main steps include raw milk reception (the first step of any dairy manufacturing process), pasteurization, standardization, deaeration, homogenization and cooling, and filling of a variety of different containers. The product from this point should be stored and transported at 48C. 1.2.2 Milk and Whey Powders This is basically a two-step process whereby 87% of the water in pasteurized milk is removed by evaporation under vacuum and the remaining water is removed by spray drying. Whey powder can be produced in the same way. The condensate produced during evaporation may be collected and used for boiler feedwater. 1.2.3 Cheese Because there are a large variety of different cheeses available, only the main processes common to all types will be discussed. The first process is curd manufacturing, where pasteurized milk is mixed with rennet and a suitable starter culture. After coagulum formation and heat and mechanical treatment, whey separates from the curd and is drained. The finished curd is then salted, pressed, and cured, after which the cheese is coated and wrapped. During this process two types of wastewaters may arise: whey, which can either be disposed of or used in the production of whey powder, and wastewater, which can result from a cheese rinse step used during the manufacturing of certain cheeses. © 2006 by Taylor & Francis Group, LLC Treatment of Dairy Processing Wastewaters 3 Table 1.1 Reported BOD and COD Values for Typical Dairy Products and Domestic Sewage Product BOD5 (mg/L) COD (mg/L) Reference Whole milk 114,000 183,000 4 110,000 190,000 5 120,000 6 104,000 7 Skim milk 90,000 147,000 4 Downloaded by [Universidade de Sao Paulo (USP) (CRUESP)] at 13:22 16 August 2016 85,000 120,000 5 70,000 6 67,000 7 Buttermilk 61,000 134,000 4 75,000 110,000 5 68,000 7 Cream 400,000 750,000 4 400,000 860,000 5 400,000 6 399,000 7 Evaporated milk 271,000 378,000 4 208,000 7 Whey 42,000 65,000 4 45,000 80,000 5 40,000 6 34,000 7 Ice cream 292,000 7 Domestic sewage 300 500 4, 5 BOD, biochemical oxygen demand; COD, chemical oxygen demand. Source: Refs. 4–7. 1.2.4 Butter Cream is the main raw material for manufacturing butter. During the churning process it separates into butter and buttermilk. The drained buttermilk can be powdered, cooled, and packed for distribution, or discharged as wastewater. 1.2.5 Evaporated Milk The milk is first standardized in terms of fat and dry solids content after which it is pasteurized, concentrated in an evaporator, and homogenized, then packaged, sterilized, and cooled for storage. In the production of sweetened condensed milk, sugar is added in the evaporation stage and the product is cooled. 1.2.6 Ice Cream Raw materials such as water, cream, butter, milk, and whey powders are mixed, homogenized, pasteurized, and transferred to a vat for ageing, after which flavorings, colorings, and fruit are added prior to freezing. During primary freezing the mixture is partially frozen and air is incorporated to obtain the required texture. Containers are then filled and frozen. © 2006 by Taylor & Francis Group, LLC 4 Britz et al. 1.2.7 Yogurt Milk used for yogurt production is standardized in terms of fat content and fortified with milk solids. Sugar and stabilizers are added and the mixture is then heated to 608C, homogenized, and heated again to about 958C for 3 –5 minutes. It is then cooled to 30 –458C and inoculated with a starter culture. For set yogurts, the milk base is packed directly and the retail containers are incubated for the desired period, after which they are cooled and dispatched. For stirred yogurts, the milk base is incubated in bulk after which it is cooled and packaged, and then distributed. Downloaded by [Universidade de Sao Paulo (USP) (CRUESP)] at 13:22 16 August 2016 1.2.8 Wastewater from Associated Processes Most of the water consumed in a dairy processing plant is used in associated processes such as the cleaning and washing of floors, bottles, crates, and vehicles, and the cleaning-in-place (CIP) of factory equipment and tanks as well as the inside of tankers. Most CIP systems consist of three steps: a prerinse step to remove any loose raw material or product remains, a hot caustic wash to clean equipment surfaces, and a cold final rinse to remove any remaining traces of caustic. 1.3 CHARACTERISTICS AND SOURCES OF WASTEWATER The volume, concentration, and composition of the effluents arising in a dairy plant are dependent on the type of product being processed, the production program, operating methods, design of the processing plant, the degree of water management being applied, and, subsequently, the amount of water being conserved. Dairy wastewater may be divided into three major categories: 1. Processing waters, which include water used in the cooling and heating processes. These effluents are normally free of pollutants and can with minimum treatment be reused or just discharged into the storm water system generally used for rain runoff water. 2. Cleaning wastewaters emanate mainly from the cleaning of equipment that has been in contact with milk or milk products, spillage of milk and milk products, whey, pressings and brines, CIP cleaning options, and waters resulting from equipment malfunctions and even operational errors. This wastewater stream may contain anything from milk, cheese, whey, cream, separator and clarifier dairy waters , to dilute yogurt, starter culture, and dilute fruit and stabilizing compounds. 3. Sanitary wastewater, which is normally piped directly to a sewage works. Dairy cleaning waters may also contain a variety of sterilizing agents and various acid and alkaline detergents. Thus, the pH of the wastewaters can vary significantly depending on the cleaning strategy employed. The most commonly used CIP chemicals are caustic soda, nitric acid, phosphoric acid, and sodium hypochloride ; these all have a significant impact on wastewater pH. Other concerns related to CIP and sanitizing strategies include the biochemical oxygen demand (BOD) and chemical oxygen demand (COD) contributions (normally ,10% of total BOD concentration in plant wastewater), phosphorus contribution resulting from the use of phosphoric acid and other phosphorus-containing detergents, high water volume usage for cleaning and sanitizing (as high as 30% of total water discharge), as well as general concerns regarding the impact of detergent biodegradability and toxicity on the specific waste treatment facility and the environment in general. © 2006 by Taylor & Francis Group, LLC Treatment of Dairy Processing Wastewaters 5 Dairy industry wastewaters are generally produced in an intermittent way; thus the flow and characteristics of effluents could differ between factories depending on the kind of products produced and the methods of operation. This also influences the choice of the wastewater treatment option, as specific biological systems have difficulties dealing with wastewater of varying organic loads. Published information on the chemical composition of dairy wastewater is scarce. Some of the more recent information available is summarized in Tables 1.2 and 1.3. Milk has a BOD content 250 times greater than that of sewage. It can, therefore, be expected that dairy wastewaters will have relatively high organic loads, with the main contributors being lactose, Downloaded by [Universidade de Sao Paulo (USP) (CRUESP)] at 13:22 16 August 2016 fats, and proteins (mainly casein), as well as high levels of nitrogen and phosphorus that are largely associated with milk proteins [12,17]. The COD and BOD for whey have, for instance, been established to be between 35,000 –68,000 mg/L and 30,000 –60,000 mg/L, respectively, with lactose being responsible for 90% of the COD and BOD contribution. 1.4 TREATMENT OPTIONS The highly variable nature of dairy wastewaters in terms of volumes and flow rates (which is dependent on the factory size and operation shifts) and in terms of pH and suspended solid (SS) content (mainly the result of the choice of cleaning strategy employed) makes the choice of an effective wastewater treatment regime difficult. Because dairy wastewaters are highly biodegradable, they can be effectively treated with biological wastewater treatment systems, but can also pose a potential environmental hazard if not treated properly. The three main options for the dairy industry are: (a) discharge to and subsequent treatment of factory wastewater at a nearby sewage treatment plant; (b) removal of semisolid and special wastes from the site by waste disposal contractors; or (c) the treatment of factory wastewater in an onsite wastewater treatment plant [25,26]. According to Robinson , the first two options are continuously impacted by increasing costs, while the control of allowable levels of SS, BOD, and COD in discharged wastewaters are also becoming more stringent. As a result, an increasing number of dairy industries must consider the third option of treating industrial waste onsite. It should be remembered, however, that the treatment chosen should meet the required demands and reduce costs associated with long-term industrial wastewater discharge. 1.4.1 Direct Discharge to a Sewage Treatment Works Municipal sewage treatment facilities are capable of treating a certain quantity of organic substances and should be able to deal with certain peak loads. However, certain components found in dairy waste streams may present problems. One such substance is fat, which adheres to the walls of the main system and causes sedimentation problems in the sedimentation tanks. Some form of onsite pretreatment is, therefore, advisable to minimize the fat content of the industrial wastewater that can be mixed with the sanitary wastewater going to the sewage treatment facility. Dairy industries are usually subjected to discharge regulations, but these regulations differ significantly depending on discharge practices and capacities of municipal sewage treatment facilities. Sewer charges are based on wastewater flow rate, BOD5 mass, SS, and total P discharged per day. Some municipal treatment facilities may demand treatment of high- strength industrial effluents to dilute the BOD load of the water so that it is comparable to that of domestic sewage. © 2006 by Taylor & Francis Group, LLC 6 Downloaded by [Universidade de Sao Paulo (USP) (CRUESP)] at 13:22 16 August 2016 Table 1.2 Chemical Characteristics of Different Dairy Plant Wastewaters Alkalinity BOD5 COD FOG TS TSS (mg/L as Industry (mg/L) (mg/L) pH (g/L) (mg/L) (mg/L) CaCO3) Reference Cheese 14 Cheese/whey plants 565– 5722 785 – 7619 6.2 – 11.3 – 1837– 14,205 326– 3560 225–1550 10 Cheese/whey plant 377– 2214 189 – 6219 5.2 – – 188– 2330 – 13 Cheese factory – 5340 5.22 – 4210 – 335 14 Cheese factory – 2830 4.99 – – – – 15 Cheese processing industry – 63,300 3.38 2.6 53,200 12,500 – 16 Cheese/casein product plant – 5380 6.5 0.32 – – – 15 Cheese/casein product plant 8000 – 4.5 – 6.0 0.4 – – – 17 Milk Milk processing plant – 713 – 1410 7.1 – 8.1 – 900– 1470 360– 920 – 18 Milk/yogurt plant – 4656 6.92 – 2750 – 546 14 Milk/cream bottling plant 1200– 4000 2000– 6000 8 – 11 3 –5 – 350– 1000 150–300 19, 20 Butter/milk powder Butter/milk powder plant – 1908 5.8 – 1720 – 532 14 Butter/milk powder plant 1500 – 10 – 11 0.4 – – – 17 Butter/Comte´cheese plant 1250 2520 5–7 – – – – 21 Whey Whey wastewater 35,000 – 4.6 0.8 – – – 17 Raw cheese whey – 68,814 – – 3190 1300 – 22 BOD, biological oxygen demand; COD, chemical oxygen demand; TS, total solids; TSS, total suspended solids; FOG, fats, oil and grease. Britz et al. © 2006 by Taylor & Francis Group, LLC Treatment of Dairy Processing Wastewaters Downloaded by [Universidade de Sao Paulo (USP) (CRUESP)] at 13:22 16 August 2016 Table 1.3 Concentrations of Selected Elements in Different Dairy Wastewaters Total P PO4-P TKN NH4-N Naþ Kþ Ca2þ Mg2þ Industry (mg/L) (mg/L) (mg/L) (mg/L) (mg/L) (mg/L) (mg/L) (mg/L) Reference Cheese 14 Cheese/whey plants 29– 181 6 –35 14 – 140 1– 34 263 – 1265 8.6– 155.5 1.4 – 58.5 6.5– 46.3 16 Cheese/whey plant 0.2– 48.0 0.2 –7.9 13 – 172 0.7– 28.5 – – – – 13 Cheese factory 45 – 102 – 550 140 30 35 15 Cheese/casein product plant 85 – 140 – 410 125 70 12 15 Cheese/casein product plant 100 – 200 – 380 160 95 14 17 Milk Milk/cream bottling plant – 20 –50 50 – 60 – 170 – 200 35 – 40 35– 40 5– 8 19, 20 Butter/milk powder Butter/milk powder plant 35 – 70 – 560 13 8 1 17 Butter/Comté cheese plant 50 – 66 – – – – – 21 Whey Whey wastewater 640 – 1400 – 430 1500 1250 100 17 Raw cheese whey 379 327 1462 64.3 – – – – 22 © 2006 by Taylor & Francis Group, LLC 7 8 Britz et al. In a recent survey conducted by Danalewich et al. at 14 milk processing plants in Minnesota, Wisconsin, and South Dakota, it was reported that four facilities directed both their mixed sanitary and industrial wastewater directly to a municipal treatment system, while the rest employed some form of wastewater treatment. Five of the plants that treated their wastewater onsite did not separate their sanitary wastewater from their processing wastewater, which presents a major concern when it comes to the final disposal of the generated sludge after the wastewater treatment, since the sludge may contain pathogenic microorganisms. It would thus be advisable for factories that employ onsite treatment to separate the sanitary and processing wastewaters, and dispose of the sanitary wastewater by piping directly to a sewage Downloaded by [Universidade de Sao Paulo (USP) (CRUESP)] at 13:22 16 August 2016 treatment facility. 1.4.2 Onsite Pretreatment Options Physical Screening The main purpose of screens in wastewater treatment is to remove large particles or debris that may cause damage to pumps and downstream clogging. It is also recommended that the physical screening of dairy wastewater should be carried out as quickly as possible to prevent a further increase in the COD concentration as a result of the solid solubilization. Wendorff recommended the use of a wire screen and grit chamber with a screen aperture size of 9.5 mm, while Hemming recommended the use of even finer spaced mechanically brushed or inclined screens of 40 mesh (about 0.39 mm) for solids reduction. According to Droste , certain precautionary measures should be taken to prevent the settling of coarse matter in the wastewater before it is screened. These requirements include the ratio of depth to width of the approach channel to the screen, which should be 1 : 2, as well as the velocity of the water, which should not be less than 0.6 m/sec. Screens can be cleaned either manually or mechanically and the screened material disposed of at a landfill site. pH Control As shown in Table 1.2, large variations exist in wastewater pH from different dairy factories. This may be directly attributed to the different cleaning strategies employed. Alkaline detergents generally used for the saponification of lipids and the effective removal of proteinacous substances would typically have a pH of 10 – 14, while a pH of 1.5 –6.0 can be encountered with acidic cleaners used for the removal of mineral deposits and acid-based sanitizers [11,29]. The optimum pH range for biological treatment plants is between 6.5 and 8.5 [30,31]. Extreme pH values can be highly detrimental to any biological treatment facility, not only for the negative effect that it will have on the microbial community, but also due to the increased corrosion of pipes that will occur at pH values below 6.5 and above 10. Therefore, some form of pH adjustment as a pretreatment step is strongly advised before wastewater containing cleaning agents is discharged to the drain or further treated onsite. In most cases, flow balancing and pH adjustment are performed in the same balancing tank. According to the International Dairy Federation (IDF) , a near-neutral pH is usually obtained when water used in different production processes is combined. If pH correction needs to be carried out in the balancing tank, the most commonly used chemicals are H2SO4, HNO3, NaOH, CO2, or lime. Flow and Composition Balancing Because discharged dairy wastewaters can vary greatly with respect to volume, strength, temperature, pH, and nutrient levels, flow and composition balancing is a prime requirement for © 2006 by Taylor & Francis Group, LLC Treatment of Dairy Processing Wastewaters 9 any subsequent biological process to operate efficiently. pH adjustment and flow balancing can be achieved by keeping effluent in an equalization or balancing tank for at least 6– 12 hours. During this time, residual oxidants can react completely with solid particles, neutralizing cleaning solutions. The stabilized effluent can then be treated using a variety of different options. According to the IDF , balance tanks should be adequately mixed to obtain proper blending of the contents and to prevent solids from settling. This is usually achieved by the use of mechanical aerators. Another critical factor is the size of the balance tank. This should be accurately determined so that it can effectively handle a dairy factory’s daily flow pattern at peak season. It is also recommended that a balancing tank should be large enough to allow a few hours Downloaded by [Universidade de Sao Paulo (USP) (CRUESP)] at 13:22 16 August 2016 extra capacity to handle unforeseen peak loads and not discharge shock loads to public sewers or onsite biological treatment plants. Fats, Oil, and Grease Removal The presence of fats, oil, and grease (FOG) in dairy processing wastewater can cause all kinds of problems in biological wastewater treatment systems onsite and in public sewage treatment facilities. It is, therefore, essential to reduce, if not remove FOG completely, prior to further treatment. According to the IDF , factories processing whole milk, such as milk separation plants as well as cheese and butter plants, whey separation factories, and milk bottling plants, experience the most severe problems with FOG. The processing of skim milk seldom presents problems in this respect. As previously mentioned, flow balancing is recommended for dairy processing plants. An important issue, however, is whether the FOG treatment unit should be positioned before or after the balancing tank. If the balancing tank is placed before the FOG unit, large fat globules can accumulate in the tank as the discharged effluent cools down and suspended fats aggregate during the retention period. If the balancing tank is placed after the FOG removal unit, the unit should be large enough to accommodate the maximum anticipated flow from the factory. According to the IDF , it is generally accepted that flow balancing should precede FOG removal. General FOG removal systems include the following. Gravity Traps. In this extremely effective, self-operating, and easily constructed system, wastewater flows through a series of cells, and the FOG mass, which usually floats on top, is removed by retention within the cells. Drawbacks include frequent monitoring and cleaning to prevent FOG buildup, and decreased removal efficiency at pH values above 8. Air Flotation and Dissolved Air Flotation. Mechanical removal of FOG with dissolved air flotation (DAF) involves aerating a fraction of recycled wastewater at a pressure of about 400 –600 kPa in a pressure chamber, then introducing it into a flotation tank containing untreated dairy processing wastewater. The dissolved air is converted to minute air bubbles under the normal atmospheric pressure in the tank [6,32]. Heavy solids form sediment while the air bubbles attach to the fat particles and the remaining suspended matter as they are passed through the effluent [6,9,25]. The resulting scum is removed and will become odorous if stored in an open tank. It is an unstable waste material that should preferably not be mixed with sludge from biological and chemical treatment processes since it is very difficult to dewater. FOG waste should be removed and disposed of according to approved methods. DAF components require regular maintenance and the running costs are usually fairly high. Air flotation is a more economical variation of DAF. Air bubbles are introduced directly into the flotation tank containing the untreated wastewater, by means of a cavitation aerator coupled to a revolving impeller. A variety of different patented air flotation systems are available on the market and have been reviewed by the IDF. These include the “Hydrofloat,” the “Robosep,” vacuum flotation, electroflotation, and the “Zeda” systems. © 2006 by Taylor & Francis Group, LLC 10 Britz et al. The main drawback of the DAF , is that only SS and free FOG can be removed. Thus, to increase the separation efficiency of the process, dissolved material and emulsified FOG solutions must undergo a physico-chemical treatment during which free water is removed and waste molecules are coagulated to form larger, easily removable masses. This is achieved by recirculating wastewater prior to DAF treatment in the presence of different chemical solutions such as ferric chloride, aluminum sulfate, and polyelectrolytes that can act as coalescents and coagulants. pH correction might also be necessary prior to the flotation treatment, because a pH of around 6.5 is required for efficient FOG removal. Enzymatic Hydrolysis of FOG. Cammarota et al. and Leal et al. utilized Downloaded by [Universidade de Sao Paulo (USP) (CRUESP)] at 13:22 16 August 2016 enzymatic preparations of fermented babassu cake containing lipases produced by a Penicillium restrictum strain for FOG hydrolysis in dairy processing wastewaters prior to anaerobic digestion. High COD removal efficiencies as well as effluents of better quality were reported for a laboratory-scale UASB reactor treating hydrolyzed dairy processing wastewater, and com- pared to the results of a UASB reactor treating the same wastewater without prior enzymatic hydrolysis treatment. 1.4.3 Treatment Methods Biological Treatment Biological degradation is one of the most promising options for the removal of organic material from dairy wastewaters. However, sludge formed, especially during the aerobic biodegradation processes, may lead to serious and costly disposal problems. This can be aggravated by the ability of sludge to adsorb specific organic compounds and even toxic heavy metals. However, biological systems have the advantage of microbial transformations of complex organics and possible adsorption of heavy metals by suitable microbes. Biological processes are still fairly unsophisticated and have great potential for combining various types of biological schemes for selective component removal. Aerobic Biological Systems. Aerobic biological treatment methods depend on micro- organisms grown in an oxygen-rich environment to oxidize organics to carbon dioxide, water, and cellular material. Considerable information on laboratory- and field-scale aerobic treatments has shown aerobic treatment to be reliable and cost-effective in producing a high-quality effluent. Start-up usually requires an acclimation period to allow the development of a competitive microbial community. Ammonia-nitrogen can successfully be removed, in order to prevent disposal problems. Problems normally associated with aerobic processes include foaming and poor solid – liquid separation. The conventional activated sludge process (ASP) is defined as a continuous treatment that uses a consortium of microbes suspended in the wastewater in an aeration tank to absorb, adsorb, and biodegrade the organic pollutants ((Fig. 1.1). Part of the organic composition will be completely oxidized to harmless endproducts and other inorganic substances to provide energy to sustain the microbial growth and the formation of biomass (flocs). The flocs are kept in suspension either by air blown into the bottom of the tank (diffused air system) or by mechanical aeration. The dissolved oxygen level in the aeration tank is critical and should preferably be 1 –2 mg/L and the tank must always be designed in terms of the aeration period and cell resi- dence time. The mixture flows from the aeration tank to a sedimentation tank where the activated sludge flocs form larger particles that settle as sludge. The biological aerobic metabolism mode is extremely efficient in terms of energy recovery, but results in large quantities of sludge being produced (0.6 kg dry sludge per kg of BOD5 removed). Some of the sludge is returned to the aeration tank but the rest must be processed and disposed of in an environmentally acceptable © 2006 by Taylor & Francis Group, LLC Treatment of Dairy Processing Wastewaters 11 Downloaded by [Universidade de Sao Paulo (USP) (CRUESP)] at 13:22 16 August 2016 Figure 1.1 Simplified illustrations of aerobic wastewater treatment processes: (a) aerobic filter, (b) activated sludge process (from Refs. 31, and 35– 37). manner, which is a major operating expense. Many variations of the ASP exist, but in all cases, the oxygen supplied during aeration is the major energy-consuming operation. With ASPs, problems generally encountered are bulking , foam production, precipitation of iron and carbonates, excessive sludge production, and a decrease in efficiency during winter periods. Many reports show that ASP has been used successfully to treat dairy industry wastes. Donkin and Russell found that reliable COD removals of over 90% and 65% reductions in total nitrogen could be obtained with a milk powder/butter wastewater. Phosphorus removals were less reliable and appeared to be sensitive to environmental changes. Aerobic filters such as conventional trickling or percolating filters (Fig. 1.1) are among the oldest biological treatment methods for producing high-quality final effluents. The carrier media (20 – 100 mm diameter) may consist of pumice, rock, gravel, or plastic pieces, which is populated by a very diverse microbial consortium. Wastewater from a storage tank is normally dosed over the medium and then trickles downward through a 2-m medium bed. The slimy microbial mass growing on the carrier medium absorbs the organic constituents of the wastewater and decomposes them aerobically. Sludge deposits require removal from time to time. Aerobic conditions are facilitated by the downward flow and natural convection currents resulting from temperature differences between the air and the added wastewater. Forced ventilation may be employed to enhance the decomposition, but the air must be deodorized by © 2006 by Taylor & Francis Group, LLC 12 Britz et al. passing through clarifying tanks. Conventional filters, with aerobic microbes growing on rock or gravel, are limited in depth to about 2 m, as deeper filters enhance anaerobic growth with subsequent odor problems. In contrast, filters with synthetic media can be fully aerobic up to about 8 m. The final effluent flows to a sedimentation or clarifying tank to remove sludge and solids from the carrier medium. It is generally recommended that organic loading for dairy wastewaters not exceed 0.28 –0.30 kg BOD/m3 and that recirculation be employed. A 92% BOD removal of a dairy wastewater was reported by Kessler , but since the BOD of the final effluent was still too high, it was further treated in an oxidation pond. Downloaded by [Universidade de Sao Paulo (USP) (CRUESP)] at 13:22 16 August 2016 An inherent problem is that trickling filters can be blocked by precipitated ferric hydroxide and carbonates, with concomitant reduction of microbial activity. In the case of overloading with dairy wastewater, the medium becomes blocked with heavy biological and fat films. Maris et al. reported that biological filters are not appropriate for the treatment of high-strength wastewaters, as filter blinding by organic deposition on the filter medium is generally found. The rotating biological contactors (RBC) design contains circular discs (Fig. 1.2) made of high-density plastic or other lightweight material. The discs, rotating at 1– 3 rpm, are placed on a horizontal shaft so that about 40 –60% of the disc surface protrudes out of the tank; this allows oxygen to be transferred from the atmosphere to the exposed films. A biofilm develops on the disc surface, which facilitates the oxidation of the organic components of the wastewater. When the biofilm sludge becomes too thick, it is torn off and removed in a sedimentation tank. Operation efficiency is based on the g BOD per m2 of disc surface per day. Rusten and his coworkers reported 85% COD removal efficiency with an organic loading rate (OLR) of 500 g COD/m3 hour while treating dairy wastewater. The RBC process offers several advantages over the activated sludge process for use in dairy wastewater treatment. The primary advantages are the low power input required, relative ease of operation and low maintenance. Furthermore, pumping, aeration, and wasting/recycle of solids are not required, leading to less operator attention. Operation for nitrogen removal is also relatively simple and routine maintenance involves only inspection and lubrication. The sequencing batch reactor (SBR) is a single-tank fill-and-draw unit that utilizes the same tank (Fig. 1.2) to aerate, settle, withdraw effluent, and recycle solids. After the tank is filled, the wastewater is mixed without aeration to allow metabolism of the fermentable compounds. This is followed by the aeration step, which enhances the oxidation and biomass formation. Sludge is then settled and the treated effluent is removed to complete the cycle. The SBR relies heavily on the site operator to adjust the duration of each phase to reflect fluctua- tions in the wastewater composition. The SBR is seen as a good option with low- flow applications and allows for wider wastewater strength variations. Eroglu et al. and Samkutty et al. reported the SBR to be a cost-effective primary and secondary treat- ment option to handle dairy plant wastewater with COD removals of 91 –97%. Torrijos et al. also demonstrated the efficiency of the SBR system for the treatment of wastewater from small cheese-making dairies with treatment levels of.97% being obtained at a loading rate of 0.50 kg COD/m3 day. In another study, Li and Zhang successfully operated an SBR at a hydraulic retention time (HRT) of 24 hours to treat dairy waste with a COD of 10 g/L. Removal efficiencies of 80% in COD, 63% in total solids, 66% in volatile solids, 75% Kjeldahl nitrogen, and 38% in total nitrogen, were obtained. In areas where land is available, lagoons/ponds/reed beds (Fig. 1.2) constitute one of the least expensive methods of biological degradation. With the exception of aerated ponds, no mechanical devices are used and flow normally occurs by gravity. As result of their simplicity and absence of a sludge recycle facility, lagoons are a favored method for effective wastewater treatment. However, the lack of a controlled environment slows the reaction times, resulting in © 2006 by Taylor & Francis Group, LLC Treatment of Dairy Processing Wastewaters 13 Downloaded by [Universidade de Sao Paulo (USP) (CRUESP)] at 13:22 16 August 2016 Figure 1.2 Simplified illustrations of aerobic wastewater treatment processes: (a) sequencing batch reactor, (b) rotating biological contactor, (c) treatment pond (from Refs. 35, 40, 42, 45, 47 – 49). long retention times of up to 60 days. Operators of sites in warmer climates may find the use of lagoons a more suitable and economical wastewater treatment option. However, the potential does exist for surface and groundwater pollution, bad odors, and insects that may become a nuisance. Aerated ponds are generally 0.5– 4.0 m deep. Evacuation on the site plus lining is a simple method of lagoon construction and requires relatively unskilled attention. Floating aerators may be used to allow oxygen and sunlight penetration. According to Bitton , aeration for 5 days at 208C in a pond normally gives a BOD removal of 85% of milk wastes. Facultative ponds are also commonly used for high-strength dairy wastes. Although © 2006 by Taylor & Francis Group, LLC 14 Britz et al. ponds/lagoons are simple to operate, they are the most complex of all biologically engineered degradation systems. In these systems, both aerobic and anaerobic metabolisms occur in addition to photosynthesis and sedimentation. Although most of the organic carbon is converted to microbial biomass, some is lost as CO2 or CH4. It is thus essential to remove sludge regularly to prevent buildup and clogging. The HRT in facultative ponds can vary between 5 and 50 days depending on climatic conditions. Reed-bed or wetland systems have also found widespread application. A design manual and operating guidelines were produced in 1990 [49,50]. Reed beds are designed to treat wastewaters by passing the latter through rhizomes of the common reed in a shallow bed of soil Downloaded by [Universidade de Sao Paulo (USP) (CRUESP)] at 13:22 16 August 2016 or gravel. The reeds introduce oxygen and as the wastewater percolates through it, aerobic microbial communities establish among the roots and degrade the contaminants. Nitrogen and phosphorus are thus removed directly by the reeds. However, reed beds are poor at removing ammonia, and with high concentrations of ammonia being toxic, this may be a limiting factor. The precipitation of large quantities of iron, manganese, and calcium within the reed beds will also affect rhizome growth and, in time, reduce the permeability of the bed. According to Robinson et al. , field studies in the UK have shown that reed beds have enormous potential and, in combination with aerobic systems, provide high effluent quality at reasonable cost. Anaerobic Biological Systems. Anaerobic digestion (AD) is a biological process per- formed by an active microbial consortium in the absence of exogenous electron acceptors. Up to 95% of the organic load in a waste stream can be converted to biogas (methane and carbon dioxide) and the remainder is utilized for cell growth and maintenance [51,52]. Anaerobic systems are generally seen as more economical for the biological stabilization of dairy wastes , as they do not have the high-energy requirements associated with aeration in aerobic systems. Anaerobic digestion also yields methane, which can be utilized as a heat or power source. Furthermore, less sludge is generated, thereby reducing problems associated with sludge disposal. Nutrient requirements (N and P) are much lower than for aerobic systems , pathogenic organisms are usually destroyed, and the final sludge has a high soil conditioning value if the concentration of heavy metals is low. The possibility of treating high COD dairy wastes without previous dilution, as required by aerobic systems, reduces space requirements and the associated costs. Bad odors are generally absent if the system is operated efficiently [51,54]. The disadvantages associated with anaerobic systems are the high capital cost, long start- up periods, strict control of operating conditions, greater sensitivity to variable loads and organic shocks, as well as toxic compounds. The operational temperature must be maintained at about 33 – 378C for efficient kinetics, because it is important to keep the pH at a value around 7, as a result of the sensitivity of the methanogenic population to low values. As ammonia- nitrogen is not removed in an anaerobic system, it is consequently discharged with the digester effluent, creating an oxygen demand in the receiving water. Complementary treatment to achieve acceptable discharge standards is also required. The anaerobic lagoon (anaerobic pond) (Fig. 1.3) is the simplest type of anaerobic digester. It consists of a pond, which is normally covered to exclude air and to prevent methane loss to the atmosphere. Lagoons are far easier to construct than vertical digester types, but the biggest drawback is the large surface area required. In New Zealand, dairy wastewater was treated at 358C in a lagoon (26,000 m3) covered with butyl rubber at an organic load of 40,000 kg COD per day, pH of 6.8 –7.2, and HRT of 1 –2 days. The organic loading rate (OLR) of 1.5 kg COD/m3 day was on the low side. The pond’s effluent was clarified and the settled biomass recycled through the substrate feed. The clarified effluent was then treated in an 18,000 m3 aerated lagoon. The efficiency of the total system reached a 99% reduction in COD. © 2006 by Taylor & Francis Group, LLC Treatment of Dairy Processing Wastewaters 15 Downloaded by [Universidade de Sao Paulo (USP) (CRUESP)] at 13:22 16 August 2016 Figure 1.3 Simplified illustrations of anaerobic wastewater treatment processes: (a) anaerobic filter digester, (b) fluidized-bed digester, (c) UASB digester, (d) anaerobic lagoon/pond (from Refs. 31, 35, 51, 58, 70). Completely stirred tank reactors (CSTR) are, next to lagoons, the simplest type of anaerobic digester (Fig. 1.4). According to Sahm , the OLR rate ranges from 1–4 kg organic dry matter m23 day21 and the digesters usually have capacities between 500 and 700 m3. These reactors are normally used for concentrated wastes, especially those where the polluting matter is present mainly as suspended solids and has COD values of higher than 30,000 mg/L. In the CSTR, there is no biomass retention; consequently, the HRT and sludge retention time (SRT) are not separated, necessitating long retention times that are dependent on the growth rate of the © 2006 by Taylor & Francis Group, LLC 16 Britz et al. Downloaded by [Universidade de Sao Paulo (USP) (CRUESP)] at 13:22 16 August 2016 Figure 1.4 Simplified illustrations of anaerobic wastewater treatment processes: (a) conventional digester, (b) Contact digester, (c) fixed-bed digester (from Refs. 31, 57, 58, 60, 64, 66, 79). slowest-growing bacteria involved in the digestion process. Ross found that the HRT of the conventional digesters is equal to the SRT, which can range from 15 –20 days. This type of digester has in the past been used by Lebrato et al. to treat cheese factory wastewater. While 90% COD removal was achieved, the digester could only be operated at a minimum HRT of 9.0 days, most probably due to biomass washout. The wastewater, consisting © 2006 by Taylor & Francis Group, LLC Treatment of Dairy Processing Wastewaters 17 of 80% washing water and 20% whey, had a COD of 17,000 mg/L. While the CSTR is very useful for laboratory studies, it is hardly a practical option for full-scale treatment due to the HRT limitation. The anaerobic contact process (Fig. 1.4) was developed in 1955. It is essentially an anaerobic activated sludge process that consists of a completely mixed anaerobic reactor followed by some form of biomass separator. The separated biomass is recycled to the reactor, thus reducing the retention time from the conventional 20 – 30 days to ,1.0 days. Because the bacteria are retained and recycled, this type of plant can treat medium-strength wastewater (200 – 20,000 mg/L COD) very efficiently at high OLRs. The organic loading rate can vary Downloaded by [Universidade de Sao Paulo (USP) (CRUESP)] at 13:22 16 August 2016 from 1 to 6 kg/m3 day COD with COD removal efficiencies of 80 – 95%. The treatment temperature ranges from 30 –408C. A major difficulty encountered with this process is the poor settling properties of the anaerobic biomass from the digester effluent. Dissolved air flotation and dissolved biogas flotation techniques have been attempted as alternative sludge separation techniques. Even though the contact digester is considered to be obsolete there are still many small dairies all over the world that use the system. The upflow anaerobic filter (Fig. 1.3) was developed by Young and McCarty in 1969 and is similar to the aerobic trickling filter process. The reactor is filled with inert support material such as gravel, rocks, coke, or plastic media and thus there is no need for biomass separation and sludge recycling. The anaerobic filter reactor can be operated either as a downflow or an upflow filter reactor with OLR ranging from 1 – 15 kg/m3 day COD and COD removal efficiencies of 75 –95%. The treatment temperature ranges from 20 to 358C with HRTs in the order of 0.2– 3 days. The main drawback of the upflow anaerobic filter is the potential risk of clogging by undegraded suspended solids, mineral precipitates or the bacterial biomass. Furthermore, their use is restricted to wastewaters with COD between 1000 and 10,000 mg/L. Bonastre and Paris listed 51 anaerobic filter applications of which five were used for pilot plants and three for full-scale dairy wastewater treatment. These filters were operated at HRTs between 12 and 48 hours, while COD removal ranged between 60 and 98%. The OLR varied between 1.7 and 20.0 kg COD/m3 day. The expanded bed and/or fluidized-bed digesters (Fig. 1.3) are designed so that wastewaters pass upwards through a bed of suspended media, to which the bacteria attach. The carrier medium is constantly kept in suspension by powerful recirculation of the liquid phase. The carrier media include plastic granules, sand particles, glass beads, clay particles, and activated charcoal fragments. Factors that contribute to the effectiveness of the fluidized-bed process include: (a) maximum contact between the liquid and the fine particles carrying the bacteria; (b) problems of channeling, plugging, and gas hold-up commonly encountered in packed-beds are avoided; and (c) the ability to control and optimize the biological film thickness. OLRs of 1– 20 kg/m3 day COD can be achieved with COD removal efficiencies of 80– 87% at treatment temperatures from 20 to 358C. Toldrá et al. used the process to treat dairy wastewater with a COD of only 200 –500 mg/L at an HRT of 8.0 hours with COD removal of 80%. Bearing in mind the wide variations found between different dairy effluents, it can be deduced that this particular dairy effluent is at the bottom end of the scale in terms of its COD concentration and organic load. The dairy wastewater was probably produced by a dairy with very good product-lo