Structure and Function of the Respiratory System PDF
Document Details
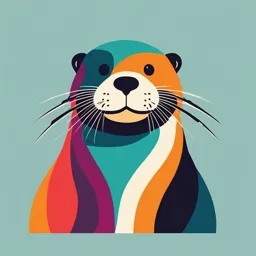
Uploaded by InterestingAshcanSchool
Tags
Related
Summary
This document provides a detailed overview on the structure and function of the respiratory system, including the different components, and their roles in respiration. It covers various aspects, with particular attention to the anatomical organization of the respiratory system. The structure of the lungs, conducting airways, and the gas exchange processes within the alveoli are presented.
Full Transcript
CHAPTER 29: STRUCTURE AND FUNCTION OF THE RESPIRATORY SYSTEM Copyright Copyright © 2015 Wolters © 2019 Wolters Kluwer Health Kluwer| Lippincott...
CHAPTER 29: STRUCTURE AND FUNCTION OF THE RESPIRATORY SYSTEM Copyright Copyright © 2015 Wolters © 2019 Wolters Kluwer Health Kluwer| Lippincott All RightsWilliams Reserved & Wilkins The movement of air between the Ventilation atmosphere and the respiratory portion of the lungs COMPONENTS OF THE Perfusion The flow of blood through the lungs RESPIRATORY SYSTEM Diffusion The transfer of gases between the air- filled spaces in the lungs and the blood Copyright © 2019 Wolters Kluwer All Rights Reserved STRUCTURAL ORGANIZATION OF THE RESPIRATORY SYSTEM Consists of the air passages and the lungs Divided into two parts by function: Conducting airways: through which air moves as it passes between the atmosphere and the lungs Respiratory tissues of the lungs: where gas exchange takes place Copyright © 2019 Wolters Kluwer All Rights Reserved STRUCTURE OF THE LUNGS Soft, spongy, cone-shaped organs located side by side in the chest cavity Separated from each other by the mediastinum and its contents Divided into lobes (three in the right lung, two in the left) Apex: upper part of the lung; lies against the top of the thoracic cavity Base: lower part of the lung; lies against the diaphragm Copyright © 2019 Wolters Kluwer All Rights Reserved STRUCTURE AND FUNCTION OF THE LARYNX Structure Functions Connects the oropharynx Helping to produce speech with the trachea Protecting the lungs from Located in a strategic substances other than air position between the upper airways and the lungs Copyright © 2019 Wolters Kluwer All Rights Reserved QUESTION #1 Which of the following is directly responsible for gas exchange? A. Trachea B. Bronchi C. Bronchial circulation D. Pulmonary circulation E. Respiratory membrane Copyright © 2019 Wolters Kluwer All Rights Reserved ANSWER TO QUESTION #1 E. Respiratory membrane Rationale: The respiratory membrane is the anatomical site of gas exchange in the lungs. It is located in the alveoli. Copyright © 2019 Wolters Kluwer All Rights Reserved VENTILATION AND GAS EXCHANGE Ventilation The movement of gases into and out of the lungs Inspiration Air is drawn into the lungs as the respiratory muscles expand the chest cavity. Expiration Air moves out of the lungs as the chest muscles recoil, and the chest cavity becomes smaller. Copyright © 2019 Wolters Kluwer All Rights Reserved Depends on the conducting airways: Nasopharynx and oropharynx Larynx Tracheobronchial tree VENTILATION Function: Move air out of the lungs but does not participate in gas exchange Copyright © 2019 Wolters Kluwer All Rights Reserved STRUCTURES OF THE AIRWAYS Conducting Nasal passages Mouth and pharynx Larynx Trachea Bronchi Bronchioles Mucociliary blanket Respiratory tissues Alveolar bundle Respiratory membrane Copyright © 2019 Wolters Kluwer All Rights Reserved COMPOSITION OF THE ALVEOLAR STRUCTURES Type I Alveolar Cells Flat squamous epithelial cells across which gas exchange takes place Type II Alveolar Cells Produce surfactant, a lipoprotein substance that decreases the surface tension in the alveoli and allows for greater ease of lung inflation Copyright © 2019 Wolters Kluwer All Rights Reserved LUNG CIRCULATION Pulmonary Circulation Arises from the pulmonary artery Provides for the gas exchange function of the lungs Bronchial Circulation Arises from the thoracic aorta Supplies the lungs and other lung structures with oxygen Distributes blood to the conducting airways Warms and humidifies incoming air Copyright © 2019 Wolters Kluwer All Rights Reserved LUNG INNERVATION The sympathetic and parasympathetic divisions of the autonomic nervous system innervate the lung Parasympathetic stimulation Vagus nerve Responsible for the slightly constricted smooth muscle tone in the normal resting lung Airway constriction Increased glandular secretion Greater in the large airways and diminishes toward the smaller airways Sympathetic stimulation Paravertebral sympathetic ganglia Airway relaxation Blood vessel constriction Inhibition of glandular secretion Copyright © 2019 Wolters Kluwer All Rights Reserved LUNG INNERVATION There is no voluntary motor innervation of the lung, nor are there pain fibers Pain fibers are found only in the pleura Copyright © 2019 Wolters Kluwer All Rights Reserved PLEURA Thin, transparent, double-layered serous membrane that lines the thoracic cavity and encases the lungs Parietal layer (outer) Lines the pulmonary cavities Adheres to the thoracic wall, the mediastinum, and the diaphragm Visceral pleura (inner) Closely covers the lung Adherent to all its surfaces Continuous with the parietal pleura at the hilum of the lung, where the major bronchus and pulmonary vessels enter and leave the lung A thin film of serous fluid separates the two pleural layers, allowing them to glide over each other The pleural cavity is a potential space in which serous fluid or inflammatory exudate can accumulate Copyright © 2019 Wolters Kluwer All Rights Reserved PLEURAL EFFUSION Copyright © 2019 Wolters Kluwer All Rights Reserved PROPERTIES OF GASES Respiratory pressures Intrapulmonary pressure or alveolar pressure Pressure inside the airways and alveoli Gases in this area are in communication with atmospheric pressure When the glottis is open and air is not moving into or out of the lungs, as occurs just before inspiration or expiration, the intrapulmonary pressure is zero or equal to atmospheric pressure Intrapleural pressure Pressure in the pleural cavity Always negative in relation to alveolar pressure Intrathoracic Pressure Pressure in the thoracic cavity Atmospheric pressure Partial pressures Copyright © 2019 Wolters Kluwer All Rights Reserved LUNG COMPLIANCE Lung Compliance Lung compliance refers to the ease with which the lungs can be inflated C = ΔV/ΔP The change in lung volume (ΔV) that can be accomplished with a given change in respiratory pressure (ΔP) Compliance can be appreciated by comparing the ease of blowing up a stiff and resistant new balloon that is noncompliant with a compliant one that has been previously blown up and is easy to inflate. It takes more pressure to move the same amount of air into a noncompliant lung than it does into a compliant one. Copyright © 2019 Wolters Kluwer All Rights Reserved LUNG COMPLIANCE Lung compliance is determined by: Elastin (increase lung inflation)and collagen fibers (resist stretching)of the lung Water content Surface tension Compliance of the thoracic or chest cage It is diminished by conditions that: Reduce the natural elasticity of the lung Block the bronchi or smaller airways Increase the surface tension in the alveoli Impair the flexibility of the thoracic cage Copyright © 2019 Wolters Kluwer All Rights Reserved AIRWAY RESISTANCE Airway Airflow The volume of air that moves into and out of the air exchange portion of the lungs Directly related to the pressure difference between the lungs and the atmosphere Inversely related to the resistance the air encounters as it moves through the airways Copyright © 2019 Wolters Kluwer All Rights Reserved AIRWAY RESISTANCE Airway resistance is greatly affected by lung volume Expiration The lungs and airways expand during inspiration (lower resistance), while they deflate during expiration (higher resistance) During inspiration, the lungs and airways expand, which increases the airway diameter and alveolar diameter. One of the reasons why people with conditions that increase airway resistance, such as asthma (reactive airway), usually have less difficulty during inspiration than expiration. Inspiration During expiration, the lungs and airways deflate, which narrows the airways and increases airway resistance. In emphysema, the elastic tissue in the lungs that keeps the small airways open can be destroyed; this can cause airway collapse during expiration, and further increase airway resistance. Copyright © 2019 Wolters Kluwer All Rights Reserved AIRWAY RESISTANCE Airway resistance is also affected by the bronchial smooth muscle tone that controls airway diameter The smooth muscles in the airway, from the trachea down to the terminal bronchioles, are under autonomic nervous system control Stimulation of the parasympathetic nervous system causes bronchial constriction as well as increased mucus secretion Sympathetic stimulation has the opposite effect Pulling It Together: In asthma, there is increased stimulation of the parasympathetic nervous system secondary to, for example, environmental triggers (increased vagal responsiveness) The pathophysiology of asthma is increased bronchial constriction and mucus secretion Anticholinergics, a class of medications commonly used in asthma treatment, opposes the parasympathetic response, thus reducing symptoms Copyright © 2019 Wolters Kluwer All Rights Reserved LUNG VOLUMES Spirometer: instrument used to Tidal Volume (TV) measure dynamic lung function Amount of air that moves into and out of the lungs during a normal breath Inspiratory Reserve Volume (IRV) The maximum amount of air that can be inspired in excess of the normal TV Expiratory Reserve Volume (ERV) Maximum amount of air that can be exhaled in excess of the normal TV Residual Volume (RV) The air that remains in the lungs after forced respiration (cannot be measured by a spirometer; this air cannot be expressed from the lungs) Copyright © 2019 Wolters Kluwer All Rights Reserved LUNG CAPACITIES IRV + TV + ERV Vital capacity The amount of air that can be exhaled from the point of maximal inspiration TV + IRV Inspiratory The amount of air a person can breathe in capacity beginning at the normal expiratory level and distending the lungs to the maximal amount Functional RV + ERV The volume of air that remains in the lungs at residual capacity the end of normal expiration Total lung Sum of all the volumes in the lungs capacity: Copyright © 2019 Wolters Kluwer All Rights Reserved LUNG CAPACITIES Copyright © 2019 Wolters Kluwer All Rights Reserved PULMONARY FUNCTION STUDIES Pulmonary function is measured for various clinical purposes: Diagnosis of respiratory disease, preoperative surgical and anesthetic risk evaluation, symptom & disability evaluation for legal or insurance purposes Evaluating dyspnea, cough, wheezing, and abnormal radiologic or laboratory findings Copyright © 2019 Wolters Kluwer All Rights Reserved PULMONARY FUNCTION STUDIES Maximum Voluntary Ventilation The volume of air a person can move into and out of the lungs during maximum effort lasting for 12 to 15 seconds. Forced Vital Capacity (FVC) Involves full inspiration to total lung capacity, followed by forceful maximal expiration Forced Expiratory Volume (FEV) The expiratory volume achieved in a given time period Forced Inspiratory Vital Flow (FIF) The respiratory response during rapid maximal inspiration Copyright © 2019 Wolters Kluwer All Rights Reserved QUESTION #2 Which of the following comprises the vital capacity? A. IRV + ERV B. VT + ERV C. VT + IRV + ERV D. VT + IRV – residual volume Copyright © 2019 Wolters Kluwer All Rights Reserved ANSWER TO QUESTION #2 C. VT + IRV + ERV Rationale: VT + IRV + ERV are the basic components of the vital capacity. Copyright © 2019 Wolters Kluwer All Rights Reserved PROCESSES OF PULMONARY GAS EXCHANGE—VENTILATION The primary functions of the lungs are: Oxygenation of the blood Removal of carbon dioxide Ventilation: the flow of gases into and out of the alveoli of the lungs Pulmonary ventilation: the total exchange of gases between the atmosphere and the lungs Alveolar ventilation: the exchange of gases within the gas exchange portion of the lungs Copyright © 2019 Wolters Kluwer All Rights Reserved DEAD SPACE Dead space refers to the air that is moved with each breath but does not participate in gas exchange. Anatomic Dead Space Air that is contained in the conducting airways Constitutes air contained in the nose, pharynx, trachea, and bronchi Approximately 150-200ml Alveolar Dead Space Air that is contained in the respiratory portion of the lung Alveoli that are ventilated but deprived of blood flow, and do not contribute to gas exchange Approximately 5-10ml Physiologic Dead Space The anatomic dead space plus alveolar dead space Copyright © 2019 Wolters Kluwer All Rights Reserved PROCESSES OF PULMONARY GAS EXCHANGE—PERFUSION Copyright © 2019 Wolters Kluwer All Rights Reserved PROCESSES OF PULMONARY GAS EXCHANGE—PERFUSION Primary functions of the pulmonary circulation: Perfuse or provide blood flow to the gas exchange portion of the lung Facilitate gas exchange The pulmonary circulation serves several other important functions: Filters all the blood that moves from the right to the left side of the circulation Removes most of the thromboemboli that might form Serves as a reservoir of blood for the left side of the heart Copyright © 2019 Wolters Kluwer All Rights Reserved PROCESSES OF PULMONARY GAS EXCHANGE—PERFUSION The movement of blood through the pulmonary capillary bed requires that the mean pulmonary arterial pressure be greater than the mean pulmonary venous pressure Pulling It Together: Pulmonary venous pressure increases in left-sided heart failure, allowing blood to accumulate in the pulmonary capillary bed This causes pulmonary edema you may see in left-sided congestive heart failure Copyright © 2019 Wolters Kluwer All Rights Reserved HYPOXIA-INDUCED VASOCONSTRICTION Blood vessels in the pulmonary circulation are highly sensitive to alveolar O2 levels and undergo marked vasoconstriction when exposed to hypoxia When alveolar O2 levels drop below 60 mm Hg, marked vasoconstriction may occur At very low O2 levels, the local flow may be almost abolished In regional hypoxia (atelectasis), vasoconstriction is localized to a specific region of the lung. In this case, vasoconstriction has the effect of directing blood flow away from the hypoxic regions of the lungs. When alveolar hypoxia no longer exists, blood flow is restored. Generalized hypoxia (high altitudes; people with chronic hypoxia due to lung disease) causes vasoconstriction throughout the lung Copyright © 2019 Wolters Kluwer All Rights Reserved HYPOXIA-INDUCED VASOCONSTRICTION Pulling It Together: In lung diseases that cause chronic hypoxia, pulmonary hypertension (increase in pulmonary vascular resistance secondary to lung disease) and increased workload on the right heart can occur This can cause cor pulmonale, an alteration in the structure/function of the right heart caused by lung disease In patients with COPD, cor pulmonale is common Copyright © 2019 Wolters Kluwer All Rights Reserved SHUNTS Shunt refers to blood that moves from the right to the left side of the circulation without being oxygenated. Anatomic Shunt Typically secondary to an anatomical abnormality Blood moves from the venous to the arterial side of the circulation without going through the lungs Physiologic Shunt When blood flows to unventilated or poorly ventilated alveoli, even though there is not an anatomical anomaly Pneumonia, acute respiratory distress syndrome, pleural effusions Pulling It Together: Hypoxemia occurs in disease states such as pneumonia and pleural effusion because there is blockage of the gas exchange at the alveolar level The blood that leaves these alveolar capillaries are not fully oxygenated, leading Copyright to hypoxemia © 2019 Wolters Kluwer All Rights Reserved MATCHING VENTILATION AND PERFUSION Gas exchange properties of the lung depend on matching ventilation and perfusion, ensuring that equal exchange of gases occurs between the alveoli and the blood in pulmonary capillaries Copyright © 2019 Wolters Kluwer All Rights Reserved MATCHING VENTILATION AND PERFUSION Two factors interfere with the matching of ventilation and perfusion: Dead air space With dead air space, (right), there is ventilation without perfusion, therefore, oxygen cannot enter the bloodstream Blood flow without oxygen Results in a high ventilation–perfusion ratio Occurs in conditions that impair blood flow to the lung (pulmonary embolism) Shunt With shunt, (left), there is perfusion without ventilation, therefore blood flow is occurring without adequate oxygenation Oxygen without blood flow Results in a low ventilation–perfusion ratio Occurs in conditions of airway obstruction (atelectasis) Copyright © 2019 Wolters Kluwer All Rights Reserved PROCESSES OF PULMONARY GAS EXCHANGE—DIFFUSION Diffusion Occurs in the respiratory portions of the lung Refers to the movement of gases across the alveolar-capillary membrane Copyright © 2019 Wolters Kluwer All Rights Reserved PROCESSES OF PULMONARY GAS EXCHANGE—DIFFUSION Several factors influence the diffusion of gases in the lung: Increases Diffusion Decreases Diffusion Administration of high concentrations Diseases that destroy lung of oxygen tissue/increase the thickness of the Increases the difference in partial alveolar–capillary membrane pressure between the two sides of the Adversely influence the diffusing membrane; increases the diffusion of capacity of the lungs the gas Removal of one lung Reduces the diffusing capacity by ½ Thickness of the alveolar–capillary membrane/distance for diffusion Pulmonary edema or pneumonia The characteristics of the gas and its molecular weight and solubility constitute the diffusion coefficient and determine how rapidly a gas diffuses through the respiratory membranes. For example, carbon dioxide diffuses 20 times more rapidly than oxygen because of its greater solubility in the respiratory membranes Copyright © 2019 Wolters Kluwer All Rights Reserved OXYGEN AND CARBON DIOXIDE TRANSPORT Although the lungs are responsible for the exchange of gases with the external environment, the blood transports these gases between the lungs and body tissues In the clinical setting, blood gas measurements are used to determine the partial pressure of oxygen (PO2) and carbon dioxide (PCO2), which are the same or nearly the same as the partial pressures in the alveoli Copyright © 2019 Wolters Kluwer All Rights Reserved OXYGEN AND CARBON DIOXIDE TRANSPORT Oxygen PO2 of arterial blood normally is above 80 mm Hg Oxygen Transport In chemical combination with hemoglobin (carries 98% to 99% of O2) In dissolved state (small amount) Hemoglobin Transport Oxyhemoglobin: hemoglobin bounded with oxygen In the lungs, oxygenalveolar–capillary membraneplasma red blood cell: RAPID process Hemoglobin is approximately 95-97% saturated by O2 as it moves out of the left heart; drops to 75% as it mixes with venous blood as it returns to right heart Carbon Dioxide PCO2 is in the range of 35 to 45 mm Hg Carbon Dioxide Transport Dissolved in carbon dioxide (10%) Attached to hemoglobin (30%) Bicarbonate (60%) The acid–base balance is influenced by the amount of dissolved carbon dioxide and the bicarbonate level in the blood. Copyright © 2019 Wolters Kluwer All Rights Reserved Copyright © 2019 Wolters Kluwer All Rights Reserved CONTROL OF BREATHING Respiratory Center Pons and medulla Two bilateral aggregates of respiratory neurons Dorsal: Concerned primarily with inspiration Control the activity of the phrenic nerves that innervate the diaphragm Drive the ventral group of respiratory neurons Integrates sensory input from the lungs and airways into the ventilatory response Ventral: Contains inspiratory and expiratory neurons Controls the spinal motor neurons of the intercostal and abdominal muscles Copyright © 2019 Wolters Kluwer All Rights Reserved CONTROL OF BREATHING Pacemaker Center Apneustic center Excitatory effect on inspiration, tending to prolong inspiration Pneumotaxic center Switches inspiration off, assisting in the control of respiratory rate and inspiratory volume Brain injuries that damage the connection between the pneumotaxic and apneustic centers result in an irregular breathing pattern that consists of prolonged inspiratory gasps interrupted by expiratory efforts: Apneustic breathing Copyright © 2019 Wolters Kluwer All Rights Reserved CONTROL OF BREATHING Automatic Regulation Of Ventilation Controlled by input from two types of sensors or receptors, chemoreceptors and lung receptors Chemoreceptors: Monitor blood levels of oxygen, carbon dioxide Adjust ventilation to meet the changing metabolic needs of the body Copyright © 2019 Wolters Kluwer All Rights Reserved CONTROL OF BREATHING Chemoreceptor Central Peripheral Located in chemosensitive Located in the carotid and aortic regions near the respiratory bodies, which are found at the center in the medulla bifurcation of the common carotid arteries and in the arch of the aorta, respectively Senses changes in PCO2 Monitors arterial O2 levels If elevated, increases ventilation Exert little control over ventilation until the PO2 has dropped below 60 mm Hg Declines if hypercapnia remains Hypoxia is the main stimulus for ventilation in people with chronically elevated levels of carbon dioxide Copyright © 2019 Wolters Kluwer All Rights Reserved CONTROL OF BREATHING Automatic Regulation Of Ventilation Lung receptors: Monitor the status of breathing in terms of airway resistance and lung expansion Copyright © 2019 Wolters Kluwer All Rights Reserved CONTROL OF BREATHING Lung Stretch Irritant Juxtacapillary (J Receptor receptors) Located in the smooth Located between the Located in the muscle layers of the airway epithelial cells alveolar wall, close to conducting airways the pulmonary capillaries Respond to changes in Stimulated by noxious Sense lung congestion pressure in the walls of gases, cigarette smoke, the airways inhaled dust, and cold air When the lungs are Stimulation of the irritant May be responsible inflated, they inhibit receptors leads to airway for the rapid, shallow inspiration and promote constriction and a pattern breathing that occurs expiration of rapid, shallow with pulmonary breathing. (Probably edema, pulmonary protects respiratory tissues embolism, and from the damaging pneumonia effects of toxic inhalants) Important in establishing Mechanical stimulation breathing patterns and initiates periodic sighing minimizing the work of and yawning (may ensure breathing by adjusting more uniform lung respiratory rate and VT expansion Copyright © 2019 Wolters Kluwer All Rights Reserved CONTROL OF BREATHING Voluntary Regulation of Ventilation Integrates breathing with voluntary acts such as speaking, blowing, and singing These acts, initiated by the motor and premotor cortex, cause a temporary suspension of automatic breathing Copyright © 2019 Wolters Kluwer All Rights Reserved COUGH REFLEX One of the primary defense mechanisms of the respiratory tract Neurally mediated reflex that protects the lungs from: Accumulation of secretions Entry of irritating and destructive substances Initiated by receptors located in the tracheobronchial wall Extremely sensitive Afferent impulses are transmitted through the vagus to the medullary center, which integrates the cough response. Copyright © 2019 Wolters Kluwer All Rights Reserved MECHANISMS INVOLVED IN DYSPNEA Stimulation of lung receptors Associated conditions Increased sensitivity to Primary lung diseases changes in ventilation Heart disease perceived through central Neuromuscular disorders nervous system mechanisms Reduced ventilatory capacity or breathing reserve Stimulation of neural receptors in the muscle fibers of the intercostals and diaphragm and of receptors in the skeletal joints Copyright © 2019 Wolters Kluwer All Rights Reserved QUESTION #3 Which of the following accurately describes your breathing pattern after running to class? A. Cheyne-stokes B. Normal C. Dyspnea D. Eupnoea E. Hypoxemia Copyright © 2019 Wolters Kluwer All Rights Reserved ANSWER TO QUESTION #3 C. Dyspnea Rationale: Dyspnea is simply labored breathing; it is not necessarily pathological in nature. Copyright © 2019 Wolters Kluwer All Rights Reserved IN SUMMARY Respiratory system Circulation Conducting airways: air warmed, filtered & Pulmonary circulation: pulmonary artery; provides for the gas exchange function humidified as it passes into and out of the of the lungs lungs(nasal passages, mouth, nasopharynx, larynx, tracheobronchial tree). Bronchial circulation (thoracic aorta) distributes blood to the conducting Respiratory tissues (respiratory bronchioles, airways & supporting structures of the alveoli, and pulmonary capillaries): where lung gas exchange takes place Innervation Lungs also inactivate bradykinin; they Parasympathetic innervation causes convert angiotensin I to angiotensin II; airway constriction and an increase in respiratory secretions Gas exchange Sympathetic innervation causes Oxygen from the alveoli diffuses across the bronchial dilation and a decrease in alveolar-capillary membrane into the respiratory tract secretions blood, and carbon dioxide from the blood Pleura diffuses into the alveoli. Thin, double-layered serous membrane; Type I Alveolar cells: provide the gas parietal and visceral exchange function of the lung. Pleural cavity is a potential space in Type II Alveolar cells: produce surfactant which serous fluid or inflammatory exudate can accumulate and serve as progenitor cells for type I cells Copyright © 2019 Wolters Kluwer All Rights Reserved IN SUMMARY Properties of Gases Circulation Conducting airways: air warmed, filtered & Pulmonary circulation: pulmonary artery; provides for the gas exchange function humidified as it passes into and out of the of the lungs lungs(nasal passages, mouth, nasopharynx, larynx, tracheobronchial tree). Bronchial circulation (thoracic aorta) distributes blood to the conducting Respiratory tissues (respiratory bronchioles, airways & supporting structures of the alveoli, and pulmonary capillaries): where lung gas exchange takes place Innervation Lungs also inactivate bradykinin; they Parasympathetic innervation causes convert angiotensin I to angiotensin II; airway constriction and an increase in respiratory secretions Gas exchange Sympathetic innervation causes Oxygen from the alveoli diffuses across the bronchial dilation and a decrease in alveolar-capillary membrane into the respiratory tract secretions blood, and carbon dioxide from the blood Pleura diffuses into the alveoli. Thin, double-layered serous membrane; Type I Alveolar cells: provide the gas parietal and visceral exchange function of the lung. Pleural cavity is a potential space in Type II Alveolar cells: produce surfactant which serous fluid or inflammatory exudate can accumulate and serve as progenitor cells for type I cells Copyright © 2019 Wolters Kluwer All Rights Reserved