CAIE Physics IGCSE Topic 3: Waves Summary Notes PDF
Document Details
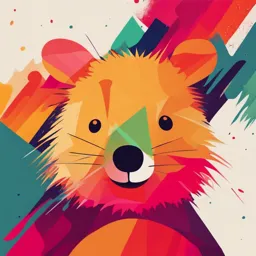
Uploaded by PraisingMulberryTree4656
CAIE
Tags
Summary
These are summary notes for CAIE Physics IGCSE Topic 3: Waves. The document covers general wave properties, including amplitude, wavelength and frequency. It also discusses types of waves, reflection, refraction and diffraction. The notes include diagrams and practical examples.
Full Transcript
CAIE Physics IGCSE Topic 3: Waves Summary Notes This work by PMT Education is licensed under https://bit.ly/pmt-cc https://bit.ly/pmt-edu-cc CC BY-NC-ND 4.0...
CAIE Physics IGCSE Topic 3: Waves Summary Notes This work by PMT Education is licensed under https://bit.ly/pmt-cc https://bit.ly/pmt-edu-cc CC BY-NC-ND 4.0 https://bit.ly/pmt-cc https://bit.ly/pmt-edu https://bit.ly/pmt-cc 3.1 General properties of waves Waves transfer energy without transferring matter; particles oscillate about a fixed point. Amplitude – the distance from the equilibrium position to the maximum displacement Wavelength – the distance between a point on one wave and the same point on the next wave Frequency – the number of waves that pass a single point per second Speed – the distance travelled by a wave each second Wave motion refers to the transfer of energy from one point to another without the transfer of matter, typically involving oscillations or vibrations. Speed is related to frequency and wavelength by: 𝑠𝑝𝑒𝑒𝑑 = 𝑓𝑟𝑒𝑞𝑢𝑒𝑛𝑐𝑦 × 𝑤𝑎𝑣𝑒𝑙𝑒𝑛𝑔𝑡ℎ 𝑣 = 𝑓λ Types of waves: Transverse waves ○ Has peaks and troughs ○ Vibrations are at right angles to the direction of travel ○ An example is light Longitudinal waves ○ Consists of compressions (particles pushed together) and rarefactions (particles moved apart) ○ Vibrations are in the same direction as the direction of travel ○ An example is sound A wavefront is a surface containing points affected in the same way by a wave at a given time such as crests or troughs. Reflection: Waves reflect off smooth, plane surfaces rather than getting absorbed ○ Angle of incidence = angle of reflection Rough surfaces scatter the light in all directions, so they appear matte and unreflective Frequency, wavelength, and speed are all unchanged Refraction: The speed of a wave changes when it enters a new medium If the wave enters a more optically dense medium, its speed decreases and it bends towards the normal If the wave enters a less optically dense medium, its speed increases and it bends away from the normal In all cases, the frequency stays the same but the wavelength changes. Diffraction: Waves spread out when they go around the sides of an obstacle or through a gap The narrower the gap or the greater the wavelength, the more the diffraction Frequency, wavelength, and speed are all unchanged https://bit.ly/pmt-cc https://bit.ly/pmt-edu https://bit.ly/pmt-cc A ripple tank is a shallow tank of water used to study wave motion. It is equipped with a vibrating paddle to generate waves and often has a lamp above to project the wave patterns onto a screen or surface below. Reflection at a Plane Surface: Setup: Place a straight barrier (e.g., a ruler) at an angle in the ripple tank. Observation: Waves strike the barrier and bounce off, obeying the law of reflection: ○ Angle of incidence = Angle of reflection. The reflected waves can be observed moving away from the barrier at the same angle they approached. Refraction Due to a Change in Depth: Setup: Place a transparent shallow block in the ripple tank to create a region with different water depth. Observation: ○ Waves slow down and have shorter wavelengths in the shallow region. ○ The direction of the waves bends (refraction) as they pass from deeper to shallower water or vice versa. This illustrates how wave speed changes with depth, causing refraction. Diffraction Due to a Gap: Setup: Place two barriers with a small gap between them in the tank. Observation: ○ When waves pass through the gap, they spread out into semicircles. ○ The amount of diffraction increases as the gap size decreases relative to the wavelength. Diffraction Due to an Edge: Setup: Place a single barrier or object in the path of the waves. Observation: ○ As waves encounter the edge of the barrier, they bend around it, spreading into the region behind the barrier. ○ This illustrates how diffraction occurs even without a gap, showing the wave's ability to bend around obstacles. ○ Waves with longer wavelengths (e.g., sound waves) diffract more significantly around edges. ○ This is because longer wavelengths are more comparable in size to the edge, allowing the wave to bend around it effectively. https://bit.ly/pmt-cc https://bit.ly/pmt-edu https://bit.ly/pmt-cc 3.2 Light 3.2.1 Reflection of light Reflection of light is the process by which light rays bounce off a surface and change direction, staying in the same medium. The angle of incidence (angle between the incoming ray and the normal) equals the angle of reflection (angle between the reflected ray and the normal). Definitions: Normal: A line perpendicular to the reflecting surface at the point where the light ray strikes. Angle of Incidence (i): The angle between the incident ray and the normal. Angle of Reflection (r): The angle between the reflected ray and the normal. Formation of an Optical Image by a Plane Mirror: Characteristics of the image: ○ Same size as the object. ○ Same distance behind the mirror as the object is in front. ○ Virtual: The image cannot be projected onto a screen. ○ Laterally inverted: Left and right are reversed. Law of Reflection: Angle of Incidence = Angle of Reflection (i = r). This law applies to all reflective surfaces. Constructions, Measurements, and Calculations: Ray Diagrams for Plane Mirrors: ○ Draw the object and the mirror. ○ Construct incident rays from the object to the mirror, ensuring the angles with the normal are equal for reflection. ○ Extend the reflected rays behind the mirror to locate the virtual image. Measurements: ○ Use a protractor to verify i = r. Calculations: ○ Determine image location and distances using geometry (e.g., image distance = object distance from the mirror). https://bit.ly/pmt-cc https://bit.ly/pmt-edu https://bit.ly/pmt-cc 3.2.2 Refraction of light Refraction can be shown when light is passed through a glass slab at an angle to its normal (the imaginary line 90º to the surface of the material). When light enters a more optically dense medium, the angle of incidence (the angle between the incident ray and the normal) is greater than the angle of refraction (the angle between the refracted ray and the normal). The opposite is true when light enters a less optically dense medium. The refractive index n of a medium is defined as the ratio between the speed of light 𝑠𝑝𝑒𝑒𝑑 𝑜𝑓 𝑙𝑖𝑔ℎ𝑡 𝑖𝑛 𝑣𝑎𝑐𝑢𝑢𝑚 in a vacuum and the speed of light in the medium:𝑛 = 𝑠𝑝𝑒𝑒𝑑 𝑜𝑓 𝑙𝑖𝑔ℎ𝑡 𝑖𝑛 𝑡ℎ𝑒 𝑚𝑒𝑑𝑖𝑢𝑚 Snell's law relates the angle of incidence and the angle of refraction to the refractive 𝑠𝑖𝑛 𝑖 index by: 𝑛 = 𝑠𝑖𝑛 𝑟 where i is the angle of incidence and r is the angle of refraction. Total internal reflection: At a certain angle of incidence called the critical angle, the light will travel along the boundary between the two media. Total internal reflection occurs when the angle of incidence is greater than the critical angle and the light reflects back into the medium. For total internal reflection to occur, the light must also be travelling from a more optically dense medium into a less optically dense medium (most common example is glass to air). The critical angle can be related to the refractive index by: 1 𝑛= 𝑠𝑖𝑛 𝑐 Optical fibres: An optical fibre is a long thin rod of glass surrounded by cladding which uses total internal reflection to transfer information by light, even when bent. Extensive use in medicine (endoscopes, inside-body flexible cameras) and communications (high speed data transfer). https://bit.ly/pmt-cc https://bit.ly/pmt-edu https://bit.ly/pmt-cc 3.2.3 Thin Lenses Converging lens: A converging lens is a transparent block which brings light rays together at a point called the principal focus by utilising refraction. The focal length is the distance between the centre of the lens and the principal focus. The image formed by a converging lens can be either real or virtual. ○ Real images are formed when the distance of the object from the centre of the lens is greater than the focal length. They are images where light actually converges to a position and can be projected onto a screen. ○ Virtual images are formed when the distance of the object from the centre of the lens is smaller than the focal length. They are images where light only appears to have converged and they cannot be projected onto a screen. You can draw ray diagrams for real images (shown on the left below) and virtual images (shown on the right below). The image formed is enlarged/same size/diminished and upright/inverted. ○ The image on the left above is diminished and inverted. ○ The image on the right above is enlarged and upright. Converging lenses are used in magnifying glasses and binoculars (to enlarge the image). Converging lenses bring light closer together, aiding long-sighted eyes (Hyperopia). Diverging lenses spread light apart, aiding short-sighted eyes (Myopia). 3.2.4 Dispersion of light When white light is passed through a glass prism, it splits up into its constituent colours. This happens because the different colours travel at different speeds in the glass, so they refract by different amounts. The seven colours in order of decreasing wavelength are red, orange, yellow, green, blue, indigo and violet (ROYGBIV). The greater the wavelength, the slower the speed in glass and the greater the refractive index. Light of a single frequency is described as monochromatic. https://bit.ly/pmt-cc https://bit.ly/pmt-edu https://bit.ly/pmt-cc 3.3 Electromagnetic spectrum Properties of electromagnetic waves: Transverse waves Do not need a medium All electromagnetic waves travel with the same high speed of 3.0 x 108 ms-1 in a vacuum and approximately the same speed in air. You need to learn the main groups of the electromagnetic spectrum in order of wavelength. As speed is constant for all electromagnetic waves, as wavelength decreases, frequency must increase. The higher the frequency of an EM wave, the greater its energy. Uses of electromagnetic waves: Radio waves are used for radio and television communications. They have a long wavelength and are reflected by the ionosphere. Microwaves are used for satellite communication and in microwave oven. They pass through the ionosphere and penetrate deep into food. Infrared radiation is used in remote controllers and infrared cameras. Visible light is used in fibre optics. Ultraviolet light is used in tanning beds. X-rays are used in medical imaging and in security as they can penetrate material easily. Gamma radiation is used in medical treatment due to its high energy. Hazards: Too much exposure to ultraviolet light skin increases the risk of skin cancer. ○ Sun cream prevents over-exposure in summer. X-rays and gamma rays are ionising radiation that can cause mutations leading to cancer. ○ Exposure to these kinds of radiation should be minimised. Microwaves can cause internal heating of body tissues. Infrared radiation can cause skin burns. https://bit.ly/pmt-cc https://bit.ly/pmt-edu https://bit.ly/pmt-cc Communication with Artificial Satellites: Microwaves are the primary method for communication with satellites because they can penetrate the Earth’s atmosphere. Satellite phones: ○ Low orbit satellites: Used for some satellite phones, requiring multiple satellites to provide coverage. ○ Geostationary satellites: Used for direct broadcast satellite TV and some satellite phones, remaining fixed relative to a point on Earth. Speed of Electromagnetic Waves: Electromagnetic waves travel at 3.0×108 m/s in a vacuum and approximately the same in air. Communication Systems and Electromagnetic Radiation: Mobile Phones and Wireless Internet: ○ Use microwaves, which can penetrate walls and require only short aerials for transmission and reception. Bluetooth: ○ Uses low-energy radio waves or microwaves, which can pass through walls but weaken when doing so. Optical Fibres: ○ Use visible light or infrared for cable TV and high-speed broadband. ○ Glass is transparent to these wavelengths, which can carry high data rates efficiently. Digital vs Analogue Signals: Analogue Signal: Continuously varying signals that represent data (e.g., sound waves). Digital Signal: Consists of discrete binary values (0s and 1s) for transmitting data. Sound Transmission: Sound can be transmitted as either: ○ An analogue signal (direct representation of sound waves). ○ A digital signal (converted into binary for processing and transmission). Benefits of Digital Signaling: Higher Data Transmission Rates: Digital signals can carry more information per second. Accurate Signal Regeneration: Digital signals can be regenerated accurately over long distances, reducing noise and improving signal quality. Increased Range: Better performance in long-distance communication due to less degradation compared to analogue signals. https://bit.ly/pmt-cc https://bit.ly/pmt-edu https://bit.ly/pmt-cc 3.4 Sound Sound waves are longitudinal waves created by vibrating sources. A medium is needed to transmit sound waves (such as air). The greater the amplitude of a sound wave, the louder it is. The greater the frequency of a sound wave, the higher its pitch. To measure the speed of sound in air, you can make a noise at a known, large distance from a solid wall and record the time for the echo (reflected sound) to be heard, then use speed = distance/time, taking into account the fact that the sound had to go there and back. The speed of sound in air is 343 ms-1, the speed of sound in water is 1493 ms-1, and the speed of sound in steel is 5130 ms-1. The range of audible frequencies for a healthy human ear is 20 Hz to 20000 Hz. Ultrasound is sound with a frequency greater than 20000 Hz: When ultrasound reaches a boundary between two media it is partially reflected back. The remainder of the waves continue to pass through. A transceiver can emit ultrasound and record the reflected waves to find the distance of things below the surface. Ultrasound is used for things such as SONAR and for medical imaging without using ionising radiation. Compression and rarefactions are variations of pressure within a sound wave. Regions in a sound wave where particles are pushed closer together, creating areas of high pressure. Regions where particles are spread further apart, creating areas of low pressure. Together, compressions and rarefactions form longitudinal waves, the typical motion of sound waves in a medium. Speed of Sound in Different Media: Sound travels faster in solids than in liquids and faster in liquids than in gases: ○ Solids: Strong intermolecular forces and closely packed particles allow efficient energy transfer. ○ Liquids: Particles are less tightly packed than in solids, leading to slower sound propagation. ○ Gases: Particles are far apart, resulting in the slowest sound transmission. Sonar can be used to detect flaws in materials (e.g., cracks in metal or plastic) without damaging them. Ultrasound waves reflect from boundaries between materials or defects, identifying issues. Sonar can also be used in medical sca nning to image soft tissues (e.g., organs or a fetus during pregnancy). Ultrasound waves reflect at boundaries between different tissues, forming an image. Sonar can also be used to measure water depth or locate underwater objects (e.g., submarines, fish). A pulse of ultrasound is sent underwater and the time taken for the echo to return is measured. Depth Calculation: Depth or Distance = (Speed of Sound x Time) / 2 https://bit.ly/pmt-cc https://bit.ly/pmt-edu https://bit.ly/pmt-cc