Cardiovascular Physiology Fall 2024 PDF
Document Details
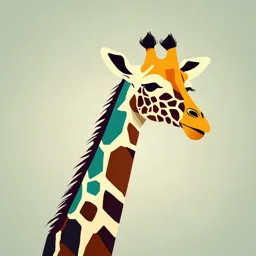
Uploaded by ExaltedZombie
University of Alberta
2024
Kyla Smith, PhD
Tags
Summary
These lecture notes cover Cardiovascular Physiology, likely for a Fall 2024 undergraduate course.
Full Transcript
Cardiovascular Physiology Kyla Smith, PhD [email protected] Reading (optional): Chapter 12 Vander’s Why Do We Have a Cardiovascular System? The requirement for a circulating system is an evolutionary consequence of the increasing c...
Cardiovascular Physiology Kyla Smith, PhD [email protected] Reading (optional): Chapter 12 Vander’s Why Do We Have a Cardiovascular System? The requirement for a circulating system is an evolutionary consequence of the increasing complexity of large multicellular organisms Diffusion is not adequate to supply nutrients to centrally located cells and to remove waste products as distances are too large The circulation provides a steep concentration gradient in close proximity to every cell allowing for the RAPID exchange of materials between blood and cells Cardiovascular Physiology Hemodynamics Hemodynamics F = ∆P/R P1 P2 F = flow (mL/min) ∆P = pressure difference between two fixed points (P1 and P2) (mmHg) P1 = 100 mmHg P2 = 10 mmHg ∆P = 90 mmHg R = resistance to flow (mmHg/mL/min) Flow rate = 10 mL/min Flow: high to low pressure P1 P2 To have flow, ∆P > R P1 = 500 mmHg P2 = 410 mmHg ∆P = 90 mmHg Flow rate = 10 mL/min Changing R will change F R= ∆P/F = 90mmHg/10mL/min Hydrostatic pressure → pressure = 9mmHg/mL/min exerted by a fluid Hemodynamics What happens when there is no pressure difference? R= 10 mmHg/mL/min (given) P1 P2 F= ∆P/ R = (P1 - P2)/R = (100 mmHg - 100 mmHg)/ P1 = 100 mmHg ∆P = 0 mmHg P2 = 100 mmHg 10 mmHg/mL/min = 0 mmHg/10 mmHg/mL/min = 0 mL/min Pressure difference between two points (P1, P2) creates flow Pressure Differences in pressure move blood – Pressure gradient (not absolute pressure) creates blood flow Pressure created from contraction of heart chambers and pressure of blood on the walls of the blood vessels and heart chambers What Determines Resistance to Blood Flow? Viscosity, length of the vessel and diameter of the vessel – Viscosity → friction between molecules of a flowing fluid – Length and diameter → determines amount of contact between moving blood and stationary wall of vessel – Poiseuille’s equation R = 8ηl Layers of blood flowing through small πr4 and large vessels Parabolic flow profile ▪ R = resistance to blood flow V=0 ▪ η = viscosity of blood V max ▪ l = length of vessel ▪ r = radius of vessel (raised to fourth power) 2^4=16 Resistance to Blood Flow Laminar flow Transitional flow Turbulent flow In fluid dynamics, laminar flow is characterized by fluid particles following smooth paths in layers, with each layer moving smoothly past the adjacent layers with little or no mixing. Cardiovascular Physiology Cardiovascular System Functions and Components Functions of the Cardiovascular System To deliver oxygen and nutrients and remove waste products of metabolism Fast chemical signaling to cells by circulating hormones or neurotransmitters Thermoregulation Mediation of inflammatory and host defense responses against invading microorganisms Components of the Lungs Pulmonary capillaries Cardiovascular System Pulmonary circulation Pulmonary trunk Pulmonary and arteries veins Cardiovascular system: Vena cava – Heart Right Left Aorta atrium atrium – Blood vessels Right Left ventricle ventricle – Blood Systemic circulation Arteries → away from the heart Veins → towards the heart Systemic Closed circulatory system Systemic veins arteries – Generate greater pressures Systemic arterioles, capillaries, and venules in all organs and tissues Chapter 12 Vander’s Human Physiology except the lungs Anatomy of the Heart Four chambers: Aorta Base – 2 atria – 2 ventricles Superior vena cava Pulmonary trunk Atria Left atrium – Thin-walled Right atrium – Low pressure Left ventricle chambers Inferior vena cava – Receive blood returning to the heart Right ventricle Ventricles Right side of heart Left side of heart – Forward propulsion of blood Apex Septa Divided by septa – Interatrial septum separates left and right atria – Interventricular septum separates Interatrial septum left and right ventricles Dual pump Interventricular septum Cardiovascular Physiology 2 Serial Circuits The Circulatory System: 2 Serial Circuits Pulmonary circulation – Blood to and from the gas exchange surfaces of the lungs – Blood entering lungs = poorly oxygenated blood – Oxygen diffuses from lung tissue to blood – Blood leaving lungs = oxygenated blood Systemic circulation – Blood to and from the rest of the body – Blood entering tissues = oxygenated blood – Oxygen diffuses from blood to body tissues – Blood leaving tissues = poorly oxygenated blood Pulmonary trunk Path of Blood Flow Pulmonary arteries Pulmonary arterioles Capillaries of lungs Pulmonary Pulmonary venules circulation Left heart: receives blood from Pulmonary veins pulmonary circulation and pumps Pulmonary valve Left atrium to systemic circulation Right ventricle Left AV valve Right AV valve Left ventricle Heart Right heart: receives blood from Right atrium Aortic valve systemic circulation and pumps to Aorta pulmonary circulation Arteries Arterioles Capillaries Systemic Venules circulation Veins Venae cavae head and upper limbs Series vs Parallel Flow jugular veins capillaries carotid arteries to head, subclavian arteries to upper from head, limbs Series flow within the cardiovascular subclavian veins from upper limbs system superior pulmonary vena cava pulmonary artery artery – Blood must pass through the pulmonary and systemic circuits capillaries capillaries in sequence pulmonary Right lung inferior Left Lung vein Parallel flow within the systemic vena cava circuit (most organs) liver digestive tract – Each organ supplied by Veins returning blood to heart kidneys Arteries supplying blood to organs different artery capillaries – Independently regulate flow pelvic organs to different organs capillaries lower limbs Distribution of Blood Flow at Rest and During Exercise Flow during strenuous exercise (mL/min) Flow at rest (mL/min) Cardiovascular system must ensure adequate perfusion of capillaries supplying the organs at rest, during exercise or emergency situations. Cardiovascular Physiology The Pericardium Functions of the Pericardium Pericardium → fibrous sac surrounding the heart and roots of great vessels Functions of pericardium: – Stabilization of the heart in thoracic cavity – Protection of the heart from mechanical trauma, infection – Secretes pericardial fluid to reduce friction – Limits overfilling of the chambers, prevents sudden distension The Pericardial Structure Fibrous pericardium 3 layers Parietal layer – (1) Fibrous pericardium Pericardial cavity – Serous pericardium Visceral layer ▪ (2) Parietal ▪ (3) Visceral (epicardium) Fibrous pericardium Parietal Pericardial cavity layer Visceral layer – Pericardial fluid (epicardium) decreases friction Diaphragm The Pericardial Structure Fibrous Parietal Pericardial cavity Visceral (epicardium) Heart chamber Inside heart Outside heart Cardiac Tamponade Pericardial cavity Pericarditis → inflammation of pericardium Cardiac tamponade → compression of heart chambers due to excessive accumulation of pericardial fluid Decreases ventricular filling Cardiovascular Physiology The Heart Wall and Cardiac Muscle Cells Ventricular Walls Left ventricular wall Right ventricular wall Left ventricle is thicker (wall) than the right ventricle – Left ventricle develops higher pressures than right ventricle Ventricular Walls Right Left http://www.med.uottawa.ca/patho/eng/Public/cardio/lab2.html The Heart Wall Epicardium (visceral pericardium) Fibrous pericardium Parietal pericardium – Covers outer surface of heart Pericardial cavity Myocardium – Muscular wall ▪ Cardiac muscle cells (myocytes), blood vessels, nerves Endocardium Endocardium – Endothelium covering inner surfaces Myocardium of heart and heart valves Epicardium Structure of atria and ventricles (visceral pericardium) Cardiac Muscle Cells Myocyte = cardiac muscle cell – Branched (“Y”) and joined longitudinally – Striated, one nucleus per cell, many mitochondria Intercalated disk Nucleus Intercalated disk – Interdigitated region of attachment ▪ Desmosomes and gap junctions Branching of myocyte (Y-shaped) Desmosomes Anchor cells together in tissues Plasma membranes subject to considerable stretching Intermediate filament Dense Mechanically couple cells plaque Extracellular space Cadherins Gap Junctions Communicating junctions Plasma Transmembrane channels membranes linking adjacent cells Gap-junction membrane protein (connexons) Electrically couple heart cells Extracellular 1.5 nm diameter space channels linking – Small molecules and ions cytosol of adjacent cells – Spreading action potentials across the atria or ventricles Cardiovascular Physiology The Heart Valves The Four Valves of the Heart Valves – Thin flaps of flexible, endothelium-covered fibrous tissue attached at the base to Valve rings the valve rings Pulmonary ▪ Leaflets or cusps (semilunar) valve ▪ Collagen Aortic (semilunar) valve Valve rings – Dense fibrous connective Left AV (bicuspid or tissue mitral) valve – Site of attachment Right AV (tricuspid) valve for the heart valves The Valves and the Path of Blood Flow Pulmonary trunk Pulmonary arteries Pulmonary arterioles Pulmonary Capillaries of lungs circulation Pulmonary venules Pulmonary veins Pulmonary valve Left atrium Right ventricle Left AV valve Right AV valve Left ventricle Heart Right atrium Aortic valve Aorta Arteries Arterioles Capillaries Systemic Venules circulation Veins Venae cavae Chapter 12 Vander’s Human Physiology How do the Valves Function? Unidirectional flow of blood through heart Open/close passively due to pressure gradients – Forward pressure gradient opens one-way valve – Backward gradient closes one-way valve but it cannot open in the opposite direction When the pressure is When the pressure is greater behind the valve, greater in front of the it opens valve, it closes Direction of flow Atrioventricular (AV) Valves Between atria and ventricles Prevent backflow of blood into atria when ventricles contract Tricuspid valve Left atrium – Right AV valve Right atrium Mitral valve – Three leaflets (cusps) Left ventricle Bicuspid valve (mitral valve) – Left AV valve Tricuspid valve – Two leaflets (cusps) Right ventricle AV valve apparatus: cusps, chordae tendineae and papillary muscles Anatomy of the AV Valve Apparatus Chordae tendineae – Tendinous-type tissue – Extend from edges of each leaflet to papillary muscle Cusps Chordae Papillary muscles tendineae – Cone shaped muscles Cusps – Contraction of papillary muscle causes the chordae tendineae to become taut Chordae Papillary tendineae muscles Function of the AV Valve Apparatus Prevents eversion of the AV valves into the atria during contraction of the ventricles Valves open and close due to pressure gradients, not from contraction and relaxation of papillary muscles Pulmonary veins Pulmonary veins Left atrium Left atrium Aorta Aorta Bicuspid valve Bicuspid valve (open) Aortic semilunar (closed) valve (closed) Aortic semilunar Chordae tendineae Chordae tendineae valve (closed) (slack; tension low) (taut; tension high) Papillary muscle (relaxed) Papillary muscle Cardiac muscle (contracted) (relaxed) Cardiac muscle (contracted) Left ventricle (relaxed) Left ventricle (contracted) Arterial (Semilunar)Valves Between the ventricle and artery 3 cusps Aorta – Pulmonary valve ▪ Pulmonary trunk, right Aortic valve ventricle – Aortic valve ▪ Aorta, left ventricle No chordae tendineae, papillary muscles Left ventricle Prevent the backflow of blood from the arteries into ventricles when ventricles relax Cardiac Skeleton Pulmonary valve Fibrous skeleton of the heart Pulmonary trunk – Dense connective tissue – Separates atria from ventricles Aortic – Electrically inactive valve – Support for heart Mitral Aorta ▪ Point of attachment valve Tricuspid valve for valve leaflets, myocardium Valve rings Cardiac muscle attaches to cardiac Dense connective tissue skeleton between valve rings Cardiovascular Physiology The Conducting System of the Heart Membrane Potential Membrane potential (voltage) → difference in electrical potential between the interior and exterior of a cell – All living cells maintain a potential difference across their membrane – More negative inside than outside at rest – Membrane voltage, or potential, is determined at any time by the relative ratio of ions (extracellular to intracellular) and the permeability of each ion Na+ - Na+ - - K+ K+ -- - Na+ K+ Na+ Na+ - K+- -70 K+ - - + K+ - - Cl- - K+ K Cl- K+ Cl- Na+ Cl- Na+ Na+ Na+ Cl- K+ What is an Action Potential? Action potential: ‒ Electrical signal ‒ Gives cells the ability to communicate ‒ Occurs in excitable cells ▪ Unique ability to generate and conduct electrical impulses ▪ ie. Neurons, cardiac myocytes The Action Potential Nerve/skeletal muscle action potential Depolarization Repolarization Na+ K+ Skeletal muscle/nerve Need a stimulus to action potential reach threshold 0 1 2 3 4 Time (msec) Hyperpolarization Action potential → rapid change in membrane potential where the inside transiently becomes more positive than the outside – Muscle cells: action potentials initiate contraction The action potential is brought on by a rapid change in membrane permeability to certain ions The Action Potential Nerve/skeletal muscle action potential Depolarization Repolarization Na+ K+ Skeletal muscle/nerve Need a stimulus to action potential reach threshold 0 1 2 3 4 Time (msec) Threshold potential → critical level to which the membrane potential must be depolarized in order to initiate an action potential Depolarization → process by which the membrane potential becomes less negative (ie. A reduction in the magnitude of the negative membrane potential) Repolarization → return of the membrane potential to resting potential following a depolarization The Cardiac Syncytium Cardiac muscle cells (myocytes) communicate with each other, and this arrangement is called a syncytium – Set of cells that act together Branching of myocyte (Y-shaped) Functional syncytium → if one cell is excited, the excitation spreads over both ventricles (or both atria) – 2 syncytia: atrial syncytium and ventricular syncytium All-or-none property Cardiac Muscle Action potentials lead to contraction of heart muscle cells Automaticity (autorhythmicity)→ cardiac muscle contracts in the absence of neural or hormonal stimulation, as a result of action potentials it generates itself Two types of cardiac muscle cells (myocytes): – Contractile cells → mechanical work of pumping, propel blood; do not initiate action potentials – Conducting cells (autorhythmic cells) → initiate and conduct the action potentials responsible for contraction of the contractile myocytes (1 % of myocytes); few myofibrils ▪ Conducting system Components of the Conducting System Internodal Atrioventricular node pathway Sinoatrial Bundle of His node Left Sinoatrial (SA) node Internodal atrium pathway Internodal pathways Right Atrioventricular (AV) node atrium Bundle of His (AV bundle) Right Bundle branches → left and bundle branch Left right Right ventricle Purkinje fibers ventricle Purkinje fibers Left bundle branch Cardiac Skeleton AV node Bundle of His Left atrium Non-conducting Right atrium AV node and Bundle of His – Only electrical connection between Left ventricle atria and ventricles Right ventricle Atrioventricular valves Sinoatrial (SA) Node Cardiac action potential is Internodal pathway not initiated by the nervous system; it arises from a group Sinoatrial of specialized cells known as node Internodal pacemaker cells pathway ‒ SA node is the cardiac Atrioventricular pacemaker node Initiates action potentials at fastest rate to set heart rate Internodal pathways – Stimulus is passed to contractile cells of both atria and to AV node Cardiac skeleton isolates the atrial and ventricular myocardium Atrioventricular (AV) Node Atrioventricular node AV nodal delay – 100 msec delay – Delay ensures atria depolarize and contract before the ventricles ▪ Contraction of the ventricles would close the AV valves, preventing blood flow from the atria into the ventricles ▪ Allows ventricles time to fill completely before they contract Excitation of the Ventricles Atrioventricular node Interatrial septum AV node and Bundle of His are Bundle of His Sinoatrial only electrical connection node between atria and ventricles Internodal pathway Left and right bundle branches travel along interventricular septum Purkinje fibers Right bundle – Large number, diffuse branch distribution, fast conduction velocity Purkinje fibers – Left and right ventricular myocytes depolarize and contract nearly simultaneously Interventricular Left bundle septum branch Sequence of Excitation RA LA SAN AVN Cardiac skeleton RV LV Bundle of His = Contractile cells RA = Right atrium LA = Left atrium = Conducting cells of internodal pathways RV = Right ventricle SAN = Sinoatrial node LV = Left ventricle AVN = Atrioventricular node Summary: Conducting System of the Heart SA node Internodal pathways Atrial myocardium AV node Bundle of His Right and left bundle branches Purkinje fibers Ventricular myocardium Wolff-Parkinson-White Syndrome Normal electrical pathways Accessory pathway Accessory pathway – Electrical signals bypass the AV node and move from the atria to the ventricles faster than usual Transmits electrical impulses abnormally from the ventricles back to the atria Rapid heart rate (tachycardia), arrhythmias Cardiovascular Physiology Action Potentials in the Heart Two Types of Action Potentials Fast action potential Slow action potential – Atrial myocardium – Sinoatrial node – Ventricular myocardium – Atrioventricular node – Bundle of His, Bundle branches, Purkinje fibers Chapter 12 Vander’s Human Physiology The Cardiac Action Potential Phases of the cardiac action potential are associated with changes in permeability of the cell membrane mainly to Na+, K+ and Ca2+ ions – Opening and closing of ion channels alters permeability Myocyte K+ K+ [K+]IN > [K+]OUT Ca2+ Ca2+ [Ca2+]OUT > [Ca2+]IN Na+ Na+ [Na+]OUT > [Na+]IN SA Node Action Potential Pacemaker potential ‒ Slow depolarization to threshold – Regular spontaneous generation of action potentials – The discharge rate of the SA node determines the heart rat 1. Pacemaker potential ~ 150 msec → K+ channels: iK → F-type channels (funny): iNa → T-type channels (transient): iCa 2. Depolarization → L-type channels (long lasting): iCa 3. Repolarization → K+ channels: iK Summary of Slow Action Potential Repolarization phase: Opening of K+ channels and closing of L-type Ca2+ Depolarization phase: L-type channels. K+ exits through K+ channels. channels open at threshold and Ca2+ slowly enters cell. Membrane potential (mV) 0 Pacemaker potential: Closing of Threshold K+ channels, Na+ entering through F-type channels and Ca2+ –50 entering through T-type channels Ca2+ enters through Na+ enters through T-type channels Begin: -60 mV. F-type channels K+ channelsbegin to –100 close 0 0.15 0.30 Time (sec) Depolarization phase is slow due to slow movement of Ca2+ Pacemaker potential due to changes in movement of ions Is the action potential different in SA vs AV nodes? AV node pacemaker current rises to threshold more slowly The Action Potential Nerve/skeletal muscle action potential Depolarization Repolarization Na+ K+ Skeletal muscle/nerve Need a stimulus to action potential reach threshold 0 1 2 3 4 Time (msec) Threshold potential → critical level to which the membrane potential must be depolarized in order to initiate an action potential Depolarization → process by which the membrane potential becomes less negative (ie. A reduction in the magnitude of the negative membrane potential) Repolarization → return of the membrane potential to resting potential following a depolarization Ventricular Muscle Cell Action Potential Resting phase Depolarization Notch Plateau Repolarization ECF Na+ Ca2+ ICF K+ K+ K+ K+ Depolarization: Notch: Due to transient Opening of fast opening of K+ channels 0 voltage-gated Na+ channels at Membrane potential (mV) threshold Plateau: Ca2+ entering through L-type channels and Repolarization: slow opening of K+ channels Opening of K+ channels that will repolarize cell and closing of Ca2+ channels Stable resting phase: K+ Threshold 250 - 300 msec -100 0 0.15 0.30 Time (sec) Ventricular Muscle Cell Action Potential Comparison of the Cardiac Action Potentials Sinoatrial Node Threshold Longer to reach threshold Threshold Atrioventricular Node Shorter plateau Atrial Myocardium Ventricular Myocardium Cardiovascular Physiology Electrocardiogram (ECG) The Electrocardiogram (ECG, EKG) Graphic recording of electrical events Electrical activity of the heart detected on the surface of the body Voltage gradients in the heart may be as much as 100 mV → this is translated to changes of up to 1 mV at the skin surface Used to diagnose problems with the heart’s conduction system Placement of Electrodes in the 12 Lead ECG (–) Lead I (+) (+) (–) (+) (–) (–) aVR aVL (–) Lead II (–) Lead III V1 V2 aVF V6 (+) V3 V4 V5 Ground (+) (+) Different angles for viewing heart’s electrical activity Established electrode pattern results in specific tracing pattern ECG Recording P-wave → spread of depolarization across atria – Atria contract 25 msec after start of R P-wave +1 Potential (mV) QRS-complex → spread of P T depolarization across ventricles – Atria repolarize simultaneously 0 Q S T-wave → ventricular repolarization Time (sec) The ECG and the Heart’s Action Potentials +1 R Potential (mV) Ventricular Depolarization The relationship T between the P electrocardiogram 0 Q S (ECG) Atrial Depolarization Ventricular Repolarization +20 And…….. Membrane potential (mV) Typical action potentials Ventricular action Atrial recorded from atrial and action potential potential ventricular myocardial cells –90 0.3 Time (sec) Some ECGs…. 1. Normal ECG QRS QRS QRS QRS QRS ‒ P-wave always followed by P T P T P T P T P QRS complex and T-wave No QRS complex or T-wave 2. Partial AV node block QRS QRS QRS ‒ Every 2nd P-wave is not P T P P T P P T followed by QRS complex Increased time between P-wave and QRS 3. Complete AV node block QRS QRS ‒ No synchrony between atrial P P T P P P T and ventricular electrical activities No QRS complex or T-wave ‒ Ventricles driven by Not in correct order slower bundle of His Cardiovascular Physiology Excitation-contraction Coupling (ECC) in the Heart Cardiac Myocytes 2 myocytes joined by intercalated disks Intercalated disks Branching of myocyte Sarcoplasmic reticulum Mitochondrion Gap junction Cardiac muscle cell Nucleus Sarcolemma Desmosome Sarcolemma → cell membrane of muscle cell Sarcoplasmic reticulum → stores calcium ions for contraction Cardiac Myocytes Branching muscle fibers Intercalated disks T-tubule Invagination of sarcolemma into T-tubule Sarcoplasmic reticulum Nucleus of cardiac muscle cell Sarcomere Mitochondrion Sarcolemma (cell membrane) Myofibrils Connective tissue T-tubule and sarcoplasmic reticulum in close proximity Striated T-tubules (Transverse-tubules) → invaginations of sarcolemma; transmit depolarization of membrane into interior of muscle cell Excitation-contraction Coupling (ECC) in Cardiac Muscle Calcium ions regulate the contraction of cardiac muscle – Calcium ions bind to ryanodine receptors, releasing calcium from the sarcoplasmic reticulum ▪ This is called calcium-dependent calcium release ▪ 95% of the calcium in cytosol FYI: Activation of Cross-bridge Cycling by Ca2+ Low cytosolic Ca2+, relaxed muscle Troponin Tropomyosin Actin Troponin → contains binding sites for calcium and tropomyosin, and Actin binding site regulates access to myosin- binding sites on actin Energized myosin cross-bridge cannot bind to actin Tropomyosin → partially High cytosolic Ca2+, activated muscle cover the myosin-binding Ca2+ sites on actin at rest, Cross-bridge binding preventing cross-bridges sites are exposed from making contact with actin Cross-bridge binds to actin and generates force ECC in Cardiac Muscle: Contraction Excitation spreads along the Calcium causes the release of sarcolemma (plasma membrane) calcium from sarcoplasmic from cell to cell by gap junctions reticulum through calcium-release channels (ryanodine receptors) Excitation also spreads to interior of cell by T-tubules Elevation of cytosolic calcium During plateau phase of action Calcium binds to troponin potential, permeability of the cell → → unblocks active sites to calcium increases between actin and myosin Calcium enters through L-type Ca2+ Cross-bridge cycling and channels in sarcolemma and T-tubules contraction of myofibrils Release of Calcium During ECC Extracellular fluid 1. Muscle cell action potential Voltage-gated Na+ propagated into T-tubules Na+ channel Na+ Ryanodine Cytoplasm Sarcoplasmic receptor reticulum 2. Ca2+ enters the L-type channels Ca2+ Ca2+ Ca2+ 3. Ca2+ binds to ATPase ryanodine receptors pump Ca2+ 5. Ca2+ binds 4. Ca2+ is released L-type to troponin from the SR Ca2+ channel Ca2+ Ca2+ Troponin Transverse tubule Z Sarcomere Z L-type Ca2+ channel – Voltage-gated Ca2+ channel – Modified DHP receptors DHP = dihydropyridine ECC in Cardiac Muscle: Relaxation Influx of calcium stops as Reduced calcium binding L-type Ca2+ channels close to troponin SR is no longer stimulated to Sites for interaction between release calcium myosin and actin are blocked SR takes up cytosolic Relaxation of myofibrils calcium by Ca2+-ATPase Calcium removed from cell by Na+-Ca2+ exchanger Uptake of Calcium During ECC Extracellular fluid Ca2+ leaves cell Voltage-gated Na+ Na+ Na+ channel through Na+-Ca2+ Na+ exchanger Voltage-gated Ca2+ release Cytoplasm Ca2+ channel channel Ca2+ Ca2+ Sarcoplasmic reticulum Ca2+ Ca2+ ATPase Transverse pump tubule Ca2+ Ca2+ returned to SR by 2+ Ca -ATPase Ca2+ Ca2+ Troponin Z Sarcomere Z Refractory Period Na+ channels close and inactivate Period of time in which a new Na+ channels recover action potential cannot be Na+ channels open from inactivation and can open again initiated +20 following a stimulus Plateau Tension 0 Absolute Refractory Period Action developed Membrane potential (mV) potential – ~ 250 msec – No response to another stimulus Absolute – Inactivation of Na+ channels Refractory period –80 Prevents tetanic contraction 0 150 300 Time (msec) Cardiovascular Physiology Phases of the Cardiac Cycle The Cardiac Cycle The cardiac cycle is divided into two parts: – Systole → ventricular contraction and blood ejection – Diastole → ventricular relaxation and blood filling Example: Heart rate = 72 beats/min Cardiac cycle length is ~800 msec Systole, 300 msec; diastole, 500 msec One heart beat is 800 msec Systole Isovolumetric ventricular contraction Ventricular ejection Blood flows out of ventricle Atria Atria relaxed relaxed Ventricles Ventricles contract contract AV valves: Closed Closed Semilunar valves: Closed Open Isovolumetric ventricular contraction → all heart valves closed, blood volume in ventricles remains constant, pressures rise. Muscle develops tension but cannot shorten Ventricular ejection → pressure in ventricles exceeds that in arteries, semilunar valves open and blood ejected into the artery. Muscle fibers of ventricles shorten Stroke volume → volume of blood ejected from each ventricle during systole Isovolumetric ventricular relaxation Diastole Ventricular filling - Blood flows into ventricles Atrial contraction Atria Atria Atria relaxed relaxed contract Ventricles Ventricles Ventricles relaxed relaxed relaxed AV valves: Closed Open Open Semilunar valves: Closed Closed Closed Isovolumetric ventricular relaxation → all heart valves closed, blood volume remains constant, pressures drop Ventricular filling → AV valves open, blood flows into ventricles from atria. Ventricles receive blood passively (atria are relaxed) – Passive ventricular filling → majority of ventricles (70 %) are filled passively Atria contract at the end of ventricular filling (atrial kick) Phases of the Cardiac Cycle AV valves open, semilunar valves closed. Atrial systole begins: atrial contraction forces Ventricles in a small amount of blood into relaxed (Atrial kick) diastole, atria to ventricle, completing ventricular filling. enter systole. Ventricular diastole: Passive ventricular filling. All chambers are relaxed. AV Atrial systole ends, valves open as pressure in ventricles atrial diastole begins. drops below the atria. Blood flows Atrial systole Ventricular systole: passively from atria into ventricles. Isovolumetric contraction. Ventricles contract, closing tricular diastole Ventricular systo Cardiac AV valves, but do not create cycle enough pressure to open semilunar valves (were closed Ven e in previous diastole). l Atrial diastole Ventricular diastole: Ventricular systole: Ejection. Isovolumetric relaxation. Ventricle pressures rise and Ventricles relax, pressure in exceed pressures in arteries. ventricles drops, blood flows back The semilunar valves open and against cusps of semilunar valves blood is ejected into the arteries. and closes them. AV valves are closed. Cardiovascular Physiology The Pressure-volume Curve: Wigger’s Diagram Pressure-volume Curve Pressure is the key to understanding blood flow Wigger’s diagram patterns and opening and Dicrotic notch 110 closing of valves Pressure (mmHg) Aortic pressure 50 Blood flows from a region of Left atrial pressure higher pressure to a region of Left ventricular pressure 0 lower pressure Left ventricular volume (mL) End-diastolic Left ventricular volume 130 volume Valves open and close in 65 QRS End- response to a pressure gradient P T systolic volume ECG 1st 2nd 1 = Ventricular filling Heart sounds 2 = Isovolumetric ventricular contraction Diastole Systole Diastole Phase of cardiac cycle 1 2 3 4 1 3 = Ventricular ejection 4 = Isovolumetric ventricular relaxation Pressure-volume Curve End-diastolic volume (EDV) → amount of blood in each ventricle at the end of ventricular diastole End-systolic volume (ESV) → amount of blood in each ventricle at the end of ventricular systole Stroke volume → volume of blood pumped out of each ventricle during systole SV= EDV – ESV ~70 - 75 mL (ventricle does not empty completely) Pressure-volume Curve Pressure in ventricle increases Aortic pressure Aortic valve as ventricle 110 opens Pressure (mmHg) contracts Aortic valve Isovolumetric closes Isovolumetric ventricular 50 ventricular contraction relaxation Left atrium and Left AV ventricle are valve opens relaxed. Aortic 0 valve closed. Left ventricular volume (mL) 130 Left AV Ventricular valve closes EDV ejection 65 QRS Ventricle Passive filling almost full ESV from passive P T filling ECG 1st 2nd Diastole Systole Diastole 2 3 4 1 Phase of cardiac cycle The Right Heart 1 2 3 4 1 Pressure (mmHg) 50 The right ventricle Pulmonary artery develops lower pressure pressures than the left ventricle during systole 0 Right ventricular pressure Time 1 = Ventricular filling 2 = Isovolumetric ventricular contraction 3 = Ventricular ejection 4 = Isovolumetric ventricular relaxation Heart Sounds 2 heart sounds First heart sound → Lub 110 Pressure (mmHg) – Closure of AV valves Aortic valve closes 50 Second heart sound → Dub 0 – Closure of semilunar valves Left ventricular volume (mL) End-diastolic 130 volume Left AV valve closes Sounds result from vibrations 65 QRS End- systolic caused by (passive) closing of the P T volume valves 1st 2nd Heart sounds Diastole Systole Diastole 1 2 3 4 1 Cardiovascular Physiology Regulation of the Heart: ANS Autonomic Innervation of the Heart Parasympathetic Sympathetic Vagus nerve Thoracic spinal nerves Acetylcholine Norepinephrine M Atria β Adrenal Epinephrine medulla Ventricles β M = muscarinic β = beta-adrenergic Bloodstream Sympathetic innervation → atria, ventricles, SA node, AV node Parasympathetic innervation → atria, SA node, AV node Effect of the ANS on the Heart Parasympathetic Sympathetic stimulation stimulation SA node ↓ rate of depolarization ↑ rate of depolarization to threshold; ↓ HR to threshold; ↑ HR AV node ↓ conduction; ↑ conduction; ↑ AV nodal delay ↓ AV nodal delay Atrial muscle ↓ contractility ↑ contractility Ventricular muscle No significant ↑ contractility innervation; no effect Cardiovascular Physiology Regulation of Cardiac Output (CO) Cardiac Output Cardiac output → amount of blood pumped by each ventricle in one minute CO = HR * SV cardiac heart stroke output rate volume (mL/min) (beats/min) (mL/beat) Example: CO = 72 beats/min * 0.07 L/beat = 5 L/min Stroke volume → amount of blood pumped out of each ventricle during systole (70 - 75 mL) Factors Affecting Cardiac Output Cardiac output Heart rate – Alter activity of SA node SA Node Ventricular myocardium Heart rate Stroke volume Stroke volume – Alter contractility of ventricular myocardium Factors Affecting Heart Rate Increased sympathetic activity – Increase heart rate Increased parasympathetic activity – Decrease heart rate Effect of the ANS on Heart Rate Sympathetic → ↑ the slope of the pacemaker potential (faster depolarization) 60 a, b, and c are pacemaker potentials: – Increase HR a = control – Increases F-type and T-type b = during sympathetic stimulation Membrane potential (mV) c = during parasympathetic stimulation channel permeability Parasympathetic →↓ the slope 0 of the pacemaker potential (slower depolarization) Threshold potential – Decrease HR –40 b a – Decreases F-type channel –60 c permeability Time – Hyperpolarizes cells (↑ K+ permeability) Effect of the ANS on the Pacemaker Potential = Pacemaker potential Control Threshold –50mV Sympathetic nerve effect –50mV Parasympathetic nerve effect –50mV 250 500 750 1000 Time (msec) Summary of Factors Affecting Heart Rate Plasma epinephrine Activity of Activity of sympathetic parasympathetic nerves to heart nerves to heart SA node Heart rate Factors Affecting Cardiac Output Cardiac output Ventricular SA Node myocardium Heart rate Stroke volume CO = HR x SV 3 factors affecting stroke volume: 1. End-diastolic volume (EDV; preload) 2. Contractility of the ventricles 3. Afterload Factors Affecting Stroke Volume: EDV EDV (preload) – Frank-Starling Mechanism – Preload → tension or load on myocardium before it begins to contract or amount of filling of ventricles at the end of diastole (EDV) The Frank-Starling Mechanism 200 Relationship between EDV and SV Increased stroke volume Stroke volume (mL) Main determinant of cardiac muscle 130 mL fiber (sarcomere) length is degree of Normal 100 resting diastolic filling: preload value 70 mL Increase filling → increase EDV → Increased venous return increase cardiac fiber length → 130 mL 210 mL greater force during contraction and 0 100 200 300 400 greater SV Ventricular end-diastolic volume (mL) Mechanism of the Length-tension Relationship Actin Myosin 1 Myosin Cross Bridges Left Ventricle 1 2 3 Heart Cells Z-line Z-line 2 Pressure 3 X Titin 2 1 X X Z-line Z-line 3 Volume Z-line Z-line Factors Affecting Stroke Volume: Contractility Contractility of ventricular myocardium – Contractility → strength of contraction at any given EDV Sympathetic Stimulation Increases Contractility Sympathetic stimulation During sympathetic stimulation, the Increased Stroke volume (mL) 200 contractility SV is greater at any given EDV – Ejection Fraction (EF) = SV/EDV 100 Normal Control resting value 0 100 200 300 400 More rapid contraction and Ventricular end-diastolic volume (mL) Increased force a more rapid relaxation of contraction During stimulation of sympathetic during contraction Force developed nerves to heart Control Rapid relaxation Rapid contraction Time Factors Affecting Stroke Volume: Afterload Afterload – Afterload → tension (or arterial pressure) against which the ventricles contract ▪ “Load” – As afterload increases, SV decreases – Any factor that restricts blood flow through arterial system will increase afterload ▪ Arterial blood pressure, vascular resistance, stenotic valve Cardiovascular Physiology The Vascular System Endothelium Smooth single-celled layer of endothelial cells Endothelium of vessels continuous with endocardium of the heart Physical lining that blood cells do not normally adhere to Arteries Smooth muscle, elastic fibers, connective tissue Elastic arteries – Many elastic fibers, few smooth muscle cells – Expand and recoil in response to pressure changes Muscular arteries – Many smooth muscle cells, few elastic fibers – Distribute blood Arterioles – 1 - 2 layers of smooth muscle cells – Resistance vessels Cardiovascular Physiology The Vascular System: Arterioles and Control of Arteriolar Smooth Muscle Arterioles Functions: 1. In individual organs, determine the Arteriole relative blood flow to the organ at Smooth any given mean arterial pressure muscle cell 2. All together are the major factor in determining mean arterial pressure (MAP) Endothelium – Causes drop in MAP as distance Precapillary from heart increases sphincter Capillary Circular smooth muscle – State of contraction can be regulated Arterioles and Resistance to Blood Flow High resistance vessels due to small size F = ∆P / R – Altering arteriolar diameter Difference in flow in ∆P Pressure reservoir each tube due to alters resistance and flow differences in resistance; (“arteries”) Vasodilation → relaxation of ∆P same for each tube arteriolar smooth muscle – Increased blood flow to Variable-resistance outflow tubes organs (“arterioles”) Vasoconstriction → contraction of arteriolar smooth muscle Flow to “organs” 1, 2, 3, 4, and 5 – Decreases blood flow to organs 1 2 3 4 5 MAP = mean arterial pressure Arterioles and Resistance to Blood Flow Intrinsic or basal tone – Arteriolar smooth muscle is partially contracted in the absence of external factors – Other factors can increase or decrease the state of contraction to cause vasoconstriction or vasodilation Extrinsic or intrinsic factors alter basal tone – Extrinsic → factors external to the organ or tissue; whole body needs (MAP); nerves and hormones – Intrinsic → local controls; organs and tissues alter their own arteriolar resistances independent of nerves or hormones Extrinsic Controls: ANS Sympathetic neurons − Arterioles innervated by sympathetic postganglionic neurons − NE → vasoconstriction (α-adrenergic receptors) − Can be used to cause vasodilation − Sympathetic tone can be increased (vasoconstriction) or decreased (vasodilation) (in addition to vessel’s intrinsic/basal tone) − To vasodilate: decrease rate of sympathetic activity to below the basal level − Regulating MAP Parasympathetic neurons − Little/no parasympathetic innervation of arteriolar smooth muscle Noncholinergic, nonadrenergic neurons ▪ NO (nitric oxide) → vasodilation Extrinsic Controls: Hormones Hormones – Epinephrine from adrenal medulla – Arteriolar smooth muscle may contain both adrenergic receptor subtypes (α/β) – Causes vasoconstriction (α-adrenergic receptors) or vasodilation (β2-adrenergic receptors) – Most vascular beds contain primarily α-adrenergic receptors (outnumber β2-adrenergic receptors) – Skeletal muscle has significant number of β2-adrenergic receptors Endothelial Cells and Vascular Smooth Muscle Endothelial cells − Substances may induce contraction or relaxation of vascular smooth muscle by acting on endothelial cells adjacent to arteriolar smooth muscle − Endothelial cells will produce paracrine substances that will diffuse to the smooth muscle to cause vasodilation or vasoconstriction − NO → paracrine substance; vasodilation (also called EDFR; endothelium-derived relaxing factor) − Released continuously by endothelial cells in arterioles to cause arteriolar vasodilation in basal state − Released in response to chemical mediators https://www.creative-diagnostics.com/vascular-smooth-muscle.htm Local Controls: Active Hyperemia Begin ↑ Metabolic ↓ O2, ↑ metabolites Arteriolar dilation ↑ Blood flow activity in organ interstitial in organ to organ of organ fluid Arteriolar smooth muscle is sensitive to local chemical changes (eg. O2, CO2, H+) Local chemical changes are the result of changes in metabolic activity Increased metabolic activity – Results in vasodilation of arterioles and increased blood flow No nerves or hormones involved Local Controls: Flow Autoregulation Begin ↓ O2, ↑ ↓ Arterial metabolites, ↓ Restoration of ↓ Blood flow Arteriolar dilation pressure vessel-wall stretch blood flow toward to organ in organ in organ in organ normal in organ Begin ↑ O2, ↓ ↑ Arterial metabolites, ↑ Arteriolar Restoration of ↑ Blood flow pressure vessel-wall stretch constriction blood flow toward to organ in organ in organ in organ normal in organ Changes in arterial blood pressure alter blood flow to an organ – Changes the concentration of local chemicals (eg. O2, CO2, H+) Arterioles change their resistance to maintain constant blood flow in the presence of a pressure change Constant metabolic activity No nerves or hormones involved Local Controls: Flow Autoregulation Begin ↑ O2, ↓ ↑ Arterial Arteriolar Restoration of ↑ Blood flow metabolites, pressure constriction blood flow toward in organ to organ ↑ Vessel-wall in organ normal in organ stretch in organ Flow autoregulation may also be mediated by the myogenic response – Direct response of arteriolar smooth muscle to stretch Cardiovascular Physiology The Vascular System: Capillaries Capillaries One endothelial cell thick – No smooth muscle or Basement Endothelial cell 1 Exocytotic membrane elastic tissue vesicles Nucleus Exchange of material between blood and interstitial fluid Intercellular cleft Intercellular clefts → narrow water-filled space at the Fused vesicle junctions between cells Intercellular channel cleft – Small water-soluble Erythrocyte Capillary Endothelial cell 2 lumen substances Types of Capillaries (a) Continuous capillary (b) Fenestrated capillary Fenestra with Fenestra without diaphragm a diaphragm (c) Sinusoidal capillary Large fenestra Continuous Capillaries Endothelial cell 1 Endothelial cell 2 No gap between cells Endothelial Endothelial cells form an cell 2 uninterrupted tube, surrounded by Pericyte Basement complete basement membrane membrane Intercellular cleft Exchange of water, small solutes, Pinocytotic vesicle lipid-soluble material, no exchange of blood and plasma proteins Endothelial cell 1 Erythrocyte Most tissues Endothelial Tight cell 3 junction Continuous Capillaries Red blood cell Pericyte Capillary endothelial cells Basement membrane Nucleus Fenestrated Capillaries Fenestra with Fenestra without diaphragm a diaphragm A. Endothelial cell with diaphragm B. Endothelial cell without diaphragm Fenestrae (pores) that penetrate Endothelial cells endothelial lining Erythrocyte Surrounded by complete basement membrane Filtration pores (fenestrations) Rapid exchange of water and solutes (small peptides) Basement Endocrine organs, choroid membrane plexus, GI tract, kidneys Intercellular cleft Sinusoids Gap between cells Large fenestra Endothelial cell 1 Endothelial cell 2 Sinusoids → discontinuous Endothelial Macrophage capillaries; flattened and irregularly cells shaped capillaries – Large fenestrae and gaps between Erythrocytes cells in sinusoid – Basement membrane thin or absent (hepatocyte) Liver cell Free exchange of water and solutes Microvilli (red blood cells, cell debris, plasma Sinusoid proteins) Liver, bone marrow, spleen Microcirculation Precapillary sphincter Intercellular – At entrance to a capillary clefts Endothelial – Ring of smooth muscle cell Smooth – Alters blood flow muscles Arteriole – No innervation ▪ Respond to local factors Precapillary sphincters Enlargement of capillary Metarteriole To veins Capillaries (precapillary arterioles) – Connects arterioles to venules Metarteriole – Contains smooth muscle cells Venule – Change diameter to regulate flow Distribution of Blood Flow at Rest and During Exercise Flow during strenuous exercise (mL/min) Flow at rest (mL/min) Capillary Exchange Diffusion → movement of substance down its concentration gradient – Exchanges nutrients, metabolic end products Filtration – Lipid soluble - through cells pores (fenestrae) – Lipid insoluble - through water-filled channels Plasma proteins Transcytosis - ▪ Intercellular clefts, generally cannot use of vesicles cross capillary fenestrae, fused vesicle walls Diffusion through endothelial cells - channels lipid-soluble su